DOI:
10.1039/C7RA02298K
(Paper)
RSC Adv., 2017,
7, 20394-20397
I2/TBHP-mediated oxidative coupling of ketones and toluene derivatives: a facile method for the preparation of α-benzoyloxy ketones†
Received
24th February 2017
, Accepted 30th March 2017
First published on 7th April 2017
Abstract
An efficient oxidative approach was developed for the α-benzoyloxylation of ketones using tert-butyl hydroperoxide (TBHP). This process provided facile access to a wide range of α-benzoyloxy ketones in good to excellent yields via the direct oxidative α-benzoyloxylation of a structurally diverse series of ketones using simple arylmethane compounds.
Introduction
α-Acyloxycarbonyl compounds have received considerable interest from the organic chemistry community during the last few years because of their unique structural properties. Furthermore, compounds belonging to this structural class can be found in a wide variety of biologically interesting natural products, pharmaceuticals and synthetic intermediates.1 Traditionally, these compounds are prepared by the substitution reaction of a functionalized carbonyl compound with an alkaline carboxylate or carboxylic acid,2 as well as the direct oxidative coupling of a ketone with a carboxylic acid (or surrogates),3 all of which require the use of a highly toxic heavy metal oxidant.4 Several interesting alternatives have been developed to avoid the many drawbacks associated with the use of toxic heavy metals. Most notably, organohypervalent iodine reagents and peroxides have been widely used as surrogates for heavy metal oxidants to promote the α-benzoyloxylation of ketones.5 More recently, there have been several reports pertaining to the use oxyacylating reagents, such as aldehydes, benzylic alcohols and acetophenones, as surrogates of carboxylic acids.6 However, to the best of our knowledge, there have been very few reports describing the α-benzoyloxylation of ketones using toluene derivatives as oxyacylating reagents. Li and co-workers recently reported an efficient method for the α-benzoyloxylation of ketones using toluene derivatives as oxyacylating reagents under metal-free conditions (eqn (1)).7 However, the substrate scope of this procedure was limited to α-substituted ketones, whereas acetophenones and 2-iodo-1-phenylpropan-1-one were found to be unsuitable for this transformation. In addition, this method afforded low yields of the desired product. Hence, the development of novel, environmentally benign and high yielding procedures for the preparation of α-acetoxy ketones from simple and readily available starting materials with a broad substrate scope is still highly desirable.
Rather than only serving as effective solvents,8 toluene and its derivatives can also be used as reagents in organic synthesis for the construction of structurally complex compounds.9 Herein, we report the development of a novel route for the facile α-benzoylxoylation of a wide range of ketone substrates (e.g., acetophenones, propiophenones, butyrylbenzenes, heterocyclic arones and aliphatic ketones) using toluene and its derivatives as oxyacylating precursors in the presence of tert-butyl hydroperoxide (TBHP) and iodine to the synthesis of α-benzoylxoy ketones (eqn (2)). The key features of this new oxidation system include (i) metal-free oxidation conditions; (ii) the use of toluene and its derivatives as readily available and inexpensive oxyacylating precursors; (iii) a broad substrate scope, including aryl ketones, heterocyclic ketones, aliphatic ketones, toluenes, phenylmethanols, benzaldehydes, benzoic acids and ethylbenzenes; and (iv) milder reaction conditions than existing methods for this transformation.
|
 | (1) |
|
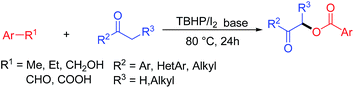 | (2) |
Results and discussion
The reaction of toluene (1a) with acetophenone (2a) was initially selected as a model reaction to determine the optimum conditions for this transformation (Table 1). The results revealed that the reaction was completed after 24 h, with no further increases observed in the product yield following extended reaction time (Table 1, entries 1–3). It is noteworthy that no reaction occurred in the absence of TBHP or iodine (I2), which indicated that these two reagents were critical to the success of the reaction (Table 1, entries 4–5). Notably, we observed a remarkable increase in the product yield when the amount of TBHP was increased to 5.0 equivalents. Further increasing the amount of TBHP to 7.0 or 9.0 equivalents did not result in any further increases in the yield, indicating that the optimum value of this reagent was 5.0 equivalents (Table 1, entries 6–8). We subsequently evaluated a variety of different peroxides in this reaction, including di-tert-butyl peroxide (DTBP), benzoyl peroxide, dicumyl peroxide (DCP), cumene hydroperoxide (CHP) and potassium hydrogen persulfate. However, all of these peroxides afforded much lower yields of the desired product than TBHP except benzoyl peroxide (Table 1, entries 9–13). We then proceeded to investigate the effects of adding different amounts of I2 and Na2CO3 to the reaction. The results of these screening experiments indicated that 1.0 equivalent was the optimal charge for both I2 and Na2CO3 (Table 1, entries 14–17). Notably, this reaction failed to afford the desired product when it was conducted in the absence of Na2CO3 (Table 1, entry 17). Finally, we did not observe any changes in the product yield when the reaction was performed under a N2 atmosphere (Table 1, entry 18).
Table 1 Optimization of reaction conditionsa
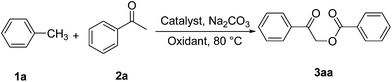
|
Entry |
Cat. (1.0 equiv.) |
Peroxide (equiv.) |
Time (h) |
Yieldb% |
Unless otherwise specified, all of these reactions were carried out as follows: 2a (0.25 mmol scale), catalyst (1.0 equiv.) and Na2CO3 (1.0 equiv.) in toluene (2.0 mL). GC yield. Iodine (0.2 equiv.). Iodine (1.5 equiv.). Na2CO3 (0.5 equiv.). Without Na2CO3. N2 (balloon). |
1 |
I2 |
Tert-butyl hydroperoxide (TBHP) (3) |
12 |
17 |
2 |
I2 |
TBHP (3) |
24 |
24 |
3 |
I2 |
TBHP (3) |
36 |
24 |
4 |
I2 |
— |
24 |
0 |
5 |
— |
TBHP (3) |
24 |
0 |
6 |
I2 |
TBHP (5) |
24 |
79 |
7 |
I2 |
TBHP (7) |
24 |
86 |
8 |
I2 |
TBHP (9) |
24 |
86 |
9 |
I2 |
Di-tert-butyl peroxide (DTBP) (5) |
24 |
39 |
10 |
I2 |
Benzoyl peroxide (5) |
24 |
83 |
11 |
I2 |
Dicumyl peroxide (DCP) (5) |
24 |
58 |
12 |
I2 |
Cumene hydroperoxide (CHP) (5) |
24 |
36 |
13 |
I2 |
Potassium hydrogen persulfate (5) |
24 |
71 |
14c |
I2 |
TBHP (5) |
24 |
59 |
15d |
I2 |
TBHP (5) |
24 |
86 |
16e |
I2 |
TBHP (5) |
24 |
66 |
17f |
I2 |
TBHP (5) |
24 |
0 |
18g |
I2 |
TBHP (5) |
24 |
86 |
With the optimized conditions in hand, we proceeded to explore the scope of this newly developed α-benzoyloxylation reaction using a wide range of different ketones with toluene (1a) (Scheme 2). Acetophenones 2a–h and propiophenones 2l–o bearing a variety of electron-rich and electron-poor substituents were all tolerated under the optimized reaction conditions. Substrates bearing electron-withdrawing substituents (i.e., F−, Cl− and O2N−) performed more effectively in this reaction than those bearing electron-donating substituents (i.e., Me− and Methoxy−), to give the corresponding α-oxyacylated products 2 in relatively high yield. It is noteworthy that the yield of this α-benzoyloxylation reaction appeared to be largely unaffected by the position of the substituents on the phenyl ring. For example, 1-p-tolylethanone, 1-o-tolylethanone and 1-m-tolylethanone gave almost identical yields of the corresponding products. In addition, the inclusion of different R2 groups (R2 = H, Me, Et) had no discernible impact on the outcome of this transformation. Pleasingly, we found that this newly developed procedure was also amenable to heteroaryl (such as thienyl and furyl, see 3ai, 3aj and 3ap) and alkyl (3ak) ketones.
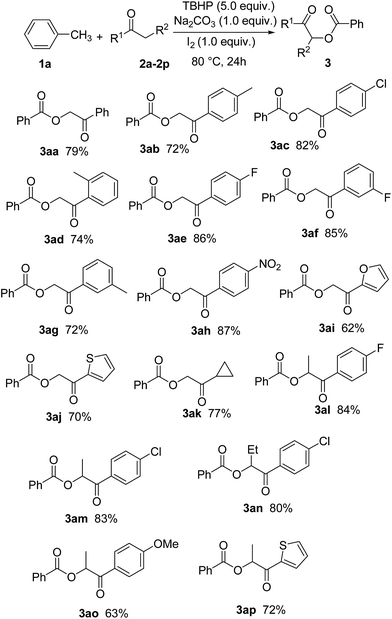 |
| Scheme 1 α-Benzoyloxylation of different ketones (all of these reactions were carried out on 2 (1.0 mmol) in toluene (2.0 mL)). | |
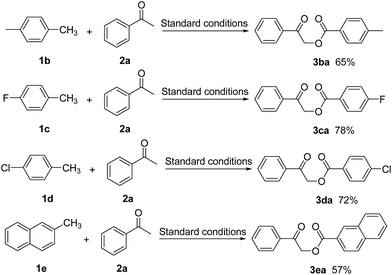 |
| Scheme 2 α-Benzoyloxylation of acetophenone with different arylmethanes (all of these reactions were carried out on 2a (1.0 mmol) with 2.0 mL of 1). | |
We also investigated the scope of substituted arylmethane used in this reaction and the results are summarized in Scheme 2. The results revealed that a variety of different substituents, such as Me−, Cl− and F−, were tolerated on the phenyl group of the toluene additive, with no discernible difference in the yield of the α-oxyacylated products compared with toluene. Most notably, 2-methylnaphthalene (1e) reacted with acetophenone (2a) to give the corresponding product 3ea in an isolated yield of 57%.
To further extend the scope of this reaction, we also tested the possibility of using phenylmethanol, benzaldehyde, benzoic acid and ethylbenzene as substrates (Scheme 3). Pleasingly, all of these substrates reacted efficiently with acetophenone to afford 1-oxo-1-phenylethyl benzoate (3aa) in 67–93% yields. Benzoic acid, in particular, afforded the desired product with almost quantitative yield. These results therefore clearly indicate that phenylmethanol, benzaldehyde, benzoic acid and ethylbenzene are suitable alternatives of toluene for this transformation, thereby greatly expanding the substrate scope of this reaction.
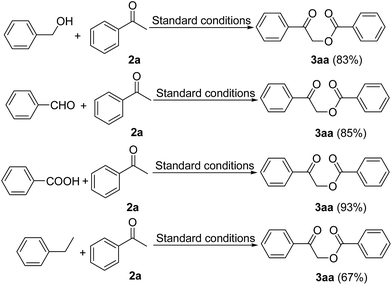 |
| Scheme 3 α-Benzoyloxylation reactions of acetophenone with phenylmethanol, benzaldehyde, benzoic acid and ethylbenzene. | |
The following control experiments were carried out to gain an insight into the mechanism of our newly developed reaction (Scheme 4). The addition of an excess of the radical scavenger TEMPO under the standard conditions completely inhibited the α-benzoyloxylation reaction, suggesting that this transformation proceeds via a radical pathway. Fortunately, 2-iodo-1-phenylethanone worked well as a surrogate for acetophenone under our standard reaction conditions, affording an almost quantitative yield of the desired α-oxyacylated products, which suggested that 2-iodo-1-phenylethanone was being formed as an intermediate in this reaction. However, this reaction failed to afford the desired α-oxyacylated products when it was conducted in the absence of TBHP. Remarkably, the addition of tert-butylperoxybenzoate to 2a resulted in the formation of the desired α-benzoxylation product 3aa in 93% yield. In addition, we noticed that these reactions afforded 3aa in excellent yields when phenylmethanol, benzaldehyde and benzoic acid were used as surrogates for acetophenone (Scheme 2). These findings therefore suggested that benzoic acid or tert-butylperoxybenzoate was being formed in situ as an intermediate from toluene.
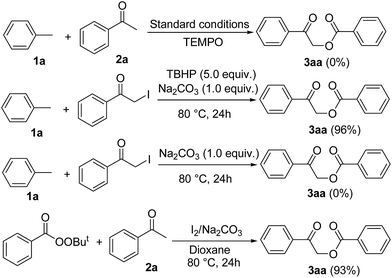 |
| Scheme 4 Control experiments to determine the reaction mechanism. | |
Based on the results above and previous reports from the literature, we proposed a plausible mechanism for the current α-benzoyloxylation reaction, which is shown in Scheme 5. The intial reaction of acetophenone (2a) with molecular iodine would afford 2-iodo-1-phenylethanone A,10 which would be oxidized by TBHP to produce α-carbonyl radical B.11 Toluene (1a) would also be oxidized by TBHP to produce the radical intermediate C, which would be further oxidized to form the tert-butylperoxybenzoate intermediate E.12 The subsequent coupling of B and E would lead to the formation of the desired product 3aa, in a manner similar to that reported by Li.7 Alternatively, in the presence of TBHP, C could be further converted to carboxylate anion D.7,13 Finally, the reaction of A with D affords the final product 3aa.
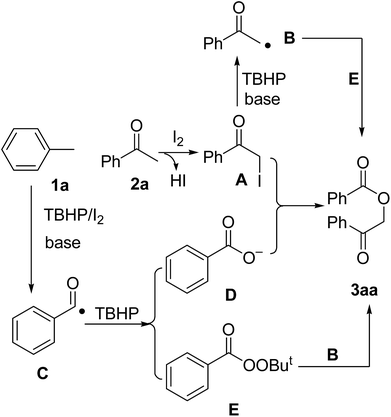 |
| Scheme 5 A reaction pathway for the formation of 3aa. | |
Experimental
General information
All the reactions were carried out at 80 °C for 24 h in a round-bottom flask equipped with magnetic stir bar. Unless otherwise stated, all reagents and solvents were purchased from commercial suppliers and used without further purification. Melting points were measured on a melting point apparatus equipped with a thermometer. 1H NMR and 13C NMR spectra were recorded on a 400 MHz spectrometer in solutions of CDCl3 using tetramethylsilane as the internal standard, δ values are given in ppm and coupling constants (J) in Hz. HR-MS were obtained on a Q-TOF micro spectrometer.
Typical procedure: 1-oxo-1-phenylethyl benzoate (Scheme 1, 3aa)
A mixture of acetophenone (2a) (120 mg, 1.0 mmol), Na2CO3 (106 mg, 1.0 mmol), TBHP (70% aqueous solution, 0.65 g, 5.0 mmol), iodine (254 mg, 1.0 mmol) and toluene (2.0 mL) was added successively in a round-bottom flask, and the resulting soln stirred for 24 h at 80 °C. The mixture was purified by column chromatography on silica gel to afford product 3aa with PE/ethyl acetate as the eluent.
Conclusions
In summary, we have developed an efficient approach for the facile preparation of α-benzoyloxy ketones by the direct oxidative α-benzoyloxylation of a structurally diverse range of ketones using simple arylmethane compounds in good to excellent yields. It is noteworthy that the TBHP oxidant used in this reaction also acted as a source of oxygen for the introduction of the benzoyloxy group.
Acknowledgements
We thank the Guangdong Provincial Department of Science and Technology (No. 916014) and the Department of Education of Guangdong Province (No. 916021) for financial support.
Notes and references
- M. Shun-Ichi, S. Takao, H. Hidenori, M. Yoshihide, N. Takeshi, K. Hidenori and A. Susumu, J. Org. Chem., 1993, 58, 2929 CrossRef; T. W. Green and P. G. M. Wuts, Protective Groups in Organic Synthesis, Wiley, New York, 1991, p. 87 Search PubMed.
- T. Ara and K. Z. Khan, J. Pharm. Res., 2014, 8, 828 Search PubMed; J. H. Clark and J. M. Miller, Tetrahedron Lett., 1977, 7, 599 CrossRef CAS.
- L. M. Chang and G. Q. Yuan, Tetrahedron, 2016, 72, 7003 CrossRef CAS; Y. D. Wu, B. Huang, Y. X. Zhang, X. X. Wang, J. J. Dai, J. Xu and H. J. Xu, Org. Biomol. Chem., 2016, 14, 5936 Search PubMed.
- K. P. Malova and F. Hammerschmidt, Eur. J. Org. Chem., 2013, 5143 CrossRef CAS PubMed; V. Cadierno, J. Francos and J. Gimeno, Organometallics, 2011, 30, 852 CrossRef.
- M. Uyanik, D. Suzuki, T. Yasui and K. Ishihara, Angew. Chem., Int. Ed., 2011, 123, 5443 CrossRef CAS; R. N. Reddi, P. K. Prasad and A. Sudalai, Org. Lett., 2014, 16, 5674 CrossRef PubMed.
- R. N. Reddi, P. K. Prasad and A. Sudalai, Org. Biomol. Chem., 2013, 11, 6477 Search PubMed; S. J. Guo, J. T. Yu, Q. Dai, H. T. Yang and J. Cheng, Chem. Commun., 2014, 50, 6240 RSC; J. Du, X. L. Zhang, X. Sun and L. Wang, Chem. Commun., 2015, 51, 4372 RSC.
- C. L. Li, T. Jin, X. L. Zhang, C. J. Li, X. S. Jia and J. Li, Org. Lett., 2016, 18, 1916 CrossRef CAS PubMed.
- H. Zong, H. Y. Huang, G. L. Bian and L. Song, J. Org. Chem., 2014, 79, 11768 CrossRef CAS PubMed; J. D. O. Silva, R. A. Angnes, V. H. M. Silva, B. M. Servilha, M. A. A. C. Braga, A. Aponick and C. R. D. Correia, J. Org. Chem., 2016, 81, 2010 CrossRef PubMed.
- Z. Q. Liu, X. L. Zhang, J. X. Li, F. Li, C. J. Li, X. S. Jia and J. Li, Org. Lett., 2016, 18, 4052 CrossRef CAS PubMed; Y. Çimen, S. Akyüz and H. Türk, New J. Chem., 2015, 39, 3894 RSC.
- Q. H. Gao, X. Wu, S. Liu and A. X. Wu, Org. Lett., 2014, 16, 1732 CrossRef CAS PubMed; Q. H. Gao, X. Wu, S. Liu and A. X. Wu, Org. Lett., 2014, 16, 4582 CrossRef PubMed; H. F. Jiang, H. W. Huang, H. Cao and C. R. Qi, Org. Lett., 2010, 12, 5561 CrossRef PubMed.
- K. S. Vadagaonkar, H. P. Kalmode, K. Murugan and A. C. Chaskar, RSC Adv., 2015, 5, 5580 RSC; N. Mupparapu, R. A. Vishwakarma and Q. N. Ahmed, Tetrahedron, 2015, 71, 3417 CrossRef CAS; P. S. Baran and J. M. Richter, J. Am. Chem. Soc., 2004, 126, 7450 CrossRef PubMed; K. Xu, Y. Fang, Z. Yan, Z. Zha and Z. Wang, Org. Lett., 2013, 15, 2148 CrossRef PubMed.
- S. K. Rout, S. Guin, W. Ali, A. Gogoi and B. K. Patel, Org. Lett., 2014, 16, 3086 CrossRef CAS PubMed; G. Majji, S. Guin, A. Gogoi, S. K. Rout and B. K. Patel, Chem. Commun., 2013, 49, 3031 RSC.
- A. Al-Hunaiti, T. Niemi, A. Sibaouih, P. Pihko, M. Leskelä and T. Repo, Chem. Commun., 2010, 46, 9250 RSC; S. Mannam and G. Sekar, Tetrahedron Lett., 2008, 49, 2457 CrossRef CAS.
Footnote |
† Electronic supplementary information (ESI) available. See DOI: 10.1039/c7ra02298k |
|
This journal is © The Royal Society of Chemistry 2017 |
Click here to see how this site uses Cookies. View our privacy policy here.