DOI:
10.1039/C7RA01709J
(Paper)
RSC Adv., 2017,
7, 17497-17504
1-Alkyl-3-methylimidazolium 4-organyloxy-2,3,5,6-tetrafluorophenyltrifluoroborates as a new platform for ionic liquids with specific properties†
Received
10th February 2017
, Accepted 12th March 2017
First published on 20th March 2017
Abstract
A new synthetic platform for ionic liquids (ILs) with specific properties was suggested based on the polyfluorophenyltrifluoroborate anions, Q[4-XC6F4BF3] (X = F, RO). Convenient preparative approaches to these ILs including the ones with chiral counteranions were developed. The dependence of physicochemical properties of ILs on the nature of substituents in the fluorinated ring was studied.
Introduction
It is known that the properties of molten salt systems are mainly determined by the Coulomb interactions between the cations and anions1 which are responsible for the formation of ion pairs and higher ionic clusters that shorten the interionic distance and decrease the coordination number of ions. As a result, the volume increases during melting and forming “free” volume.2 According to the current definitions, ionic liquids (ILs) are salts in the liquid state at temperatures below 100 °C; they are a special kind of molten salt system.3 Unique physical and chemical properties of ILs are tunable upon changing the anion and cation and have determined application areas of ionic liquids, including electrochemistry,4–7 organic synthesis,8–16 liquid/liquid extraction,17–19 catalysis,20–26 and nanostructured materials.27–34 There are several features which make ILs different from the conventional molten salts.35 First, at least one of the ions of the ionic liquid must possess a molecular asymmetry. This prevents the strong charge ordering which promotes the system to crystallize in the case of conventional molten salts.36 Second, the delocalization of the positive and negative charges affects directly the energy of the Coulomb interactions of the cations and anions. Addition to the Coulomb interactions is a network of hydrogen bonds between the cations and anions inducing their structural orientation.2
The anions with delocalized negative charge in combination with 1,3-dialkylimidazolium cations produce low-viscous and poorly water-miscible ionic liquids.18,37–44 1,3-Dialkylimidazolium salts (RR′Im+X−) are the most stable and conductive among the known ionic liquids.7,40,44–49 One of these salts are corresponding tetrafluoroborates, Q[BF4].50 Many researchers studied their properties. The main drawback of ILs based on tetrafluoroborate anion is their limited hydrolytic stability.3 The hydrolytic stability of ILs based on fluoroborates salts can be improved by substituting of perfluoroalkyl39,51–54 or cyano37 group for one or more fluorine atoms in the BF4 anion. The first attempt to produce ionic liquids with an arylboron-containing anion, phenyltrifluoroborate, is reported elsewhere.55 This approach was used for preparing a series of ionic liquids with interesting properties.36,37,56–60
There are only scarce literature data on ionic liquids with aromatic anions;35 the salts of arylcarboxylic and arylsulfonic acids are only known.61–63 However, ILs with aryl-containing counteranion are expected to feature many interesting properties due to more efficient delocalization of the negative charge and a number of specific interactions of the aromatic ring.5,60–66
Despite the progress in preparing functional ILs, it is still necessary to develop synthetic routes to new types of these materials is of actual importance. Moreover, the universal methods for modification of the IL structure and preparation of a wide range of new materials by this way are of great interest. Polyfluorinated aryltrifluoroborates are attractive as starting compounds for the synthesis of functional ILs.67–69 The cumulative effect of several fluorine atoms in the aromatic ring provides a high chemical stability along with unique electron withdrawing properties characteristic of these compounds.68 In the present work we offer a new chemical platform for ionic liquids with specific properties based on polyfluorophenyltrifluoroborate salts.68,69
Results and discussion
Synthesis of ionic liquids
New ionic liquids were obtained by ion exchange (metathesis) reactions of Q+X− (X = Cl, Br; Q = Et4N (1a), 1-ethyl-3-methylimidazolium (EMIM) (1b), 1-butyl-3-methylimidazolium (BMIM) (1c), 1-methyl-3-octylimidazolium (OMIM) (1d) and 1-butyl-2,3-dimethylimidazolium (BMMIM) (1e)) with potassium pentafluorophenyltrifluoroborate (2) (Scheme 1). The reactions can be carried out in the solvents where the starting compounds but not the produced potassium halide are readily soluble (for example, acetonitrile or acetone). In acetone, the preferable initial materials are ionic liquids 1 containing chloride ions due to the fact that the alkali metal chlorides, the ion exchange by-product, are ca. 200–300 times less soluble in acetone than the corresponding bromides. In the acetone media the reaction proceeds at room temperature and allows halogen-free ionic liquids 3 to be obtained in the presence of a minor excess of salt 2 to be removed by dissolving the reaction mixture in dichloromethane.
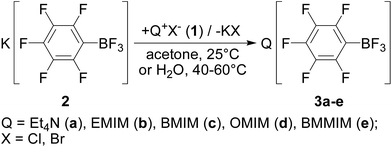 |
| Scheme 1 Preparation of the ionic liquids based on the C6F5BF3− anion. | |
Attempts to prepare 3a–e in water led shortly to the formation of a new phase of the hydrophobic ionic liquid. The optimal temperature for this metathesis was found to range from 40 to 60 °C. Higher temperatures favored the phase separation due to a decreased viscosity of ILs, but in parallel way, the solubility of salt 2 in water also increased.
For preparation of 1-butyl-3-methylimidazolium 4-RO-2,3,5,6-tetrafluorophenyltrifluoroborates 5a–h we used the reaction of BMIMCl with the corresponding salts 4a–h in acetone (Scheme 2). The salts were obtained by the earlier described reaction of nucleophilic substitution of p-fluorine atom in pentafluorotrifluoroborate anion with appropriate nucleophiles.70 The same technique was used for synthesis of chiral salts 4g and 4h from L(−)-isopulegol and L(−)-borneol respectively.
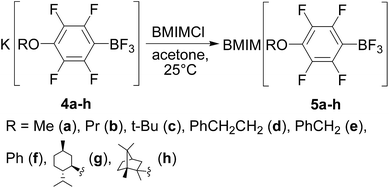 |
| Scheme 2 Preparation of the ionic liquids based on the [4-ROC6F4BF3]-anions. | |
Alternatively, ionic liquids 5 may be prepared directly from BMIM[C6F5BF3] by nucleophilic substitution with a suitable reagent (Scheme 3).
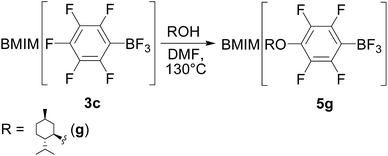 |
| Scheme 3 An alternative approach to preparation of ionic liquids 5 (with preparation 5g as an example). | |
The presented results demonstrate a high synthetic potential of pentafluorophenylborate anion for preparation of new ILs. A variety of ILs can be obtained either by cation metathesis of QX and K[ArBF3] or by modification of Q[C6F5BF3] by an appropriate O-nucleophile. This opens wide opportunities for easy tuning of desired physico-chemical properties of ILs using the optimal synthetic routes.
Thermal properties
The presented data (Table 1) indicate that the cationic moiety influences significantly the primary characteristics of the resulting material. For example, in contrast to K[C6F5BF3] decomposed at 328–329 °C,71 salts 3 are the less thermally stable. ILs 3b–3d are less stable, they are decomposed at ca. 200 °C, whereas the far lower decomposition temperature of Et4N[C6F5BF3] 3a is accounted for by the Hofmann-type decomposition of the tetraethylammonium cation. Introduction of a substituent at the para-position of the fluorinated ring does not affect significantly the thermal stability of the compounds: 5a–h compounds also are decomposed at 200–220 °C.
Table 1 Thermal properties of ionic liquids Q[ArBF3]
Ionic liquid |
Melting point, °C |
Temperature of decomposition,a °C |
Temperature of starting decomposition. Data from ref. 40. Glass transition temperature. |
BMIM[BF4] |
−83b |
425b |
Et4N[C6F5BF3] (3a) |
+55 |
145 |
EMIM[C6F5BF3] (3b) |
+40 |
199 |
BMIM[C6F5BF3] (3c) |
+35 |
203 |
OMIM[C6F5BF3] (3d) |
+10 |
200 |
BMMIM[C6F5BF3] (3e) |
+35 |
219 |
BMIM[4-MeOC6F4BF3] (5a) |
+9 |
217 |
BMIM[4-PrOC6F4BF3] (5b) |
−3 |
223 |
BMIM[4-t-BuOC6F4BF3] (5c) |
+33 |
227 |
BMIM[4-Ph(CH2)2OC6F4BF3] (5d) |
∼−50c |
218 |
BMIM[4-PhCH2OC6F4BF3] (5e) |
+22 |
210 |
BMIM[4-PhOC6F4BF3] (5f) |
+78 |
219 |
BMIM[4-isopulegylOC6F4BF3] (5g) |
∼−50c |
208 |
BMIM[4-bornylOC6F4BF3] (5h) |
+70 |
205 |
The cation nature affects the salt melting temperatures in a more complicated manner. IL 3a is solid under normal conditions. Salts 3b and 3c can be supercooled melts at room temperature. These substances tend to bulk crystallization on cooling but remain at room temperature solid upon heating. Note that a similar effect, i.e. the difference between the melting and freezing points, was described earlier for other ionic liquids based on the dialkylimidazolium cation.46,47,72 The IL melting point reduces as the hydrocarbon chain of the imidazolium cation lengthens. We can suppose, by analogy with Q+Hal−, that the further lengthening of the alkyl constituent (12 carbon atoms and more) of the cation again causes a higher melting point due to a stronger influence of the long hydrocarbon chain on the IL physical properties.
The introduction of a RO group at the para-position of the fluorinated aromatic ring has an evident effect. While 5f and 5h are solids (melting points 70–78 °C, see Table 1), the other compounds 5 are liquids at room temperature.
Melting points of ionic liquids with substituents of identical nature depend on their structure. In a series of ionic liquids 5d–f with phenyl and phenylalkyl moieties of the fluorinated anion, the melting temperature decreases with increasing of the substituents in size. This is not the same with ionic liquids containing aliphatic structural fragments of the anion. Ionic liquids 5c and 5h contain bulky fragments of the anions but melt at a relatively high temperature, while 5a with a small MeO substituent at a slightly lower temperature. We failed to observe crystallization of ionic liquid 5g containing an isopulegol fragment.
In common, the reduced meting point of IL is though to result from asymmetry of at least one of the ions. However, there is no clarity in the case. Melting points of EMIM[CnH2n+1BF3] (n = 1–5) vary from −14 (n = 3) to −9 (n = 4) and 16 °C (n = 5) while EMIM[BF4] melts at 15 °C.73 This is also the case with their analogues, EMIM[CnF2n+1BF3], that melt at −1, 8 and −20 °C at n = 2, 3 and 4, respectively.54 In our series, melting points are much higher than those of BMIM[BF4] (Table 1). It may happen that the presence of boron atom gives rise to additional interactions and, therefore, to more compact relative position of anions and cations. An indirect evidence of this assumption may be the density of salt 3c (1.68 g mL−1) which close to that of BMIM[BF4] (1.21 g mL−1). The further reduction of the anion symmetry due to introduction of a substituent at the para-position to boron of the polyfluoroaromatic ring leads in most cases to a decrease in the melting temperature of ILs. If so, behavior of the salts seems abnormal. In the case of salts 5d–f the melting temperature increases, apparently, due to the π-stacking interaction between the phenyl group and the substituted tetrafluorophenyl group. Such an interaction is weakened substantially in the salts by respective bridging groups that prevent the local microstructuring in ILs.
Spectral properties
Chemical compositions of ILs were studied by 1H, 11B, 13C and 19F NMR spectroscopy as well as by elemental analysis. While chemical shifts in the spectra of imidazolium salts depend strongly on the solvent and concentration,74–78 the NMR spectra of 3a–e and 5a–h were acquired with the solutions in CDCl3 at the concentration of ca. 30 g L−1. The obtained NMR data are presented in Table 2.
Table 2 Selected NMR data for ILs Q[RBF3]
Ionic liquid Q[RBF3] |
Chemical shift, ppm |
1Ha |
13Cb |
11B |
19Fc |
H-2 of imidazolium cation. C-2 of imidazolium cation. 19F NMR signal of group BF3−. |
BMIM[BF4] |
8.83 |
136.91 |
−0.99 |
−152.67 |
BMMIM[BF4] |
— |
143.95 |
−1.24 |
−153.83 |
Et4N[C6F5BF3] (3a) |
— |
— |
1.86 |
−135.93 |
EMIM[C6F5BF3] (3b) |
9.00 |
136.64 |
2.05 |
−135.00 |
BMIM[C6F5BF3] (3c) |
8.89 |
136.60 |
2.03 |
−134.83 |
OMIM[C6F5BF3] (3d) |
8.90 |
136.57 |
2.03 |
−134.79 |
BMMIM[C6F5BF3] (3e) |
— |
— |
1.76 |
−136.14 |
BMIM[4-MeOC6F4BF3] (5a) |
8.99 |
136.71 |
2.14 |
−134.60 |
BMIM[4-PrOC6F4BF3] (5b) |
8.79 |
136.37 |
2.17 |
−134.60 |
BMIM[4-t-BuOC6F4BF3] (5c) |
8.68 |
136.19 |
2.19 |
−134.40 |
BMIM[4-Ph(CH2)2OC6F4BF3] (5d) |
8.90 |
136.69 |
2.20 |
−134.63 |
BMIM[4-PhCH2OC6F4BF3] (5e) |
9.00 |
136.24 |
2.18 |
−134.80 |
BMIM[4-PhOC6F4BF3] (5f) |
8.77 |
136.25 |
2.16 |
−134.50 |
BMIM[4-isopulegylOC6F4BF3] (5g) |
8.93 |
136.65 |
2.19 |
−134.53 |
BMIM[4-bornylOC6F4BF3] (5h) |
8.93 |
136.79 |
2.15 |
−134.54 |
In the 1H NMR spectrum, the chemical shift of the ring hydrogen H-2 in 1,3-dialkylimidazolium cation correlates with the cation–anion hydrogen bonding strength and with the inductive effect of proximity of anion to cation.74–79 For example, in the series of BMIM X the chemical shift δ(H-2) moves from 10.22 (X = Cl), to 9.78 (X = Br), 9.28 (X = I) and 8.50 (X = BF4) ppm.79 The chemical shift of H-2 in the spectra of EMIM[C6F5BF3], BMIM[C6F5BF3], and OMIM[C6F5BF3] show the less, but remarkable change of location that correlates with increase of length of alkyl chain. However, the effect of anion [C6F5BF3] is weaker than effect of [BF4]. In the 13C NMR spectrum, signals of C-2 behave similar way: the carbon atom is more deshielded in BMIM[BF4] than in BMIM[C6F5BF3]. Elongation of the carbon chain at C-3 leads to shielding of carbon C-2, although the range of difference is only 0.07 ppm (cf. Δδ(C) = δ(C-2) (OMIM[BF4]) − δ(C-2) (OMIM[NTf2]) = 0.8 ppm74–78).
The contribution of hydrogen bond (C-2)–H⋯F–B in NMR spectra of 3b–d is seen clearly when comparing 11B and 19F NMR spectra of Et4N[C6F5BF3] and BMMIM[C6F5BF3] where this intermolecular interaction is absent and chemical shifts of both boron and fluorine atoms of BF3 moiety are determined mainly by the Coulomb forces. Indeed, signals of fluorine atoms bonded to boron in anion [C6F5BF3] are located at −136 ppm while the corresponding atoms in spectra of 3b–3d are remarkably deshielded. The boron atoms in counteranions of 3b–d are deshielded too with respect to positions of the 11B resonances in spectra of 3a and 3e which located at 1.76 and 1.86 ppm (Table 2).
In common, replacement of fluorine atom at the position para to boron by RO substituent increases the cation–anion aggregation. The 1H and 13C NMR spectra of BMIM cation indicate stronger hydrogen bonding with anion in 5a, 5b, 5d–h than in BMIM[C6F5BF3]. This also confirms by the deshielding of 11B and 19F resonances of BF3 moiety of [4-ROC6F4BF3] relative those in [C6F5BF3]. The peculiar case is BMIM[4-t-BuOC6F4BF3] 5c. Both hydrogen and carbon atoms of the (C-2)–H moiety are more shielded than those in BMIM[BF4] and BMIM[C6F5BF3]. These parameters meet the criteria of the weak cation–anion interaction, e.g. their state as solvent-separated ion pair. On the other hand, the 11B and 19F NMR spectra show deshielding of boron and fluorines atoms similar to that in salts 5 (contact ion pair). We prefer to consider the spectral performance data on counteranion as a more reliable indicator of salt aggregation in the solution and believe that the observed features reflect the different organisation of contact ion pair 5c in CDCl3 with respect to contact ion pair 5a, 5b, 5d–h because of bulky tert-butyl moiety at para-position to boron.
Miscibility of ILs with water and organic solvents
Data on the solubility of prepared ILs in water and organic solvents are presented in Table 3. They all except BMIM[BF4] are seen to be insoluble or poorly soluble in water. Nevertheless, even though ionic liquids 5a and 5b exhibit hydrophobic properties, their solubility in water is rather different from the solubility of the other ionic liquids.
Table 3 Solubility of ILs in water and organic solvents
Ionic liquid |
Solubility of ILs in solventsa |
H2O |
Hydro-carbons |
Benzene |
EtOAc |
Ether |
Dioxane |
Polar solventsb |
CCc |
(+) soluble (>10 mg mL−1), (−) insoluble (<1 mg mL−1), (±) poorly soluble. Methanol, ethanol, acetone and acetonitrile. Chlorocarbons: dichloromethane, trichloromethane, 1,2-dichloroethane. BMIM[BF4] is insoluble in isopropanol. |
BMIM[BF4] |
+ |
− |
− |
− |
− |
+ |
+d |
+ |
Et4N[C6F5BF3] (3a) |
− |
− |
+ |
+ |
− |
+ |
+ |
+ |
EMIM[C6F5BF3] (3b) |
− |
− |
+ |
+ |
− |
+ |
+ |
+ |
BMIM[C6F5BF3] (3c) |
− |
− |
+ |
+ |
− |
+ |
+ |
+ |
OMIM[C6F5BF3] (3d) |
− |
− |
+ |
+ |
+ |
+ |
+ |
+ |
BMMIM[C6F5BF3] (3e) |
− |
− |
+ |
+ |
− |
+ |
+ |
+ |
BMIM[4-MeOC6F4BF3] (5a) |
± |
− |
+ |
+ |
+ |
+ |
+ |
+ |
BMIM[4-PrOC6F4BF3] (5b) |
± |
− |
+ |
+ |
+ |
+ |
+ |
+ |
BMIM[4-t-BuOC6F4BF3] (5c) |
− |
− |
− |
+ |
+ |
+ |
+ |
+ |
BMIM[4-Ph(CH2)2OC6F4BF3] (5d) |
− |
− |
+ |
+ |
− |
+ |
+ |
+ |
BMIM[4-PhCH2OC6F4BF3] (5e) |
− |
− |
+ |
+ |
− |
+ |
+ |
+ |
BMIM[4-PhOC6F4BF3] (5f) |
− |
− |
+ |
+ |
− |
+ |
+ |
+ |
BMIM[4-isopulegylOC6F4BF3] (5g) |
− |
− |
+ |
+ |
+ |
+ |
+ |
+ |
BMIM[4-bornylOC6F4BF3] (5h) |
− |
− |
+ |
+ |
− |
+ |
+ |
+ |
The solubility of ionic liquids in water is one of the most studied subjects; it differs significantly from the dissolution process of conventional salts. The interaction of cations and anions with water molecules should be considered separately when interpreting experimental data on the water solubility of ILs. Since the imidazolium and related cations possess amphiphilic properties,80 the corresponding ILs can behave as surfactants and self-assemble into various aggregates in aqueous media.81 In general, the process of water dissolution is mainly determined by solvation of anions. The energy of such interaction in the case of anions can be as high as two times of the similar energy for cations.82 This fact explains adequately the lack of observable effects during the interaction of salts 3b–d with water.
Substitution of the hydrophobic pentafluorophenyl group for a fluorine atom in BF4− leads to a decrease in the anion solvation energy. As a result, salts 3a–e and 5c–h are water insoluble. A better solubility of ionic liquids 5a and 5b can be caused by the participation of oxygen atoms of the alkoxide moiety in the formation of hydrogen bonds with water molecules. In fact, ILs 5a and 5b can be both hydrogen bond acceptor (anion) and donor (cation) and are expected to interact with solvents bearing both accepting and donating sites.83 Ionic liquids 5c–h with oxygen containing anions are poorly soluble in water, probably, due to segregation of the oxygen atoms that prevents the formation of hydrogen bonds.
In contrast to Q[BF4], ILs 3a–e and 5a–h are freely soluble in benzene. Introduction of polyfluorinated aromatic moiety into the anion is expected to improve the solubility of ILs in aromatics (due to additional intramolecular interactions such as π-stacking) but not in saturated hydrocarbons. In 5c, the tert-butyl group prevents interaction of the π-system in anion with the aromatic component of the solvent and, therefore, makes the salt poorly soluble.
Similar to aqueous ILs solutions, the mixtures of the prepared ILs with benzene are more complex than conventional mixtures of ILs with neutral solvents. The microstructure of such mixtures is largely determined by the interaction of cations with the solvent molecules,84–86 but systematic studies of the dependence of the microscopic structure of “ionic liquid – aromatic solvent” systems on the anion nature are as yet unknown. One of the first works in the area has been published recently.78
There is a more ambiguous case when an aromatic compound and ionic liquids are the solute and solvent, respectively. From literature,87,88 the benzene solubility is 1.2–2 mol per mol of BMIM[BF4]. The substitution of [C6F5BF3] for [BF4] increases the benzene solubility up to seven mol per mol BMIM[C6F5BF3] that agrees with the assumed role of π-stacking between the aromatic solute and IL solvents.
The influence of the cation nature is illustrated with IL 3b–d as an example. Elongation of the alkyl chain in IL leads to stronger hydrophobic properties and to a good solubility of IL 3d in diethyl ether. Other ILs with the pentafluorophenyltrifluoroborate anions are insoluble in this solvent. Introduction of an alkoxide moiety into the polyfluoroaromatic ring of the anion makes the corresponding ionic liquids soluble in Et2O of. However, this is not the case of salts 5d–f.
ILs 3a–e and 5a–h are insoluble in hydrocarbons but soluble in polar organic solvents (acetone, acetonitrile, alcohols, chloroalkanes). In contrast to the BMIM[BF4], ILs 3a–e and 5a–h are soluble in ethyl acetate.
Thus, the above data demonstrate clearly that the substitution of a pentafluorophenyl group for fluorine in BF4 anion can change significantly the solubility of the corresponding ionic liquids in water and organic solvents. The introduction of an alkoxy or aryloxy substituent in the perfluorinated aromatic ring allows some properties of the resulting ILs to be changed. This feature opens up opportunities for applying ILs in various fields.
Other properties of ILs
The optical rotation of chiral ionic liquids was measured. The α20D for chiral ILs 5g and 5h (acetone, 1.00 g/100 mL) are −10.7 and −15.2 respectively. This is less as 2.4 times of the optical rotation of isopulegol (α20D = −25.9) and borneol (α20D = −37.9). Since, according to NMR, we do not observed significantly structural and electronic changes in chiral substituent in the IL structure, the decrease in α20D can be accounted for by a decrease in the relative content of chiral centers per unit mass. The viscosity of IL's 3 was measured. We observed that prepared ionic liquids are more viscous than BMIM[BF4] and viscosity increases with the cation size (the data of viscosity measurements are available in the ESI†).
Experimental
The NMR spectra were recorded on a Bruker AVANCE III 500 (1H at 500.13 MHz, 11B at 160.46 MHz, 13C at 125.76 MHz, and 19F at 470.59 MHz) spectrometer. The chemical shifts were referenced to TMS (1H, 13C), BF3O(C2H5)2 in CDCl3 (15% v/v) (11B), and CCl3F (19F, with C6F6 as secondary reference (−162.9 ppm)).
TG-DSC analyses were performed using a Netzsch STA409 instrument with a heating rate of 10 K min−1 under He flow. The melting points (for ILs 3) were determined from DSC curves as Tonset. Temperature of decomposition (Td) was determined from the TG diagrams as Tonset.
Optical rotations were measured on a polAAr 3005 polarimeter with a 100 mm cell.
The melting points of the ionic liquids 5 were estimated visually by keeping of IL sample upon continuously increasing temperature (with the rate ∼1 °C min−1) using the LOIP FT 311-80 cryostat (−80 to +100 °C).
Elemental analysis was performed in the Collective Service Center of SB RAS (Novosibirsk).
DMF was purified by distillation over P4O10 under reduced pressure. NaH (60% dispersion in mineral oil) (Sigma-Aldrich), L(−)-borneol (Acros) and other commercially available was used as supplied. Potassium pentafluorophenyltrifluoroborate (2) was prepared according to literature method.89 Potassium 4-RO-tetrafluorophenyltrifluoroborates (4a–f) were prepared according to described methods.70
Potassium (1R,2S,5R)-5-methyl-2-(prop-1-en-2-yl)cyclohexyloxy-2,3,5,6-tetrafluorophenyltrifluoroborate (4g)
A 100 mL round-bottomed flask fitted with magnetic stirrer bar and an argon supply equipment was charged with 5.43 g (35 mmol) of L(−)-isopulegol. 1.28 g (32 mmol) of 60% dispersion NaH was added in portions and then 40 mL DMF was added. The resulted mixture was stirred at 25 °C for 1 h under an atmosphere of argon, and then K[C6F5BF3] (6.03 g, 22 mmol) was added in one portion. The flask was put into heating bath at 130 °C and the reaction mixture was stirred for 5 h at this temperature. Then it was cooled and 5 mL of water was added. The resulting mixture was transferred into 250 mL PP cup. 17.18 g (220 mmol) of KHF2 was added and mixture was stirred for 8 h at room temperature. To a resulting suspension 1.1 g (8 mmol) K2CO3 and 40 mL of acetonitrile were added and suspension was stirred for 1 h. The suspension was filtered through the silica gel (60–200 μm, ∼40 mL) and cake was washed by 2 × 20 mL of acetonitrile, filtrate was evaporated in vacuo. The product was purified by recrystallization from 120 mL of ethanol and dried in vacuum-desiccator. 5.60 g (63%) of 4g as white powder was prepared.
1H NMR (acetone-d6): δ 4.80–4.85 (m, 1H, Ha–CH
); 4.72–4.77 (m, 1H, Hb–CH
); 4.21 (td, 1H, 3JHH 10.5 Hz, 3JHH 4.4 Hz, H-1); 2.22–2.30 (m, 1H, H-5); 1.96 (dt, 1H, 3JHH 12.2 Hz, 3JHH 3.9 Hz, H-2); 1.77 (dd, 3H, 4JHH 1.5 Hz, 4JHH 0.8 Hz, CH3–C
); 1.64–1.71 (m, 2H, CH2-6); 1.42–1.53 (m, 2H, H-3,4); 0.96–1.18 (m, 2H, H-3,4); 0.91 (d, 3H, 3JHH 6.7 Hz, CH3-5).
19F NMR (acetone-d6): δ −133.46 (q, 3F, 2JBF 42.8 Hz, BF3), −135.53 (ddq, 2F, 3JFF 23.0 Hz, 5JFF 11.8 Hz, 4JFF(BF3) 11.7 Hz, F-2,6); −157.99 (dd, 2F, 3JFF 23.9 Hz, 5JFF 11.0 Hz, F-3,5).
Potassium 4-bornyloxy-2,3,5,6-tetrafluorophenyltrifluoroborate (4h)
A 250 mL round-bottomed flask fitted with magnetic stirrer bar and an argon supply equipment was charged with 10.86 g (70 mmol) L(−)-borneol. 85 mL of DMF and 2.46 g (64 mmol) of 60% dispersion NaH were added. The resulted mixture was stirred at 25 °C for 1 h under an atmosphere of argon, and K[C6F5BF3] (12.06 g, 44 mmol) was added in one portion. The flask was put into heating bath at 130 °C and the reaction mixture was stirred for 5 h. The reaction mixture was cooled and 10 mL of water was added. The resulting mixture was transferred into 500 mL PP cup. 34.37 g (440 mmol) of KHF2 was added and mixture was stirred for 8 h at room temperature. K2CO3 (2.2 g, 16 mmol) and 80 mL of acetonitrile were added and then suspension was stirred for 1 h. The suspension was filtered trough the silica gel (60–200 μm, ∼80 mL) and cake was washed by 2 × 40 mL of acetonitrile, the collected filtrate was evaporated in vacuo. The product was purified by recrystallization from 150 mL of ethanol and dried in vacuum-desiccator. 10.00 g (55%) of product 4h as white powder was prepared.
1H NMR (acetone-d6): δ 4.45 (d, 1H; 3JHH 10 Hz, H-2); 2.19–2.31 (m, 2H, H-3,6); 1.76–1.80 (m, 1H, H-5); 1.69 (dd, 1H, 3JHH 4.5 Hz; 3JHH 4.5 Hz, H-4); 1.28–1.39 (m, 3H, H-3,5,6); 0.95 (s, 3H, CH3–C7); 0.90 (s, 3H, CH3–C7); 0.88 (s, 3H, CH3–C1).
19F NMR (acetone-d6): δ −133.26 (q, 3F, 2JBF 43.5 Hz, BF3), −136.57 (ddq, 2F, 3JFF 23.2 Hz; 5JFF 11.6 Hz; 4JFF(BF3) 11.5 Hz, F-2,6); −158.41 (dd, 2F, 3JFF 23.9 Hz, 5JFF 10.9 Hz, F-3,5).
General procedure for preparation of Q[ArBF3]
Preparation of ILs in water. A 100 mL beaker was charged with 5.5 mmol of corresponding tetraalkylammonium or 1,3-dialkylimidazolium halide, 5 mmol of K[C6F5BF3] and 40 mL of water. The mixture was stirred at 50°C for 2 h. The water phase was removed by decantation and IL phase was washed by 2 × 20 mL of warm (50 °C) water. The products were dried in high vacuo.
Preparation of ILs in acetone. A 50 mL round-bottomed flask fitted with magnetic stirrer bar was charged with 5 mmol corresponding tetraalkylammonium or 1,3-dialkyl- or 1,2,3-trialkylimidazolium halide, 5.1 mmol of corresponding borate (1–9) and 20 mL of acetone. The mixture was stirred for 2 h at 25 °C. The solid was filtered and washed by 5 mL of acetone. The second portion of corresponding salt 1–9 (1 mmol) was added and the resulting mixture was stirred for 2 h at 25 °C. The solid was filtered and washed by 5 mL of acetone. The filtrate was evaporated in vacuo. The residue was solved in 5 mL of dichloromethane (to remove potassium halogenide and excess of potassium polyfluorophenyltrifluoroborate) and filtered. The filtrate was evaporated and product was dried in high vacuo.
An alternative approach for preparation ILs 5 (preparation of 5g). A 25 mL round-bottomed flask fitted with magnetic stirrer bar and an argon supply equipment was charged with 4.06 g (26 mmol) of L(−)-isopulegol. 0.86 g (21 mmol) of 60% dispersion NaH was added in portions and then 15 mL DMF was added. The resulted mixture was stirred at 25 °C for 1 h under an atmosphere of argon, and then solution of BMIM[C6F5BF3] (3c, 2.0 g, 5 mmol) in 5 mL of DMF was added. The flask was put into heating bath at 130 °C and the reaction mixture was stirred for 5 h at this temperature. Then it was cooled and 0.5 mL of water was added. The mixture was evaporated in vacuo and cake was suspended in 10 mL of CH2Cl2. The product was isolated by column chromatography (silica 60–200 μm, d 10 mm, h 300 mm, eluent CH2Cl2). The solution was evaporated in vacuo. The product was dried in high vacuo. 1.84 g (68%) of 5g as light brown liquid was prepared.
Conclusions
This paper presents a new platform for ionic liquids based on pentafluorophenyltrifluoroborate or para-substituted tetrafluorophenyltrifluoroborate anions (Fig. 1). New ionic liquids can be prepared at high yields by ion exchange under mild conditions or directly by nucleophilic substitution of BMIM[C6F6BF3] with appropriate reagents.
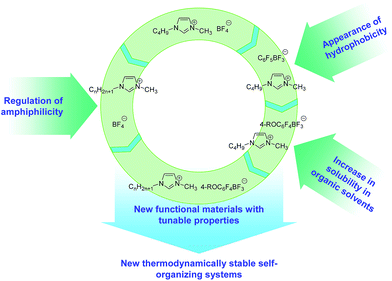 |
| Fig. 1 A place of new ionic liquids, and a generalized representation of their properties. | |
Fundamental properties of the ionic liquids are determined by the Coulomb interaction and through the formation of hydrogen bonds between hydrogen atom H-2 of the imidazolium cation and fluorine atoms of BF3 moiety. The insertion of a polyfluorinated aromatic ring into the anion changes their behavior in water and organic solvents. This circumstance opens wide possibilities for use ionic liquids obtained in this study in various fields as functional materials.
Acknowledgements
The works on preparation of novel ionic liquids was conducted within the framework of budget project No. 0303-2016-0007 for Boreskov Institute of Catalysis. The study of the ILs properties have been made with the financial support of the Russian Science Foundation (No. 14-13-00826). The works on preparation of new salts of organotrifluoroborates were supported by the Russian Foundation for Basic Research (grant 16-29-10762).
References
- W. Freyland, Coulombic Fluids: Bulk and Interfaces, Springer-Verlag, Berlin Heidelberg, 2011 Search PubMed.
- J. Dupont, Acc. Chem. Res., 2011, 44, 1223–1231 CrossRef CAS PubMed.
- T. Welton, Chem. Rev., 1999, 99, 2071–2084 CrossRef CAS PubMed.
- A. S. Shaplov, E. I. Lozinskaya, P. S. Vlasov, S. M. Morozova, D. Y. Antonov, P. H. Aubert, M. Armand and Y. S. Vygodskii, Electrochim. Acta, 2015, 175, 254–260 CrossRef CAS.
- I. A. Shkrob and T. W. Marin, J. Phys. Chem. B, 2015, 119, 14766–14779 CrossRef CAS PubMed.
- Y. L. Zhu, Y. Katayama and T. Miura, Electrochem. Solid-State Lett., 2011, 14, D110–D115 CrossRef CAS.
- A. B. McEwen, H. L. Ngo, K. LeCompte and J. L. Goldman, J. Electrochem. Soc., 1999, 146, 1687–1695 CrossRef CAS.
- M. Gazitua, R. A. Tapia, R. Contreras and P. R. Campodonico, New J. Chem., 2014, 38, 2611–2618 RSC.
- A. D. Sawant, D. G. Raut, N. B. Darvatkar and M. M. Salunkhe, Green Chem. Lett. Rev., 2011, 4, 41–54 CrossRef CAS.
- J. P. Hallett and T. Welton, Chem. Rev., 2011, 111, 3508–3576 CrossRef CAS PubMed.
- H. Zhao, Z. Y. Song, O. Olubajo and J. V. Cowins, Appl. Biochem. Biotechnol., 2010, 162, 13–23 CrossRef CAS PubMed.
- S. Fujita, M. Nishiura and M. Arai, Catal. Lett., 2010, 135, 263–268 CrossRef CAS.
- S. G. Zlotin and N. N. Makhova, Mendeleev Commun., 2010, 20, 63–71 CrossRef CAS.
- P. Wasserscheid and T. Welton, Ionic liquids in synthesis, Wiley Online Library, 2008 Search PubMed.
- A. Kamimura, T. Okagawa, N. Oyama, T. Otsuka and M. Yoshimoto, Green Chem., 2012, 14, 2816–2820 RSC.
- I. Newington, J. M. Perez-Arlandis and T. Welton, Org. Lett., 2007, 9, 5247–5250 CrossRef CAS PubMed.
- S. Oppermann, F. Stein and U. Kragl, Appl. Microbiol. Biotechnol., 2011, 89, 493–499 CrossRef CAS PubMed.
- R. K. Goyal, N. S. Jayakumar and M. A. Hashim, J. Hazard. Mater., 2011, 195, 55–61 CrossRef CAS PubMed.
- Y.-X. Wang and X.-J. Cao, Process Biochem., 2012, 47, 896–899 CrossRef CAS.
- E. Brenna, M. Crotti, F. G. Gatti, A. Manfredi, D. Monti, F. Parmeggiani, S. Santangelo and D. Zampieri, ChemCatChem, 2014, 6, 2425–2431 CrossRef CAS.
- Q. Zhang, S. Zhang and Y. Deng, Green Chem., 2011, 13, 2619–2637 RSC.
- D. Betz, P. Altmann, M. Cokoja, W. A. Herrmann and F. E. Kuhn, Coord. Chem. Rev., 2011, 255, 1518–1540 CrossRef CAS.
- H. Olivier-Bourbigou, L. Magna and D. Morvan, Appl. Catal., A, 2010, 373, 1–56 CrossRef CAS.
- M. Sureshkumar and C.-K. Lee, J. Mol. Catal. B: Enzym., 2009, 60, 1–12 CrossRef CAS.
- X. F. Liu, L. F. Xiao, H. J. Wu, Z. Li, J. Chen and C. U. Xia, Catal. Commun., 2009, 10, 424–427 CrossRef CAS.
- D. S. Gaikwad, Y. Park and D. M. Pore, Tetrahedron Lett., 2012, 53, 3077–3081 CrossRef CAS.
- Y. Liu, L. J. Qiao, Y. P. Xiang and R. Guo, Langmuir, 2016, 32, 2582–2590 CrossRef CAS PubMed.
- S. Polarz, S. Landsmann and A. Klaiber, Angew. Chem., Int. Ed., 2014, 53, 946–954 CrossRef CAS PubMed.
- X. Mulet, C. E. Conn, C. Fong, D. F. Kennedy, M. J. Moghaddam and C. J. Drummond, Acc. Chem. Res., 2013, 46, 1497–1505 CrossRef CAS PubMed.
- T. L. Greaves and C. J. Drummond, Chem. Soc. Rev., 2013, 42, 1096–1120 RSC.
- M. E. Mahmoud, Desalination, 2011, 266, 119–127 CrossRef CAS.
- M. J. Zhou, T. J. Kidd, R. D. Noble and D. L. Gin, Adv. Mater., 2005, 17, 1850–1853 CrossRef CAS.
- C. S. Consorti, P. A. Z. Suarez, R. F. de Souza, R. A. Burrow, D. H. Farrar, A. J. Lough, W. Loh, L. H. M. da Silva and J. Dupont, J. Phys. Chem. B, 2005, 109, 4341–4349 CrossRef CAS PubMed.
- L. P. Zhang, E. Z. Chen and X. J. Yu, J. Radioanal. Nucl. Chem., 2013, 298, 1055–1063 CrossRef CAS.
- K. Goossens, K. Lava, C. W. Bielawski and K. Binnemans, Chem. Rev., 2016, 116, 4643–4807 CrossRef CAS PubMed.
- S. Tsuzuki, T. Umecky and H. Matsumoto, in Molten Salts and Ionic Liquids 17, Electrochemical Soc Inc, Pennington, 2010, vol. 33, pp. 685–689 Search PubMed.
- N. V. Ignat'ev, M. Finze, J. A. P. Sprenger, C. Kerpen, E. Bernhardt and H. Willner, J. Fluorine Chem., 2015, 177, 46–54 CrossRef.
- T. Singh and A. Kumar, J. Phys. Chem. B, 2007, 111, 7843–7851 CrossRef CAS PubMed.
- Z. B. Zhou, H. Matsumoto and K. Tatsumi, Chem.–Eur. J., 2004, 10, 6581–6591 CrossRef CAS PubMed.
- H. Tokuda, K. Hayamizu, K. Ishii, M. Abu Bin Hasan Susan and M. Watanabe, J. Phys. Chem. B, 2004, 108, 16593–16600 CrossRef CAS.
- P. A. Z. Suarez, S. Einloft, J. E. L. Dullius, R. F. de Souza and J. Dupont, J. Chim. Phys. Phys.-Chim. Biol., 1998, 95, 1626–1639 CrossRef CAS.
- J. Fuller, R. T. Carlin and R. A. Osteryoung, J. Electrochem. Soc., 1997, 144, 3881–3886 CrossRef CAS.
- P. A. Z. Suarez, J. E. L. Dullius, S. Einloft, R. F. DeSouza and J. Dupont, Polyhedron, 1996, 15, 1217–1219 CrossRef CAS.
- P. Bonhote, A. P. Dias, N. Papageorgiou, K. Kalyanasundaram and M. Gratzel, Inorg. Chem., 1996, 35, 1168–1178 CrossRef CAS PubMed.
- M. Kermanioryani, M. I. A. Mutalib, Y. Dong, K. C. Lethesh, O. B. Ben Ghanem, K. A. Kurnia, N. F. Aminuddin and J. M. Leveque, J. Chem. Eng. Data, 2016, 61, 2020–2026 CrossRef CAS.
- E. Gomez, N. Calvar, A. Dominguez and E. A. Macedo, Ind. Eng. Chem. Res., 2013, 52, 2103–2110 CrossRef CAS.
- D. Rooney, J. Jacquemin and R. L. Gardas, Ionic Liq., 2009, 290, 185–212 CAS.
- C. P. Fredlake, J. M. Crosthwaite, D. G. Hert, S. Aki and J. F. Brennecke, J. Chem. Eng. Data, 2004, 49, 954–964 CrossRef CAS.
- H. L. Ngo, K. LeCompte, L. Hargens and A. B. McEwen, Thermochim. Acta, 2000, 357, 97–102 CrossRef.
- H. Xue, R. Verma and J. M. Shreeve, J. Fluorine Chem., 2006, 127, 159–176 CrossRef CAS.
- N. Nishi, A. Suzuki and T. Kakiuchi, Bull. Chem. Soc. Jpn., 2009, 82, 86–92 CrossRef CAS.
- Z. B. Zhou, H. Matsumoto and K. Tatsumi, Chem.–Eur. J., 2006, 12, 2196–2212 CrossRef CAS PubMed.
- Z. B. Zhou, H. Matsumoto and K. Tatsumi, Chem.–Eur. J., 2005, 11, 752–766 CrossRef CAS PubMed.
- Z. B. Zhou, M. Takeda and M. Ue, J. Fluorine Chem., 2004, 125, 471–476 CrossRef CAS.
- K. Iwasaki, K. Yoshii, S. Tsuzuki, T. Tsuda and S. Kuwabata, in Molten Salts and Ionic Liquids 19, Electrochemical Soc Inc, Pennington, 2014, vol. 64, pp. 83–93 Search PubMed.
- M. Shakourian-Fard, Z. Jamshidi, A. Bayat and A. Fattahi, J. Fluorine Chem., 2013, 153, 96–100 CrossRef CAS.
- S. Tsuzuki, T. Umecky, H. Matsumoto, W. Shinoda and M. Mikami, J. Phys. Chem. B, 2010, 114, 11390–11396 CrossRef CAS PubMed.
- N. Terasawa, S. Tsuzuki, T. Umecky, Y. Saito and H. Matsumoto, Chem. Commun., 2010, 46, 1730–1732 RSC.
- N. V. Ignat'ev, A. Kucheryna, G. Bissky and H. Willner, in Ionic Liquids Iv: Not Just Solvents Anymore, Amer Chemical Soc, Washington, 2007, vol. 975, pp. 320–334 Search PubMed.
- N. Nishi, S. Imakura and T. Kakiuchi, Anal. Chem., 2006, 78, 2726–2731 CrossRef CAS PubMed.
- G. Singh, G. Singh and T. S. Kang, J. Phys. Chem. B, 2016, 120, 1092–1105 CrossRef CAS PubMed.
- W. W. Xu, T. Wang, N. Cheng, Q. Z. Hu, Y. H. Bi, Y. J. Gong and L. Yu, Langmuir, 2015, 31, 1272–1282 CrossRef CAS PubMed.
- H. Wang, X. Q. Xu, J. F. Shi and G. Xu, Acta Phys.-Chim. Sin., 2013, 29, 525–532 CrossRef CAS PubMed.
- Y. Q. Gu, L. J. Shi, X. Y. Cheng, F. Lu and L. Q. Zheng, Langmuir, 2013, 29, 6213–6220 CrossRef CAS PubMed.
- A. Marciniak, Fluid Phase Equilib., 2010, 294, 213–233 CrossRef CAS.
- D. Han and K. H. Row, Molecules, 2010, 15, 2405–2426 CrossRef CAS PubMed.
- V. V. Bardin, I. K. Shundrina and H. J. Frohn, J. Fluorine Chem., 2014, 157, 73–78 CrossRef CAS.
- N. Y. Adonin and V. V. Bardin, Russ. Chem. Rev., 2010, 79, 757–785 CrossRef CAS.
- H. J. Frohn, H. Franke, P. Fritzen and V. V. Bardin, J. Organomet. Chem., 2000, 598, 127–135 CrossRef CAS.
- A. Y. Shabalin, N. Y. Adonin, V. V. Bardin, S. A. Prikhod'ko, M. N. Timofeeva, M. V. Bykova and V. N. Parmon, J. Fluorine Chem., 2013, 149, 82–87 CrossRef CAS.
- V. V. Bardin, I. K. Shundrina and H. J. Frohn, J. Fluorine Chem., 2014, 157, 73–78 CrossRef CAS.
- M. Kick, P. Keil and A. Konig, Fluid Phase Equilib., 2013, 338, 172–178 CrossRef CAS.
- Z. B. Zhou, H. Matsumoto and K. Tatsumi, ChemPhysChem, 2005, 6, 1324–1332 CrossRef CAS PubMed.
- D. Bankmann and R. Giernoth, Prog. Nucl. Magn. Reson. Spectrosc., 2007, 51, 63–90 CrossRef CAS.
- R. D. Falcone, B. Baruah, E. Gaidamauskas, C. D. Rithner, N. M. Correa, J. J. Silber, D. C. Crans and N. E. Levinger, Chem.–Eur. J., 2011, 17, 6837–6846 CrossRef CAS PubMed.
- M. N. Garaga, M. Nayeri and A. Martinelli, J. Mol. Liq., 2015, 210, 169–177 CrossRef CAS.
- B. A. Marekha, O. N. Kalugin, M. Bria and A. Idrissi, Phys. Chem. Chem. Phys., 2015, 17, 23183–23194 RSC.
- H. Shirota, S. Kakinuma, Y. Itoyama, T. Umecky and T. Takamuku, J. Phys. Chem. B, 2016, 120, 513–526 CrossRef CAS PubMed.
- S. Cha, M. Ao, W. Sung, B. Moon, B. Ahlstrom, P. Johansson, Y. Ouchi and D. Kim, Phys. Chem. Chem. Phys., 2014, 16, 9591–9601 RSC.
- H. Kato, K. Miki, T. Mukai, K. Nishikawa and Y. Koga, J. Phys. Chem. B, 2009, 113, 14754–14760 CrossRef CAS PubMed.
- J. Bowers, C. P. Butts, P. J. Martin, M. C. Vergara-Gutierrez and R. K. Heenan, Langmuir, 2004, 20, 2191–2198 CrossRef CAS PubMed.
- M. Vranes, S. Armakovic, A. Tot, S. Papovic, N. Zec, S. Armakovic, N. Banic, B. Abramovic and S. Gadzuric, J. Chem. Thermodyn., 2016, 93, 164–171 CrossRef CAS.
- U. Domanska, Pure Appl. Chem., 2005, 77, 543–557 CrossRef.
- J. B. Harper and R. M. Lynden-Bell, Mol. Phys., 2004, 102, 85–94 CrossRef CAS.
- T. Gutel, C. C. Santini, A. A. H. Padua, B. Fenet, Y. Chauvin, J. N. C. Lopes, F. Bayard, M. F. C. Gomes and A. S. Pensado, J. Phys. Chem. B, 2009, 113, 170–177 CrossRef CAS PubMed.
- T. Shimomura, T. Takamuku and T. Yamaguchi, J. Phys. Chem. B, 2011, 115, 8518–8527 CrossRef CAS PubMed.
- J. D. Holbrey, W. M. Reichert, M. Nieuwenhuyzen, O. Sheppard, C. Hardacre and R. D. Rogers, Chem. Commun., 2003, 476–477 RSC.
- J. F. B. Pereira, L. A. Flores, H. Wang and R. D. Rogers, Chem.–Eur. J., 2015, 20, 15482–15492 CrossRef PubMed.
- N. Y. Adonin, D. E. Babushkin, V. N. Parmon, V. V. Bardin, G. A. Kostin, V. I. Mashukov and H. J. Frohn, Tetrahedron, 2008, 64, 5920–5924 CrossRef CAS.
Footnote |
† Electronic supplementary information (ESI) available: Spectral information on all ionic liquids and results of viscosity measurements. See DOI: 10.1039/c7ra01709j |
|
This journal is © The Royal Society of Chemistry 2017 |
Click here to see how this site uses Cookies. View our privacy policy here.