DOI:
10.1039/C7RA04889K
(Paper)
RSC Adv., 2017,
7, 30554-30558
Rhodium(III)-catalyzed indole-directed carbenoid aryl C–H insertion/cyclization: access to 1,2-benzocarbazoles†
Received
1st May 2017
, Accepted 8th June 2017
First published on 13th June 2017
Abstract
A rhodium(III)-catalyzed indole-directed aryl C–H bond carbenoid insertion cascade of 2-arylindoles with diazo compounds has been developed. This method provides a rapid access to 1,2-benzocarbazoles and isoquinoline-based polycyclic heteroaromatics with a broad range of functional group tolerance. The primary evaluation of the photoluminescence property of the novel extended π-systems indicated that these heteroarenes could be potentially used in the field of optoelectronic materials.
Introduction
Fused polycyclic heteroaromatics (FPHs) have been referred to as privileged structures in complex natural products, pharmaceuticals and organic functional materials.1 Particularly, N-fused polycycles have attracted increasing interest for their diverse photophysical properties.2 For example, carbazole-based FPHs (A, Fig. 1) could be employed to furnish green phosphorescent organic light-emitting diodes (OLED).3 Pyran-based FPHs (B, Fig. 1)-containing molecular devices4 exhibited red emission color. Moreover, spiro-annulated triarylamine-based polycyclic heteroarenes (C, Fig. 1)5 could be used to assemble deep-blue OLED devices because these spiro molecules can provide exciton blocking and hole-injection properties due to their deep HOMO and high triplet energy level. Thus it can be seen that different core subunits generally exhibited particular optical and electroluminescent properties. However, in the past decades, although many structural skeletons have been well evaluated for their optoelectronic applications,6 1,2-benzocarbazoles, especially for isoquinolinocarbazole derivative (D, Fig. 1) which possess excellent conformational rigidity property from the molecular backbone, have rarely been reported owing to the restriction of synthetic access to these compounds (D, Fig. 1).7
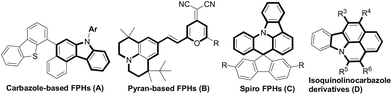 |
| Fig. 1 Selected examples of N-fused polycycles. | |
Recently, the 1,2-benzocarbazoles could be assembled relying on the construction of ring A and ring B through cascade cyclic strategies of alkynes8 (Scheme 1a) and others,9 but most of these methods suffer from the disadvantage of requiring prefunctionalized alkynes or tedious steps. Therefore, developing more efficient method to construct 1,2-benzocarbazoles utilizing easily materials remains highly desirable and challenging. On the other hand, transition metal-catalyzed C–H carbenoid functionalization provides an atom- and step-economic approach to construct C–C bonds.10 Moreover, the reactivity of acceptor moieties of carbenoids could also be fully utilized as an internal nucleophilic partner.11 From the step economy as well as the functional group diversity point of view, cascade C–H carbenoid functionalization belongs to an innovative approach to complex heterocycles. For examples, we recently reported a Rh(III)-catalyzed relay Csp2–H bond carbenoid functionalization of N-aryl ketoimines, in which a nucleophilic enamine derived from imine/enamine-isomerization was trapped by an acceptor ketocarbonyl moiety of diazo compounds to assemble 1-azaphenalenes (1-APLEs). These 1-APLEs showed promising potential in optoelectronic applications due to their low-lying HOMO levels (Scheme 1b). 12 Encouraged by this work and in combination with our interest in developing cascade C–H carbenoid functionalization toward photoelectrically interesting π-conjugated molecule synthesis, we proposed that a 2-arylindole molecule which contains a nucleophilic directing indole group, could also possibly undergo relay carbenoid aryl C–H insertion/cyclization to furnish polycyclic heteroarenes through constructing ring B even ring C13 (Scheme 1c).
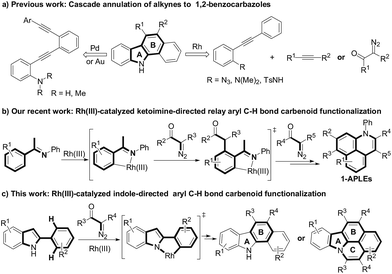 |
| Scheme 1 Aryl Csp2–H carbenoid functionalization strategies for assembling FPHs. | |
Results and discussion
To achieve this reaction, we commenced our study with 2-phenylindole (1a) and 2-diazo-3-oxobutanoic ethyl ester (2a) as a model reaction (Table 1). The effect of base on the cascade aryl C–H bond carbenoid insertion was first evaluated using [Cp*RhCl2]2 (5 mol%) as a catalyst in combination with cocatalyst Cu(OAc)2 (50 mol%) and additive KOAc (2.0 equiv.) in CH3CN under an Ar atmosphere at 80 °C for 8 h (entries 1–4), and we quickly found that this catalytic system produced the major 5-ethoxycarbonyl-6-methyl-1,2-benzocarbazole 3a under basic condition.14 Among them, Na2CO3 provided 3a (62% yield) and isoquinoline-based carbazole 4a (13% yield) (entry 2). Although the competitive aryl C–H carbenoid insertion led to poor chemoselectivity under Rh(III)/Cu(II) system, this positive result still encouraged us to conduct further optimization of the reaction conditions for achieving excellent selectivity. Low oxidation state copper(I) salt chemoselectively afforded 3a in 84% yield under NaOAc/Na2CO3 system, and no 4a was observed (compare entries 5–9 with 10). Interestingly, switching copper(I) cocatalysts into silver salts resulted in the formation of the major product 4a when employing 2,2,2-trifluoroethanol (TFE) as solvent in the absence of Na2CO3 (entries 11–13). In particular, Rh(III)/AgOAc/PivOH system significantly increased the chemoselectivity of this transformation at 120 °C and provided 45% yield of 4a (compare entries 11–13 with 14). When other alcohols such as i-PrOH were employed as solvents, no desired 3a and 4a were observed (entry 15).
Table 1 Optimization of the reaction parametersa

|
Entry |
Cocatalyst |
Additive |
Base |
Solvent |
Yield (%)b |
3a/4a |
Unless otherwise noted, all the reactions were carried out using 2-phenylindole (1a) (0.20 mmol) and diazo compound (2a) (0.40 mmol) with [Cp*RhCl2]2 catalysts (0.01 mmol, 5 mol%) in the presence of cocatalyst (copper salts: 0.10 mmol, 50 mol%; silver salts: 0.06 mmol, 30 mol%), base (0.40 mmol, 2.0 equiv.) and additives (0.06 mmol, 30 mol%) in solvent (2.0 mL) at 80 °C for 8 h under Ar in a sealed reaction tube. Followed by flash chromatography on SiO2. Isolated yield. The reaction temperature was 120 °C; 0.80 mmol of diazo compound 2a was used. |
1 |
Cu(OAc)2 |
KOAc |
— |
CH3CN |
0/0 |
2 |
Cu(OAc)2 |
KOAc |
Na2CO3 |
CH3CN |
62/13 |
3 |
Cu(OAc)2 |
KOAc |
NaHCO3 |
CH3CN |
53/19 |
4 |
Cu(OAc)2 |
KOAc |
KHCO3 |
CH3CN |
48/28 |
5 |
CuOAc |
KOAc |
Na2CO3 |
CH3CN |
61/7 |
6 |
CuBr |
KOAc |
Na2CO3 |
CH3CN |
68/5 |
7 |
CuI |
KOAc |
Na2CO3 |
CH3CN |
48/0 |
8 |
CuCN |
KOAc |
Na2CO3 |
CH3CN |
67/20 |
9 |
CuCl |
CsOAc |
Na2CO3 |
CH3CN |
58/18 |
10 |
CuCl |
NaOAc |
Na2CO3 |
CH3CN |
84/0 |
11 |
Ag(NTf)2 |
AcOH |
— |
TFE |
31/41c |
12 |
AgOAc |
AcOH |
— |
TFE |
28/39c |
13 |
AgOAc |
PhCO2H |
— |
TFE |
29/35c |
14 |
AgOAc |
PivOH |
— |
TFE |
8/45c |
15 |
AgOAc |
PivOH |
— |
i-PrOH |
0/0c |
With the optimized reaction conditions in hand, the scope of 2-arylindoles was then examined by employing 2-diazo-3-oxobutanoic ethyl ester (2a) as the coupling partner. As shown in Scheme 2, a variety of 2-arylindoles were smoothly converted to the corresponding multisubstituted 1,2-benzocarbazole derivatives in good to excellent yields. The substituents on the indole ring and the 2-indoly phenyl ring apparently exerts different electronic effects on the reactivity (3a–3w). For example, electron-rich substituents (Me, OMe) provided the corresponding 1,2-benzocarbazoles in 61–82% yields (3b, 3c, 3h, 3l, 3n and 3t). On the contrary, electron-poor substituent such as CO2Et, CN, NO2 and CF3 etc. showed poorer reactivity and afforded the target compounds in 35–58% yields (3f, 3g, 3j, 3r and 3s). It is worth noting that the reaction is sensitive to the substituent position in the indole rings. 5- or 6-Substituted 2-phenylindoles showed good reactivity (3b–3j), but 7-methyl 2-phenylindole did not furnish the desired carbazole, possibly due to steric factors (3k).
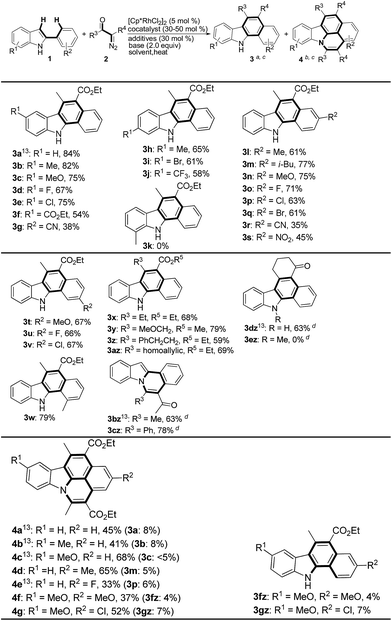 |
| Scheme 2 Substrate scope. a For the synthetic procedure of product 3: all the reactions were carried out using 2-arylindoles (1) (0.20 mmol) and diazo compounds (2) (0.40 mmol) with [Cp*RhCl2]2 (5 mol%) in the presence of CuCl (50 mol%), NaOAc (30 mol%) and Na2CO3 (2.0 equiv.) in acetonitrile (2.0 mL) at 80 °C for 8 h under Ar in a sealed reaction tube. b For the synthetic procedure of product 4: all the reactions were carried out using 1 (0.20 mmol) and 2 (0.80 mmol) with [Cp*RhCl2]2 (5 mol%) in the presence of AgOAc (30 mol%), PivOH (2.0 equiv.) in CF3CH2OH (2.0 mL) at 120 °C for 24 h under Ar in a sealed reaction tube. c Isolated yield. d Cu(OAc)2 (50 mol%) was used. | |
Moreover, the present cascade aryl C–H carbenoid insertion protocol was subsequently applied to a wide range of α-acyldiazo compounds with particular 2-phenylindole (1a) as the coupling partner. As anticipated, α-alkylacyl, α-alkoxymethylacyl and α-homoallylic acyl substituted diazoacetates could easily enable assembly of 5-ethoxycarbonyl-1,2-benzocarbazoles in 59–79% yields (3x–3az). Meanwhile, linear α-acyl diazoketones produced the corresponding 1-indolo[2,1-a]isoquinolin-5-yl-ethanones 3bz and 3cz in 63% and 78% yields, respectively, but cyclic α-acyl diazoketones produced the desired 11H-benzo[a]carbazole 3dz in 63% yield. If the indole nitrogen atom was blocked with a methyl group, this transformation did not occur (3ez).
Next, Rh(III)/Ag(I) system catalyzed double aryl C–H carbenoid insertion cyclization between 2-arylindoles and 2-diazo-3-oxobutanoic ethyl ester (2a) was further investigated. As shown in Scheme 2 (4a–4g), various methyl, alkoxy and halo-substituted 2-aryl-indoles could be smoothly employed to furnish isoquinolinocarbazoles (4a–4g) in acceptable yields (33–56%).12 It should be noted that around 5% yields of 1,2-benzocarbazoles (3a–3c, 3m, 3p, 3fz and 3gz) were observed.
Finally, the photo-physical properties, quantum yields and fluorescence lifetime spectrometer of partial molecules were explored systematically. The data of absorption and photoluminescence wavelength, the molar absorption coefficients (ε) and the excited state lifetimes (τ) as well as quantum yields (Φ) of the selected compounds 3a, 4a, 4c, 4f, 4g and 5a15 in CH2Cl2 are listed in Table S-1.† As shown in Fig. 2, the emission spectra of 3a and 5a showed a peak at 374 nm and 386 nm, respectively. In contrast, the other compounds exhibited obvious bathochromic shifts (521–539 nm) in emission.16
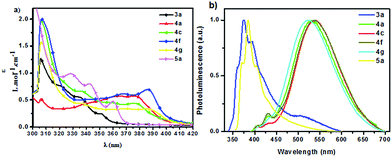 |
| Fig. 2 UV-vis absorption (a) and emission spectra (b) of the compounds 3a, 4a, 4c, 4f, 4g and 5a in dichloromethane solutions(10−5 M). | |
Their emission colour change in dichloromethane (10−5 M) under UV excitation (365 nm) further indicated that 3a and 5a showed blue light, while the others exhibited yellow light under UV excitation (Fig. 3). These results demonstrated molecules 4a, 4c, 4f and 4g were potential for furnishing white OLED devices.17
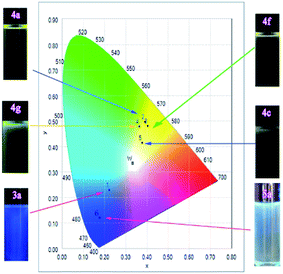 |
| Fig. 3 Emission color change of 3a, 4a, 4c, 4f, 4g, and 5a in dichloromethane (10−5 M) upon excitation at 365 nm. | |
Several control experiments were performed to further explore the possible mechanism (Scheme 3). First, the H/D exchange of 2-phenylindole 1a was conducted in a Rh(III)/Cu(I)/CH3OD or Rh(III)/Ag(I)/AcOD system in the absence of diazo compounds, and 36% and 13% deuterium incorporation at the 2-indolylphenyl ring C2- and C6-position was observed, respectively (eqn (1) and (2)). And also, the H/D exchange of C3-position from indole ring occurred possibly due to that indole C3-position possesses strong nucleophilic properties and easily attacks rhodium cation followed by metal protonation. On the contrary, no H/D exchange of N-methyl-2-phenylindole 1y was observed under the same conditions (eqn (3)), this fact implied that the indole NH moiety played a key role of chelation in promoting 2-indolylphenyl ring C–H bond cleavage. Subsequently, treating 1,2-benzocarbazole 3a with diazo compound 2a under a Rh(III)Ag(I)/PivOH system did not furnish the corresponding isoquinoline-based carbazole 4a. This control experiment also demonstrated that 1,2-benzocarbazole 3a was not the intermediate to form product 4a (eqn (4)). Finally, the competitive isotope effect (kH/kD = 2.7) further indicated that 2-indolylphenyl C–H bond-breaking was possibly involved in the rate-limiting step of 1,2-benzocarbazole-forming reaction (eqn (5)) (see ESI† for more details).
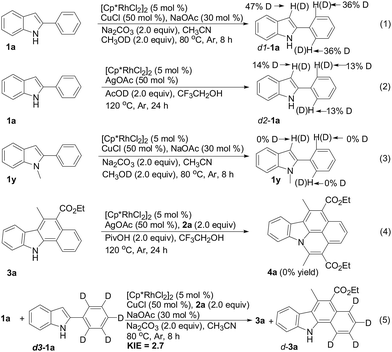 |
| Scheme 3 Preliminary mechanistic studies. | |
A proposed catalytic cycle is shown in Scheme 4. The initial N-coordination of 2-phenylindole 1a to Rh(III) catalyst followed by 2-indolylphenyl C–H bond activation afforded rhodacycle intermediate A to generate Rh-carbene species B, which would undergo migratory insertion and protonolysis to form rhodacycle intermediate C and complex D, respectively.18,19 Subsequently, Rh(III)/Cu(I) system catalyzed cyclization of D to furnish 1,2-benzocarbazole 3a, in which Cu(I) salts played a role of Lewis acid to activate keto-carbonyl group of F. Meanwhile, complex D could also further undergo the secondary indole-directed phenyl C–H carbenoid insertion/cyclization cascade under Rh(III)/Ag catalytic system to produce isoquinoline-based carbazole 4a.
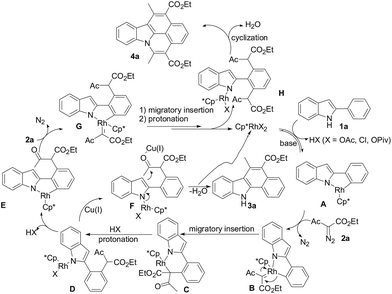 |
| Scheme 4 Proposed mechanism. | |
Conclusions
In summary, we have developed a Rh(III)-catalyzed, indole-directed phenyl C–H bond carbenoid insertion/cyclization of 2-phenylindoles20 with alpha-acyl diazocompounds21 for rapid assembly of 1,2-benzocarbazoles and isoquinoline-based carbazoles. Further exploring the photoelectronic performance of isoquinoline-based carbazoles demonstrated that these compounds could be further assembled within promising optoelectronic devices.
Acknowledgements
The authors thank the National Key Research and Development Program of China (No. 2016YFA0602900), the NSFC (No. 21372085), and the Science and Technology Program of Guangzhou (No. 156300075) for financial support.
Notes and references
- For selected reviews, see:
(a) W. Jiang, Y. Li and Z. Wang, Chem. Soc. Rev., 2013, 42, 6113 RSC;
(b) C. Beemelmanns and H. U. Reissig, Chem. Soc. Rev., 2011, 40, 2199 RSC and reference therein.
- For selected examples, see:
(a) Z. Q. Jiang, Z. Y. Liu, C. L. Yang, C. Zhong, J. G. Qin, G. Yu and Y. Q. Liu, Adv. Funct. Mater., 2009, 19, 3987 CrossRef CAS;
(b) M. S. Park and J. Y. Lee, Chem. Mater., 2011, 23, 4338 CrossRef CAS;
(c) Z. Q. Jiang, Y. H. Chen, C. Fan, C. L. Yang, Q. Wang, Y. T. Tao, Z. Q. Zhang, J. G. Qin and D. G. Ma, Chem. Commun., 2009, 45, 3398 RSC;
(d) T. Mitsumori, M. Bendikov, O. Dautel, F. Wudl, T. Shioya, H. Sato and Y. Sato, J. Am. Chem. Soc., 2004, 126, 16793 CrossRef CAS PubMed.
- Y. Chen, W. Liang, W. H. Choi, J. Huang, Q. Dong, F. Zhua and J. Su, Dyes Pigm., 2015, 123, 196 CrossRef CAS.
- K. H. Lee, M. H. Park, C. S. Kim, Y. K. Kim and S. S. Yoon, Thin Solid Films, 2011, 520, 510 CrossRef CAS.
- M. Kim and J. Y. Lee, Org. Electron., 2012, 13, 1245 CrossRef CAS.
- For selected examples, see:
(a) X. Qin, X. Li, Q. Huang, H. Liu, D. Wu, Q. Guo, J. Lan, R. Wang and J. You, Angew. Chem., Int. Ed., 2015, 54, 7167 CrossRef CAS PubMed;
(b) T. H. Huang, W. T. Whang, J. Y. Shen, Y. S. Wen, J. T. Lin, T. H. Ke, L. Y. Chen and C. C. Wu, Adv. Funct. Mater., 2006, 16, 1449 CrossRef CAS;
(c) E. Ahmed, T. Earmme, G. Q. Ren and S. A. Jenekhe, Chem. Mater., 2010, 22, 5786 CrossRef CAS;
(d) T. Spehr, R. Pudzich, T. Fuhrmann and J. Salbeck, Org. Electron., 2003, 4, 61 CrossRef CAS;
(e) K. R. J. Thomas, J. T. Lin, Y. T. Tao and C. H. Chuen, Adv. Mater., 2002, 14, 822 CrossRef CAS.
-
(a) S. Ito, Y. Tokimaru and K. Nozaki, Angew. Chem., Int. Ed., 2015, 54, 7256 CrossRef CAS PubMed;
(b) Z. Shi, S. Ding, Y. Cui and N. Jiao, Angew. Chem., Int. Ed., 2009, 48, 7895 CrossRef CAS PubMed;
(c) R. Xie, Y. Ling and H. Fu, Chem. Commun., 2012, 48, 12210 RSC.
-
(a) X. F. Xia, N. Wang, L. L. Zhang, X. R. Song, X. Y. Liu and Y. M. Liang, J. Org. Chem., 2012, 77, 9163 CrossRef CAS PubMed;
(b) N. Li, X. L. Lian, Y. H. Li, T. Y. Wang, Z. Y. Han, L. Zhang and L. Z. Gong, Org. Lett., 2016, 18, 4178 CrossRef CAS PubMed.
- For selected examples, see:
(a) S. Protti, A. Palmieri, M. Petrini, M. Fagnoni, R. Ballini and A. Albini, Adv. Synth. Catal., 2013, 355, 643 CrossRef CAS;
(b) F. Sha, Y. Tao, C. Y. Tang, F. Zhang and X. Y. Wu, J. Org. Chem., 2015, 80, 8122 CrossRef CAS PubMed;
(c) N. J. Truax, F. B. Mejia, D. O. Kwansare, M. M. Lafferty, M. H. Kean and E. T. Pelkey, J. Org. Chem., 2016, 81, 6808 CrossRef CAS PubMed.
- For selected examples, see:
(a) A. Gutierrez-Bonet, F. Julia-Hernandez, B. de Luis and R. Martin, J. Am. Chem. Soc., 2016, 138, 6384 CrossRef CAS PubMed;
(b) R. S. Phatake, P. Patel and C. V. Ramana, Org. Lett., 2016, 18, 2828 CrossRef CAS PubMed;
(c) W. W. Chan, S. F. Lo, Z. Zhou and W. Y. Yu, J. Am. Chem. Soc., 2012, 134, 13565 CrossRef CAS PubMed;
(d) K. Shibata and N. Chatani, Chem. Sci., 2016, 7, 240 RSC;
(e) S. Yu, S. Liu, Y. Lan, B. Wan and X. Li, J. Am. Chem. Soc., 2015, 137, 1623 CrossRef CAS PubMed;
(f) Y. Xia, Z. Liu, Z. Liu, R. Ge, F. Ye, M. Hossain, Y. Zhang and J. Wang, J. Am. Chem. Soc., 2014, 136, 3013 CrossRef CAS PubMed;
(g) Z. Shi, D. C. Koester, M. Boultadakis-Arapinis and F. Glorious, J. Am. Chem. Soc., 2013, 135, 12204 CrossRef CAS PubMed;
(h) T. K. Hyster, K. E. Ruhl and T. Rovis, J. Am. Chem. Soc., 2013, 135, 5364 CrossRef CAS PubMed.
- For selected examples, see:
(a) D. Zhao, J. H. Kim, L. Stegemann, C. A. Strassert and F. Glorius, Angew. Chem., Int. Ed., 2015, 54, 4508 CrossRef CAS PubMed;
(b) Y. Xia, Z. X. Liu, Z. Liu, R. Ge, F. Ye, M. Hossain, Y. Zhang and J. B. Wang, J. Am. Chem. Soc., 2014, 136, 3013 CrossRef CAS PubMed;
(c) Z. Shi, D. C. Koester, M. Boultadakis-Arapinis and F. Glorius, J. Am. Chem. Soc., 2013, 135, 12204 CrossRef CAS PubMed;
(d) X. Chen, X. Hu, Y. Deng, H. F. Jiang and W. Zeng, Org. Lett., 2016, 18, 4742 CrossRef CAS PubMed;
(e) J. Wang, M. Wang, K. Chen, S. Zha, C. Song and J. Zhu, Org. Lett., 2016, 18, 1178 CrossRef CAS PubMed.
- Y. Xie, X. Chen, X. Liu, S. J. Su, J. Li and W. Zeng, Chem. Commun., 2016, 52, 5856 RSC.
- Unfortunately, during the period that we carried out this work, two similar works have been reported through constructing ring B and ring C of isoquinolinocarbazole derivatives through Ir(III) and Rh(III) catalytical system, see:
(a) S. S. Li, Y. Q. Xia, F. Z. Hu, C. F. Liu, F. Su and L. Dong, Chem.–Asian J., 2016, 11, 3165 CrossRef CAS PubMed;
(b) B. Li, B. Zhang, X. Zhang and X. Fan, Chem. Commun., 2017, 53, 1297 RSC.
- The structures of 3a (CCDC No. 1511555) and 4e (CCDC No. 1511559) were already unambiguously assigned by their crystal X-ray analysis, please see ESI† for the more details.
- We made the compound 5a through the N-methylation of 3a in order to systematically study the emission properties. For the structure of 5a, please see ESI.†.
- B. Li, J. Lan, D. Wu and J. You, Angew. Chem., Int. Ed., 2015, 54, 14008 CrossRef CAS PubMed.
- H. H. Chou and C. H. Cheng, Adv. Mater., 2010, 22, 2468 CrossRef CAS PubMed.
- K. Morimoto, K. Hirano, T. Satoh and M. Miura, Org. Lett., 2010, 12, 2068 CrossRef CAS PubMed.
-
(a) W. Chan, S. F. Lo, Z. Zhou and W. Y. Yu, J. Am. Chem. Soc., 2012, 134, 13565 CrossRef CAS PubMed;
(b) S. Yu, S. Liu, Y. Lan, B. Wan and X. W. Li, J. Am. Chem. Soc., 2015, 137, 1623 CrossRef CAS PubMed.
-
(a) Y. Wei, I. Deb and N. Yoshikai, J. Am. Chem. Soc., 2012, 134, 9098 CrossRef CAS PubMed;
(b) S. Gore, S. Baskaran and B. König, Org. Lett., 2012, 14, 4568 CrossRef CAS PubMed;
(c) S.-D. Yang, C.-L. Sun, Z. Fang, B.-J. Li, Y.-Z. Li and Z.-J. Shi, Angew. Chem., Int. Ed., 2008, 47, 1473 CrossRef CAS PubMed.
-
(a) X. Chen, Y. Xie, X. Xiao, G. Li, Y. Deng, H. Jiang and W. Zeng, Chem. Commun., 2015, 51, 15328 RSC;
(b) R. Pasceri, H. E. Bartrum, C. J. Hayes and C. J. Moody, Chem. Commun., 2012, 48, 12077 RSC.
Footnote |
† Electronic supplementary information (ESI) available. CCDC 1511555 and 1511559. For ESI and crystallographic data in CIF or other electronic format see DOI: 10.1039/c7ra04889k |
|
This journal is © The Royal Society of Chemistry 2017 |
Click here to see how this site uses Cookies. View our privacy policy here.