DOI:
10.1039/C7RA03262E
(Paper)
RSC Adv., 2017,
7, 20548-20552
Rh(III)-catalyzed sequential C–H activation and annulation: access to N-fused heterocycles from arylazoles and α-diazocarbonyl compounds†
Received
20th March 2017
, Accepted 29th March 2017
First published on 10th April 2017
Abstract
A mild and simple protocol has been developed for the synthesis of N-fused heterocycles from arylazoles and α-diazocarbonyl compounds via Rh(III)-catalyzed sequential C–H activation and annulation. Three kinds of N-fused heterocycles derived from arylpyrazoles, arylbenzimidazoles and aryl 1,2,4-triazole were successfully obtained. The reactivity of the other four arylazole analogues was also investigated.
Introduction
N-fused heterocycles are important due to their widespread applications in the pharmaceutical industry and advanced materials.1,2 Synthesis of various N-fused heterocycles has thus attracted much attention, particularly for drug development.3 Most syntheses have been completed via traditional approaches under harsh reaction conditions like high temperature and microwave irradiation.4 The past decades have seen the development of numerous protocols via transition-metal-catalyzed coupling for this purpose,5 among which Cu- or Pd-catalyzed coupling reactions6 and transition-metal-catalyzed C–H activation7 represent two attractive strategies in forming carbon–carbon and carbon–heteroatom bonds. For instance, Fu et al. reported several elegant examples of copper-catalyzed cascade reactions to access N-fused heterocycles from aryl halides and active methylene compounds in 2011 and 2012 (Scheme 1a).6a,b,d Later, Macgregor and co-workers reported a more direct Rh- or Ru-catalyzed synthesis of pyrazoloisoquinolines using C–H activation of 3-phenylpyrazoles with aryl- and alkylalkynes7f, avoiding the use of aryl halides; however, an excess amount of oxidant was needed (Scheme 1b). Therefore, the development of a more efficient and greener synthetic approach to access N-fused heterocycles via C–H activation remains highly desirable.
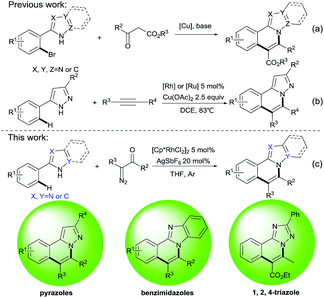 |
| Scheme 1 Synthesis of N-fused heterocycles from arylazoles. | |
Carbene migratory insertion is a well established method to directly functionalize C–H bonds,8 with α-diazocarbonyl compounds predominantly used in the directing group (DG)-assisted Rh(III)-, Co(III)- and Ru(II)-catalyzed C–H activation.9 In 2012, Yu and co-workers first developed chelation-assisted Rh(III)-catalyzed intermolecular cross-coupling of diazomalonates with arene C–H bonds.10 Recently, Li et al. developed a new Ru(II)-catalyzed intermolecular coupling of diazo substrates with arenes to access indoles,11 and the Glorius group reported the first example of Co(III)-catalyzed C–H bond activation of imines with diazo compounds for the synthesis of isoquinolin-3-ones.12 Directing groups previously used in this type of transformation included, among others, imines,13 oximes,14 amines,15 hydrazines,16 azides,17 azobenzenes18 and amides,19 delivering the corresponding isoquinolines, benzothiazine, lactams, isoquinolones, indoles and indolines. In view of the importance of N-fused heterocycles and our continued interest in Rh(III)-catalyzed C–H activation reactions and the construction of N-fused heterocycles,20 we envision using arylazoles and diazo compounds as the substrates in a Rh(III)-catalyzed sequential C–H activation and annulation that would provide valuable N-fused heterocycles (Scheme 1c).
Results and discussion
To verify the assumption mentioned above, 3,5-diphenyl-1H-pyrazole (1a) and ethyl 2-diazo-3-oxobutanoate (2a) were selected as coupling partners (Table 1). We studied the reaction between 1a and 2a in the presence of [Cp*RhCl2]2 (5 mol%) and AgSbF6 (20 mol%) at room temperature under Ar atmosphere in THF (2 mL) for 12 h. Fortunately, the desired product 3aa was obtained in 37% yield (Table 1, entry 1). Encouraged by this result, three other solvents were tested, and the results revealed that THF was the best (Table 1, compare entries 2–4). Then, considering the conversion of the substrate, the reaction time was prolonged to 24 h, and we were pleased to see that the yield of 3aa directly increased to 92% (Table 1, entry 5). Furthermore, to investigate the catalytic activity of other transition-metal catalysts, the performances of four other metal catalyst systems were compared. No desired product 3aa was obtained using [Cp*(IrCl2)]2/AgNTf2 or Ru(PPh3)3Cl2/AgSbF6 as the catalyst system (Table 1, entries 7 and 8). Cp*Co(CO)I2/AgSbF6 was less effective; the desired product 3aa was obtained in 24% yield (Table 1, entry 6). By changing the silver salt to AgNTf2, the yield of 3aa declined to 75%. In addition, no conversion of the substrate was observed without Ag salt or Rh salt (Table 1, entries 10, 11 and 12).
Table 1 Rh(III)-catalyzed sequential C–H activation and annulation of 3,5-diphenyl-1H-pyrazole (1a) with ethyl 2-diazo-3-oxobutanoate (2a): optimization of conditionsa
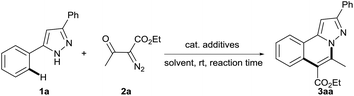
|
Entry |
Cat. (mol%) |
Reaction time |
Solvent |
Yieldb (%) |
Reaction conditions: 1a (0.2 mmol), 2a (0.25 mmol), catalyst, and solvent (2 mL) were stirred at room temperature under Ar.
Isolated yield.
|
1 |
[Cp*RhCl2]2 (5)/AgSbF6 (20) |
12 h |
THF |
37 |
2 |
[Cp*RhCl2]2 (5)/AgSbF6 (20) |
12 h |
DCE |
Trace |
3 |
[Cp*RhCl2]2 (5)/AgSbF6 (20) |
12 h |
MeOH |
Trace |
4 |
[Cp*RhCl2]2 (5)/AgSbF6 (20) |
12 h |
EtOH |
9 |
5
|
[Cp*RhCl2]2 (5)/AgSbF6 (20)
|
24 h
|
THF
|
92
|
6 |
Cp*Co(CO)I2 (5)/AgSbF6 (20) |
24 h |
THF |
24 |
7 |
[Cp*(IrCl2)]2 (5)/AgNTf2 (20) |
24 h |
THF |
Trace |
8 |
Ru(PPh3)3Cl2 (5)/AgSbF6 (20) |
24 h |
THF |
0 |
9 |
[Cp*RhCl2]2 (5)/AgNTf2 (20) |
24 h |
THF |
75 |
10 |
None |
24 h |
THF |
0 |
11 |
[Cp*RhCl2]2 (5) |
24 h |
THF |
0 |
12 |
AgSbF6 (20) |
24 h |
THF |
0 |
Having established the feasibility of Rh(III)-catalyzed sequential C–H activation and annulation to deliver N-fused heterocycles, the reactions of various 5-phenyl-1H-pyrazoles with α-diazocarbonyl compounds were explored to examine the scope and generality of the present process. As depicted in Table 2, at first, 3,5-diphenyl-1H-pyrazole (1a) was kept as the representative reaction partner. A variety of diazoketoesters participated well in this transformation, affording the corresponding products in 84–99% yields (3aa–3ae). α-Diazo-β-diketo substrates 2f–h also showed good efficiency in the cyclization to give the desired products in good yields. Among them, unsymmetrical diketone 2h performed well; only one regioisomer of 3ah was obtained in 72% yield. Then we found that the reaction of 3-phenyl-5-(p-tolyl)-1H-pyrazole (1b) with methyl 2-diazo-3-oxobutanoate (2b) afforded a mixture of regioisomeric products 3bb and 3bb′ in 97% total yield with a ratio of 1
:
1 based on NMR analysis of the crude mixture. For 5-methyl-3-phenyl-1H-pyrazole, substituents at the ortho or para position including methyl, methoxy, trifluoromethyl, iodo, fluoro, chloro or bromo showed less reactivity; by raising the reaction temperature to 60 °C, all reacted well with diazoketoester (2b) and symmetrical diketone 2i, which was prepared from cyclohexane-1,3-dione, providing 3cb–3lb and 3ci in good yields. Interestingly, a methyl substituent at the meta position (1m) underwent the transformation with good regioselectivity to give a single regioisomer in 77% yield (3mb). However, the reaction of the methoxy substituent at the meta position (1n) gave separable regioisomeric products 3nb and 3nb′ in 25% and 74% yield, respectively. Additionally, 3-methyl-5-(thiophen-3-yl)-1H-pyrazole (1o) also presented good reactivity in this reaction, giving the desired product 3ob in 99% yield. Pleasingly, a gram-scale reaction was also conducted under standard conditions, and 3aa was obtained in 95% yield.
Table 2 Rh(III)-catalyzed sequential C–H activation and annulation of substituted 3,5-diphenyl-1H-pyrazole with α-diazocarbonyl compoundsa,b
Reaction conditions: 1 (0.2 mmol), 2 (0.25 mmol), [Cp*RhCl2]2 (5 mol%)/AgSbF6 (20 mol%), and THF (2 mL) were stirred at room temperature (ca. 25 °C) under Ar for 24 h.
Isolated yield.
The reaction temperature is 60 °C.
The reaction time is 48 h.
The reaction temperature is 40 °C.
|
|
Next, other arylazoles were examined to broaden the scope of this protocol (Table 3). Unfortunately, only the coupling of 2-phenylbenzimidazole (1p) with 2-diazo-3-oxobutanoate (2a) was successful under the optimal reaction conditions above, affording the coupling product 4a in 95% yield. The structure of 4a was confirmed by NMR and HRMS (see ESI†). To our delight, by raising the reaction temperature to 100 °C, the cyclization product 3pa was isolated in 63% yield. This indicates the difference in N-nucleophilicity among indole, pyrazole and imidazole, which is consistent with previous reports.6e,7m,n At the same reaction temperature, other α-diazocarbonyl compounds also reacted with 1p, giving 3pa–3ph in moderate yields. Chloro and trifluoromethyl substituents of 2-phenylbenzimidazole at the para position were readily converted into the desired products in good yields (3qc, 3ra, 3rb). Notably, this tandem reaction was also compatible with 5-phenyl-1H-1,2,4-triazole to form 3sa in 51% yield. However, for other arylazoles, including aryl imidazole, aryl dihydroimidazole, aryl 1,2,3-triazole and aryl tetrazole, no desired product was observed under our conditions (3tb–3wb).
Table 3 Rh(III)-catalyzed sequential C–H activation and annulation of other arylazoles with α-diazocarbonyl compoundsa,b
Reaction conditions: 1 (0.2 mmol), [Cp*RhCl2]2 (5 mol%)/AgSbF6 (20 mol%), and THF (2 mL) were stirred at 100 °C under Ar for 24 h.
Isolated yield.
The reaction temperature is 60 °C.
|
|
To better understand this chemistry, we used Density Functional Theory (DFT) calculations to study the activation energy, specifically with regard to the C–H activation step.21 The C–H bond activation is facilitated by the basic pyrazole group in the substrate S1 via TS1 with an activation energy of 26.2 kcal mol−1. However, the activation energy increases to 33.2 kcal mol−1 when we use imidazole S2 as the substrate (ESI Fig. 1†). These results are consistent with the experimental observations.
Considering the previous reports9–21 on chelation-assisted Rh(III)-catalyzed intermolecular cross-coupling of diazo compounds and the formation of coupling product 4a described above, we believe that this protocol likely involves sequential C–H activation and intramolecular cyclization; thus a plausible mechanism is proposed (Scheme 2). To begin with, anion exchange occurs between [Cp*RhCl2]2 and AgSbF6, followed by the formation of a cationic Rh(III) species. Then the arylazole 1 undergoes directed C–H cleavage with Cp*Rh(III) to give a five-membered rhodacyclic intermediate I. Next, the diazo compound 2 coordinates with it to form the intermediate II. Subsequently, migratory insertion of the carbene into the Rh–C bond gives the intermediate III, which undergoes proto-demetalation to afford the alkylated product IV, releasing the Rh(III) catalyst for a new catalytic cycle. Finally, an enol intermediate is generated in situ by the tautomerization of intermediate IV, and gives the final product 3via nucleophilic cyclization.
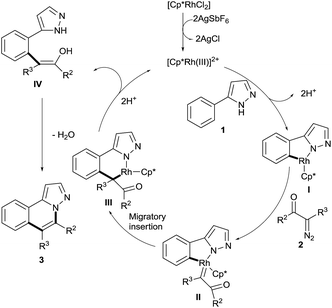 |
| Scheme 2 Proposed reaction pathway. | |
Conclusions
To sum up, we have developed a facile and practical method for the synthesis of valuable N-fused heterocycles via Rh(III)-catalyzed sequential C–H activation and annulation, using arylazoles and α-diazocarbonyl compounds as substrates. In this protocol, pyrazoles, benzimidazoles and one 1,2,4-triazole were obtained, and most of the pyrazoles were synthesized smoothly and efficiently under mild conditions. Additionally, a comparison of the reactivity for other arylazoles was conducted. This provides a new and alternative method for the construction of diverse and potentially bioactive N-fused heterocycles for drug candidate screening.
Acknowledgements
This work was supported by the National Natural Science Foundation of China (21402126), Natural Science Foundation of Liaoning Province (20141090), Program for Excellent Talents in University of Liaoning Province (LJQ2014121), and Doctoral Fund of Shenyang Normal University.
Notes and references
-
(a) E. Vitaku, D. T. Smith and J. T. Njardarson, J. Med. Chem., 2014, 57, 10257 CrossRef CAS;
(b) L. M. De Coen, T. S. A. Heugebaert, D. García and C. V. Stevens, Chem. Rev., 2016, 116, 80 CrossRef CAS PubMed.
-
(a) T. Itoh, Chem. Rev., 2012, 112, 4541 CrossRef CAS;
(b) N. A. Romero and D. A. Nicewicz, Chem. Rev., 2016, 116, 10075 CrossRef CAS.
-
(a) N. R. Candeias, L. C. Branco, P. M. P. Gois, C. A. M. Afonso and A. F. Trindade, Chem. Rev., 2009, 109, 2703 CrossRef CAS;
(b) N. M. Evdokimov, S. Van slambrouck, P. Heffeter, L. Tu, B. Le Calvé, D. Lamoral-Theys, C. J. Hooten, P. Y. Uglinskii, S. Rogelj, R. Kiss, W. F. A. Steelant, W. Berger, J. J. Yang, C. G. Bologa, A. Kornienko and I. V. Magedov, J. Med. Chem., 2011, 54, 2012 CrossRef CAS;
(c) A. T. Baviskar, C. Madaan, R. Preet, P. Mohapatra, V. Jain, A. Agarwal, S. K. Guchhait, C. N. Kundu, U. C. Banerjee and P. V. Bharatam, J. Med. Chem., 2011, 54, 5013 CrossRef CAS;
(d) W. Wei, J. Wen, D. Yang, J. Du, J. You and H. Wang, Green Chem., 2014, 16, 2988 RSC.
-
(a) G. Dyker, W. Stirner and G. Henkel, Eur. J. Org. Chem., 2000, 1433 CrossRef CAS;
(b) N. Okamoto, K. Sakurai, M. Ishikura, K. Takeda and R. Yanada, Tetrahedron Lett., 2009, 50, 4167 CrossRef CAS.
-
(a) I. Nakamura and Y. Yamamoto, Chem. Rev., 2004, 104, 2127 CrossRef CAS PubMed;
(b) N. T. Patil and Y. Yamamoto, Chem. Rev., 2008, 108, 3395 CrossRef CAS;
(c) M. Álvarez-Corral, M. Muñoz-Dorado and I. Rodríguez-García, Chem. Rev., 2008, 108, 3174 CrossRef;
(d) I. V. Seregin, A. W. Schammel and V. Gevorgyan, Tetrahedron, 2008, 64, 6876 CrossRef CAS;
(e) X.-F. Wu, H. Neumann and M. Beller, Chem. Rev., 2013, 113, 1 CrossRef CAS;
(f) C. Chen, G. Shang, J. Zhou, Y. Yu, B. Li and J. Peng, Org. Lett., 2014, 16, 1872 CrossRef CAS;
(g) X. Fan, M. Yan, Y. Wang and X. Zhang, J. Org. Chem., 2015, 80, 10536 CrossRef CAS.
-
(a) J. Lu and H. Fu, J. Org. Chem., 2011, 76, 4600 CrossRef CAS PubMed;
(b) R. Xie, H. Fu and Y. Ling, Chem. Commun., 2011, 47, 8976 RSC;
(c) L. Yan, D. Zhao, J. Lan, Y. Cheng, Q. Guo, X. Li, N. Wu and J. You, Org. Biomol. Chem., 2013, 11, 7966 RSC;
(d) T. Liu and H. Fu, Synthesis, 2012, 44, 2805 CrossRef CAS;
(e) J. Peng, G. Shang, C. Chen, Z. Miao and B. Li, J. Org. Chem., 2013, 78, 1242 CrossRef CAS;
(f) L.-R. Wen, X.-J. Jin, X.-D. Niu and M. Li, J. Org. Chem., 2015, 80, 90 CrossRef CAS;
(g) P. Ruiz-Castillo and S. L. Buchwald, Chem. Rev., 2016, 116, 12564 CrossRef CAS.
-
(a) G. Meng, H.-Y. Niu, G.-R. Qu, J. S. Fossey, J.-P. Li and H.-M. Guo, Chem. Commun., 2012, 48, 9601 RSC;
(b) S. J. Hwang, S. H. Cho and S. Chang, J. Am. Chem. Soc., 2008, 130, 16158 CrossRef CAS;
(c) X. Li and M. Zhao, J. Org. Chem., 2011, 76, 8530 CrossRef CAS;
(d) G. Zhao, C. Chen, Y. Yue, Y. Yu and J. Peng, J. Org. Chem., 2015, 80, 2827 CrossRef CAS;
(e) N. Umeda, H. Tsurugi, T. Satoh and M. Miura, Angew. Chem., Int. Ed., 2008, 47, 4019 CrossRef CAS;
(f) A. G. Algarra, W. B. Cross, D. L. Davies, Q. Khamker, S. A. Macgregor, C. L. McMullin and K. Singh, J. Org. Chem., 2014, 79, 1954 CrossRef CAS;
(g) X. Li and M. Zhao, J. Org. Chem., 2011, 76, 8530 CrossRef CAS;
(h) R. Wang and J. R. Falck, J. Organomet. Chem., 2014, 759, 33 CrossRef CAS;
(i) S. Karthik, J. Ajantha, C. M. Nagaraja, S. Easwaramoorthi and T. Gandhi, Org. Biomol. Chem., 2016, 14, 10255 RSC;
(j) H. Ikemoto, T. Yoshino, K. Sakata, S. Matsunaga and M. Kanai, J. Am. Chem. Soc., 2014, 136, 5424 CrossRef CAS;
(k) M. Choi, J. Park, N. K. Mishra, S.-Y. Lee, J. H. Kim, K. M. Jeong, J. Lee, Y. H. Jung and I. S. Kim, Tetrahedron Lett., 2015, 56, 4678 CrossRef CAS;
(l) B. Li, B. Zhang, X. Zhang and X. Fan, Chem. Commun., 2017, 53, 1297 RSC;
(m) S.-S. Li, Y.-Q. Xia, F.-Z. Hu, C.-F. Liu, F. Su and L. Dong, Chem.–Asian J., 2016, 11, 3165 CrossRef CAS;
(n) I. E. Iagafarova, D. V. Vorobyeva, A. S. Peregudov and S. N. Osipov, Eur. J. Org. Chem., 2015, 4950 CrossRef CAS.
-
(a) H. M. L. Davies and J. R. Manning, Nature, 2008, 451, 417 CrossRef CAS;
(b) Y. Xia, Y. Zhang and J. Wang, ACS Catal., 2013, 3, 2586 CrossRef CAS.
- A. Ford, H. Miel, A. Ring, C. N. Slattery, A. R. Maguire and M. A. McKervey, Chem. Rev., 2015, 115, 9981 CrossRef CAS.
- W.-W. Chan, S.-F. Lo, Z. Zhou and W.-Y. Yu, J. Am. Chem. Soc., 2012, 134, 13565 CrossRef CAS.
- Y. Li, Z. Qi, H. Wang, X. Yang and X. Li, Angew. Chem., Int. Ed., 2016, 55, 11877 CrossRef CAS.
- D. Zhao, J. H. Kim, L. Stegemann, C. A. Strassert and F. Glorius, Angew. Chem., Int. Ed., 2015, 54, 4508 CrossRef CAS.
-
(a) S.-G. Lim, J. H. Lee, C. W. Moon, J.-B. Hong and C.-H. Jun, Org. Lett., 2003, 5, 2759 CrossRef CAS;
(b) Z.-M. Sun, S.-P. Chen and P. Zhao, Chem.–Eur. J., 2010, 16, 2619 CrossRef CAS;
(c) P. Zhao, F. Wang, K. Han and X. Li, Org. Lett., 2012, 14, 3400 CrossRef CAS;
(d) Z. Qi, S. Yu and X. Li, Org. Lett., 2016, 18, 700 CrossRef CAS;
(e) H. Wang, L. Li, S. Yu, Y. Li and X. Li, Org. Lett., 2016, 18, 2914 CrossRef CAS;
(f) M. Choi, J. Park, N. K. Mishra, S.-Y. Lee, J. H. Kim, K. M. Jeong, J. Lee, Y. H. Jung and I. S. Kim, Tetrahedron Lett., 2015, 56, 4678 CrossRef CAS;
(g) J. H. Kim, S. Greßies and F. Glorius, Angew. Chem., Int. Ed., 2016, 55, 5577 CrossRef CAS;
(h) J. Wang, S. Zha, K. Chen, F. Zhang and J. Zhu, Org. Biomol. Chem., 2016, 14, 4848 RSC;
(i) X. G. Li, M. Sun, Q. Jin, K. Liu and P. N. Liu, J. Org. Chem., 2016, 81, 3901 CrossRef CAS;
(j) Y. Cheng and C. Bolm, Angew. Chem., Int. Ed., 2015, 54, 12349 CrossRef CAS.
-
(a) K. Parthasarathy and C.-H. Cheng, J. Org. Chem., 2009, 74, 9359 CrossRef CAS;
(b) P. C. Too, S. H. Chua, S. H. Wong and S. Chiba, J. Org. Chem., 2011, 76, 6159 CrossRef CAS;
(c) T. K. Hyster and T. Rovis, Chem. Commun., 2011, 47, 11846 RSC;
(d) Z. Shi, D. C. Koester, M. Boultadakis-Arapinis and F. Glorius, J. Am. Chem. Soc., 2013, 135, 12204 CrossRef CAS;
(e) B. Li, P. Jiao, H. Zhong and J. Huang, Synlett, 2013, 24, 2431 CrossRef CAS;
(f) R. S. Phatake, P. Patel and C. V. Ramana, Org. Lett., 2016, 18, 292 CrossRef CAS.
-
(a) N. K. Mishra, M. Choi, H. Jo, Y. Oh, S. Sharma, S. H. Han, T. Jeong, S. Han, S.-Y. Lee and I. S. Kim, Chem. Commun., 2015, 51, 17229 RSC;
(b) H. Chu, P. Xue, J.-T. Yu and J. Cheng, J. Org. Chem., 2016, 81, 8009 CrossRef CAS.
-
(a) J. Wang, M. Wang, K. Chen, S. Zha, C. Song and J. Zhu, Org. Lett., 2016, 18, 1178 CrossRef CAS;
(b) C. Song, C. Yang, F. Zhang, J. Wang and J. Zhu, Org. Lett., 2016, 18, 4510 CrossRef CAS.
- S. Gupta, J. Han, Y. Kim, S. W. Lee, Y. H. Rhee and J. Park, J. Org. Chem., 2014, 79, 9094 CrossRef CAS.
- J.-Y. Son, S. Kim, W. H. Jeon and P. H. Lee, Org. Lett., 2015, 17, 2518 CrossRef CAS.
-
(a) G. Song, D. Chen, C.-L. Pan, R. H. Crabtree and X. Li, J. Org. Chem., 2010, 75, 7487 CrossRef CAS;
(b) M. Presset, D. Oehlrich, F. Rombouts and G. A. Molander, Org. Lett., 2013, 15, 1528 CrossRef CAS;
(c) J. Shi, J. Zhou, Y. Yan, J. Jia, X. Liu, H. Song, H. E. Xu and W. Yi, Chem. Commun., 2015, 51, 668 RSC;
(d) L. Shi, K. Yu and B. Wang, Chem. Commun., 2015, 51, 17277 RSC;
(e) Y. Wu, P. Sun, K. Zhang, T. Yang, H. Yao and A. Lin, J. Org. Chem., 2016, 81, 2166 CrossRef CAS.
-
(a) X. Yang, Y. Jin, H. Liu, Y. Jiang and H. Fu, RSC Adv., 2012, 2, 11061 RSC;
(b) X. Yang, Y. Luo, Y. Jin, H. Liu, Y. Jiang and H. Fu, RSC Adv., 2012, 2, 8258 RSC;
(c) X. Yang, J. Jie, H. Li and M. Piao, RSC Adv., 2016, 6, 57371 RSC;
(d) J. Jie, H. Li, M. Piao and X. Yang, Heterocycles, 2016, 92, 1215 CrossRef CAS;
(e) H. Li, J. Jie, S. Wu, X. Yang and H. Xu, Org. Chem. Front., 2017, 4, 250 RSC.
- S. Qu and C. J. Cramer, J. Org. Chem., 2017, 82, 1195 CrossRef CAS.
Footnotes |
† Electronic supplementary information (ESI) available. See DOI: 10.1039/c7ra03262e |
‡ These two authors contributed equally to this work. |
|
This journal is © The Royal Society of Chemistry 2017 |
Click here to see how this site uses Cookies. View our privacy policy here.