DOI:
10.1039/C7RA06501A
(Paper)
RSC Adv., 2017,
7, 42416-42421
A hydrogen sulfide probe activates Nrf2, inhibits cancer cell growth and induces cell apoptosis
Received
10th June 2017
, Accepted 9th August 2017
First published on 1st September 2017
Abstract
It has been demonstrated that a relatively high level of endogenous hydrogen sulfide (H2S) promotes cancer cell growth. Thus, reducing endogenous H2S levels with a small molecule that selectively targets H2S in cancer cells is an attractive strategy to treat cancer. In this study, we synthesized a 3-hydroxyflavone-based dual probe (HF-NBD) targeting H2S with favourable photostability, and found that it could also activate the nuclear factor erythroid-2-related factor 2 (Nrf2) and upregulate heme oxygenase-1 (HO-1) expression. HF-NBD inhibited the growth of A549 lung cancer cells, U87 malignant glioma cells and HeLa cervical cancer cells, and induced apoptosis in HeLa cells. Herein we provide HF-NBD, a novel Nrf2 activator that promotes HO-1 expression downstream of Nrf2. The data suggested that HF-NBD might be a potential lead compound for the development of new anti-cancer drugs.
Introduction
Cancer has become an increasingly serious problem in recent years. Especially in developing countries, cervical cancer is one of the most frequently occurring malignancies causing death in women, making it a pressing issue.1 Likewise, lung cancer is a significant public health concern worldwide,2 and treating malignant glioma in the central nervous system is one of the most formidable challenges, with high rates of recurrence and mortality.3 Although there are some effective ways to prevent and reduce the occurrence of cancer, much remains to be done, such as finding more effective therapies to treat cancer or finding new effective drugs that can inhibit the growth of cancer cells without causing damage to healthy cells.
Hydrogen sulfide (H2S), known for being a highly toxic gas with an unpleasant smell, is actually an endogenous signalling gas molecule4 that regulates a range of physiological processes via the sulfhydration of target proteins.5 Thus, H2S plays an important role in cell activation. Nowadays, owing to advances in chemistry, biology research has progressed dramatically, and fluorescent probes can help us understand the cellular-level and subcellular-level better.6 Thus, developing reaction-based biocompatible probes for the sensitive and selective fluorescence detection of H2S can help to elucidate the mechanism of the modulation of its signalling pathway, cellular function and involvement in diseases.7 It has been reported that H2S at low levels can protect mitochondria from oxidative stress damage.8 In addition, a study showed that the H2S-mediated formation of Cys151-persulfide (SSH) in the Kelch-like ECH-associated protein 1 (Keap 1) can cause Keap 1 to dissociate from Nrf2, leading to Nrf2 translocation into the nucleus, where it binds to antioxidant response elements and triggers the transcription of protective genes.9,10 Therefore, low levels of endogenous H2S can exert cytoprotective effects in healthy cells. However, the evidence shows that cystathionine b-synthase (CBS), which participates in the synthesis of endogenous H2S, is overexpressed in cancer cells, suggesting that relatively high levels of endogenous H2S can promote cancer cell growth.11–13 Thus, reducing endogenous H2S levels with small molecules that selectively target H2S in cancer cells is an attractive approach for cancer treatment.
It is known that Nrf2, which is encoded by the nuclear factor erythroid 2-like 2 (NFE2L2) gene and is expressed in several tissues, belongs to the basic-leucine zipper (bZIP) family of transcription factors.14 Cisplatin, an anticancer drug, leads to oxidative damage and death in HEp-2 cells by increasing Nrf2 and HO-1 expression, suggesting an anticancer role for Nrf2. Connecting H2S with Nrf2, H2S probes that may enhance the level of ROS and reduce endogenous H2S could act as an Nrf2 activator and contribute to cancer treatment.
In this research, we investigated the effects of a 3-hydroxyflavone-based fluorescent probe, HF-NBD, which can detect and consume endogenous H2S,15 on the activity of Nrf2. We found that HF-NBD could increase the level of ROS in a time-dependent manner, and elevate the activity of Nrf2 and HO-1 mRNA expression. HF-NBD also inhibited the growth of A549 lung cancer cells, U87 malignant glioma cells and HeLa cervical cancer cells, and promoted apoptosis of HeLa cervical cancer cells.
Results and discussions
The HF-NBD probe can detect both endogenous and exogenous H2S with favorable photostability
Although many drugs have been used in the clinical practice to treat cancer, it is still crucial to explore new efficient drugs that have specific targets. Endogenous H2S plays an important role in cancer cell proliferation and therefore, a H2S probe able to reduce endogenous H2S levels may represent a strategy to treat cancer.13 HF-NBD was synthesized and proven to be a H2S probe,15 and we further investigated it in HeLa cervical cells to detect its effects. We detected the levels of fluorescence in HeLa cervical cells after treatment with 1‰ DMSO or HF-NBD at 1, 5, and 10 μM for 0.5, 1, and 3 h, respectively (Fig. 1B). The data showed that upon treatment with HF-NBD, fluorescence increased in a dose-dependent and time-dependent manner. Based on these results, we decided to treat the cells with HF-NBD at 5 μM for 1 h for the subsequent experiments. It is well-known that cystathionine β-synthase (CBS) and cystathionine γ-lyase (CSE) can catalyse the degradation of cysteine in living cells for H2S production.16 Similarly, the inhibition assay was also conducted by inhibiting CBS and CSE.17,18 We utilized HF-NBD to detect the levels of fluorescence in HeLa cervical cells. Compared with fluorescence in the green channel without the addition of cysteine (control), the level of fluorescence with the addition of 200 μM cysteine was significantly higher. When cells were incubated with 1 mM propargylglycine (PAG) or 1 mM aminooxyacetic acid (AOAA) or PAG/AOAA, followed by incubation with the HF-NBD probe, almost no fluorescence change was observed compared to the control group (Fig. 1C). We also detected the level of fluorescence in HeLa cervical cells after treatment with 0, 0.1, 0.5, and 1 mM NaHS, followed by incubation with the HF-NBD probe and found that the relative fluorescence increased in a dose-dependent manner (Fig. 1D). The results confirmed that HF-NBD can target both endogenous and exogenous H2S in HeLa cervical cells. We further investigated 5 μM HF-NBD and incubated it with HeLa cervical cells for 1 h to detect the photo stability during 0, 60, 120, 180, and 300 s, and the data suggested that HF-NBD does exhibit favourable photostability (Fig. 1E).
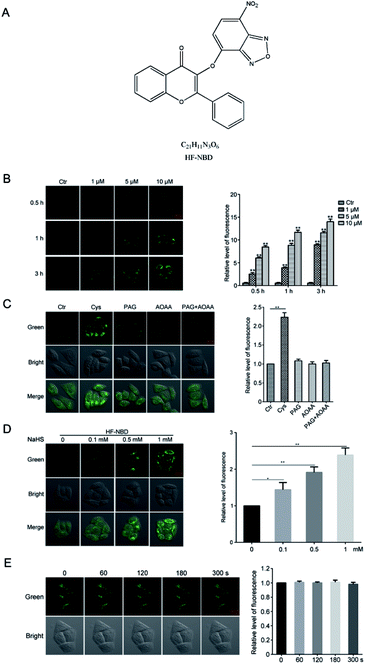 |
| Fig. 1 HF-NBD can detect both endogenous and exogenous H2S with favourable photostability. (A) Structure of HF-NBD. (B) Fluorescence in HeLa cells after treatment with 1‰ DMSO or HF-NBD at 1, 5, and 10 μM for 0.5, 1, and 3 h. (C) HeLa cells respectively treated with 200 μM cysteine, 1 mM propargylglycine (PAG), 1 mM aminooxyacetic acid (AOAA) or PAG/AOAA, fluorescence was measured after incubation with 5 μM HF-NBD for 1 h. (D) HeLa cells treated with 0, 0.1 and 0.5, and 1 mM NaHS, fluorescence was measured after incubation with HF-NB. (E) HeLa cells incubated with 5 μM HF-NBD for 1 h to evaluate photostability during 0, 60, 120, 180, 300 s. Data are presented as the mean ± SEM, *p < 0.05, **p < 0.01, n = 3. | |
HF-NBD up regulated Nrf-2 activity as well as the expression of HO-1 in HeLa cells significantly
Because Nrf2 activators have shown strong potential in disease prevention,19 we firstly investigated the effect of HF-NBD on Nrf2 activity. HeLa cells stably transfected with a luciferase-based Nrf2 reporter plasmid were treated with 10 μM HF-NBD for 12 h and 24 h (Fig. 2A). The luciferase assay showed that HF-NBD markedly increased Nrf2 activity compared with the control. Nrf2, a redox sensitive transcription factor, plays an important role as an antioxidant, protecting cells from oxidative stress.20 It has been reported that oxidative stress can induce HO-1 gene transcription in tumorous cells through activation of Nrf-2,21 where HO-1 is the downstream gene that is regulated by Nrf2 and responds to the stress. Thus, we detected the mRNA levels of HO-1 after treatment with 10 μM HF-NBD for 0.5, 1, 3, 6, 12, and 24 h (Fig. 2B). The results showed that HF-NBD could markedly upregulate the expression of HO-1 after treating with it for 12 h.
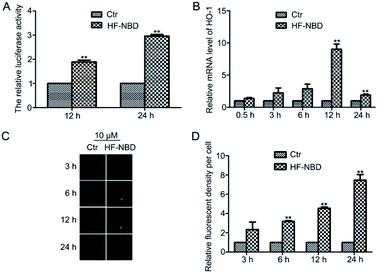 |
| Fig. 2 HF-NBD upregulates Nrf2 activity, HO-1 expression, and induces oxidative stress in HeLa cells. (A) HeLa cells stably transfected with a luciferase-based Nrf2 reporter plasmid were treated with 10 μM HF-NBD for 12 h and 24 h. (B) HO-1 mRNA levels measured after treatment with 10 μM HF-NBD for 0.5, 1, 3, 6, 12, and 24 h. (C) ROS levels measured after treatment with 10 μM HF-NBD for 3, 6, 12, and 24 h. (D) Relative ROS levels normalized to the ROS levels in the control and expressed as percent of control. Data are presented as the mean ± SEM, *p < 0.05, **p < 0.01, n = 3. | |
HF-NBD increases oxidative stress in a time-dependent manner
It has been reported that oxidative stress can alter the redox balance in tumorous microenvironments and impact metabolic pathways in cancer cells.22 Cancer cells are vulnerable to high levels of reactive oxygen species (ROS).23 The evidence indicates that ROS influences proliferation and apoptosis in various types of cancer cells.24 The overproduction of ROS results in oxidative stress and induces cell apoptosis.25 We supposed that the increased Nrf2 activity and HO-1 expression may be a response to oxidative stress caused by HF-NBD, which reduces H2S levels in cells. Thus, we detected the level of ROS after treatment with HF-NBD at 10 μM for 3, 6, 12, and 24 h (Fig. 2C). The data showed that HF-NBD increased ROS levels in a time-dependent manner (Fig. 2D), which conformed to our hypothesis that HF-NBD triggered the oxidative stress by reducing H2S levels in cells.
HF-NBD inhibits the growth of A549 lung cancer cells, U87 malignant glioma cells and HeLa cervical cancer cells with low IC50 values
We investigated the cell viability of A549 lung cancer cells (Fig. 3A), U87 malignant glioma cells (Fig. 3B) and HeLa cervical cancer cells (Fig. 3C) using sulforhodamine B (SRB) assay after treatment with HF-NBD at different concentrations for 12 h and 24 h to know if HF-NBD could affect the growth of cancer cells. We found that HF-NBD significantly inhibited the growth of these three types of cancer cells at low IC50 values (Table 1).
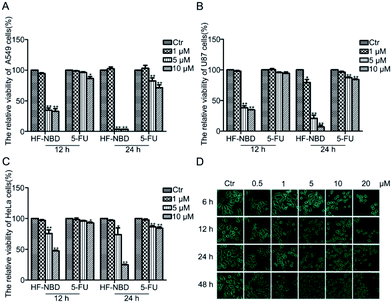 |
| Fig. 3 HF-NBD inhibits the growth of A549 lung cancer cells, U87 malignant glioma cells and HeLa cells with low IC50 values. Cell viability of A549 cells (A), U87 cells (B) and HeLa cells (C), determined by the SRB assay after treatment with 1‰ DMSO (control) and HF-NBD at 1, 5, and 10 μM for 12 h and 24 h. (D) Morphological changes in HeLa cells after treatment with HF-NBD at 0.5, 1, 5, 10, and 20 μM for 6, 12, 24 and 48 h, as observed with a phase contrast microscope. Data are presented as the mean ± SEM, *p < 0.05, **p < 0.01, n = 3. | |
Table 1 IC50 values (μM, 24 h) for HF-NBD in A549 lung cancer cells, U87 malignant glioma cells and HeLa cells
Cell type |
IC50 values |
A549 lung cancer cell |
1.77 |
U87 malignant glioma cell |
2.09 |
HeLa cervical cancer cell |
6.73 |
In order to find out how HF-NBD influenced the growth of cancer cells, we selected HeLa cells for the subsequent experiment, since the HeLa cervical cancer cell line is the most widely used cell line in biomedical research.26 We also observed the morphological changes in HeLa cells after treatment with 0.5, 1, 5, 10, and 20 μM HF-NBD for 6, 12, 24 and 48 h using a phase contrast microscope (Fig. 3D). The living cell density decreased and the cells shrank, with apoptotic bodies being released in response to treatment with HF-NBD compared with the control group.
HF-NBD did not lead HeLa cervical cancer cells to necrosis
In natural conditions, for elucidating the mechanism of toxicity of a xenobiotic, several cell death pathways need to be taken into account.27 According to a recent research, necrosis is a kind of cell programmed death.28 Thus, we tested whether HF-NBD would lead to necrosis of HeLa cervical cancer cells using an LDH assay kit, and no significant differences were found between HF-NBD-treated cells and control cells. The result showed that HF-NBD did not induce necrosis of HeLa cells at doses lower than 10 μM for 24 h (Fig. 4).
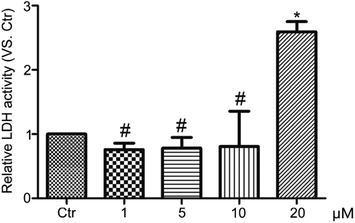 |
| Fig. 4 HF-NBD did not lead HeLa cervical cancer cells to necrosis. HeLa cells treated with 1‰ DMSO (control) or HF-NBD at 1, 5, 10, and 20 μM for 24 h. Data are presented as the mean ± SEM, *p < 0.05, **p < 0.01, n = 3. | |
HF-NBD induces apoptosis in HeLa cells
Apoptosis is one of the important programmed cellular processes that allows cells to cope with the damage induced by various stressors.29 To detect whether HF-NBD could induce apoptosis in HeLa cells, we performed a western blot experiment to measure the levels of cleaved-PARP protein. The data showed that the protein level of cleaved-PARP (89 kDa) increased after treatment with 1‰ DMSO (as control group) and HF-NBD at 1, 5, 10 μM for 24 h (Fig. 5A) and with 1‰ DMSO (as control group) for 12 h and HF-NBD at 5 μM for 6, 12, 24 h (Fig. 5B). These results demonstrated that HF-NBD could induce apoptosis in HeLa cells.
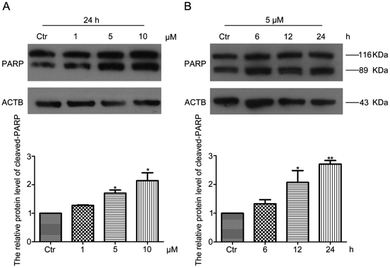 |
| Fig. 5 HF-NBD induces apoptosis in HeLa cells. HeLa cells were treated with 1‰ DMSO or HF-NBD at 1, 5, and 10 μM for 24 h (A), and with 1‰ DMSO for 12 h or HF-NBD at 5 μM for 6, 12, and 24 h (B); cleaved-PARP (89 kDa) protein levels were determined by western blot. Relative cleaved-PARP levels were normalized to ACTB levels and results were expressed as percent of control. Data are presented as the mean ± SEM, *p < 0.05, **p < 0.01, n = 3. | |
It has been reported that ROS may induce cell apoptosis,30 which is consistent with our data. However, some reports claim that activating Nrf2 may induce cancer cell apoptosis.31 Reducing the endogenous H2S levels in cells may affect sulfhydration or sulfidation,9 impair the balance between H2S production and clearance in cells,10 and modulate the levels of some protein kinases or proteins, as well as the level of gene expression associated with apoptosis, including Nrf2 expression.32–35 However, specific issues need specific analysis. Our next step is to establish which proteins are affected by reduced H2S levels, triggering ROS production and activating Nrf2, but here, we propose a potential pathway and mainly focus on the applications of HF-NBD. Thus, we reached the conclusion that HF-NBD can inhibit cancer cell growth by reducing endogenous H2S levels and increasing ROS levels, activating the Nrf2/HO-1 pathway and inducing cell apoptosis.
Experimental
Materials and methods
Cell culture. The human cervical cancer cell line HeLa was cultured in Dulbecco Modified Eagle Medium (DMEM, Gibco, 12800-058) with 10% (v/v) new born calf serum. The human malignant glioma cell line U87 was cultured in Dulbecco Modified Eagle Medium (DMEM, Gibco, 12800-058) with 10% (v/v) fetal bovine serum. The human lung cancer cell line A549 was cultured in RPMI-1640 medium (Gibco, 3180-022) with 10% (v/v) new born calf serum. HeLa cells stably transfected with a luciferase-based Nrf2 reporter plasmid were cultured in Dulbecco Modified Eagle Medium (DMEM, Gibco, 12800-058) with 10% new born calf serum. All cell lines were allowed to grow in air with 5% CO2 and appropriate humidity levels at 37 °C. Cells were seeded in 96-well plates or other types of dishes at a density of 40
000 cells per ml. HeLa cells and A549 cells were purchased from the ATCC (American Type Culture Collection), U87 cells were provided by Bing Yan (Institute of Analytic Chemistry, School of Chemistry and Chemical Engineering, Shandong University, Jinan, China), and HeLa cells stably transfected with a luciferase-based Nrf2 reporter plasmid were provided by Qing Ye and Fan Jiang (The Key Laboratory of Cardiovascular Remodeling and Function Research, Chinese Ministry of Education and Chinese Ministry of Health, Qilu Hospital, Shandong University, Jinan, China).
Fluorescence imaging. HeLa cells were seeded in appropriate dishes or plates and subsequently treated with 1‰ DMSO or 1, 5, 10 μM HF-NBD for 0.5, 1, and 3 h. HeLa cells were seeded and 200 μM Cys, 1 mM PAG, 1 mM AOAA, or 1 mM PAG/AOAA were separately added followed by incubation with 5 μM HF-NBD. HeLa cells were seeded and 0, 0.1, 0.5, 1 mM NaHS was added followed by incubation with 5 μM HF-NBD. HeLa cells were seeded and treated with 5 μM HF-NBD for 1 h. After treatment, cells were washed twice with PBS and imaged under a laser scanning confocal microscopy (LSM 700) instrument at an excitation wavelength of 405 nm.
Luciferase assay. HeLa cells stably transfected with a luciferase-based Nrf2 reporter plasmid were seeded in 96-well plates at a density of 40
000 cells per ml and cultured for at least 12 h, then incubated with HF-NBD at 10 μM for 12 h or 24 h. Luciferase activity in cells was determined with the Luciferase Reporter Gene Assay Kit (Beyotime, China) and results were normalized to cell viability as measured by the SRB assay.
Quantitative real-time PCR. Total RNA was extracted using the Trizol reagent (Invitrogen, USA). The PrimeScript RT reagent kit with gDNA Eraser (DRR047, TAKARA) was used for the reverse transcription. Relative HO-1 mRNA levels were quantified by RT-PCR with the SYBR Premix Ex Taq kit (Tli RNaseH Plus). Results were normalized to β-actin expression with a melting curve for each reaction. Primers for HO-1 were: sense TGCACATCCGTGCAGAGAAT; antisense CTGGGTTCTGCTTGCTTGTTTCGC. Primers for β-actin were: sense GAAGTGTGACGTGGACATCC; antisense CCGATCCACACGGAGTACTT. Primers for Nrf2 were: sense ACACGGTCCACAGCTCATC; antisense GTCCTGTACCTAAACTAACTGT.
Measurement of intracellular ROS levels. HeLa cells were seeded in 24-well plates and intracellular ROS levels were measured with 2′,7′-dichlorodihydrofluorescein (DCHF, Sigma-Aldrich). Cells were incubated with 10 μM DCHF at 37 °C for 30 min after treatment with 10 μM HF-NBD for 3, 6, 12, and 24 h. Then, cells were washed 3 times with PBS and photographed using an Olympus BH-2 (Japan) fluorescence microscope.
Cell viability assay (SRB). HeLa cells were cultured in 96-well plates at a density of 40
000 cells per ml, with each well containing 100 μl of cell suspension. Cells were treated with 1‰ DMSO or HF-NBD and 5-FU at 1, 2, 5, and 10 μM for 12 h or 24 h. Cell viability was measured by the SRB assay following the manufacturer's instructions. U87 cells and A549 cells were treated under the same conditions.
LDH assay. Cell culture medium was collected after treatment with 1‰ DMSO (control) and HF-NBD at 1, 5, 10, and 20 μM for 24 h. The LDH assay was performed with the lactate dehydrogenase (LDH) kit (Nanjing Jiancheng Co, China) following the manufacturer's instructions.
Western blot. HeLa cells were treated with 1‰ DMSO or HF-NBD at 1, 5, and 10 μM for 24 h, and with 1‰ DMSO for 12 h or HF-NBD at 5 μM for 6, 12, and 24 h. Subsequently, cells were washed twice with PBS and lysed in 80 μl of protein lysis buffer (Shanghai beyotime Co., China). All cell lysates were centrifuged at 12
000 × g for 15 min at 4 °C. Then, protein concentrations were analyzed with the bicinchoninic acid (BCA) protein assay kit (Beyotime Co, China). The SDS-PAGE assay was performed at 4 °C for 2 h, then proteins were transferred to PVDF membranes (Millipore, USA) at 4 °C for 2 h. The PVDF membranes were blocked with 5% non-fat milk in Tris-buffered saline containing 1‰ Tween 20 (TBST) at room temperature for 1 h. Membranes were incubated with anti-PARP (Cell Signaling, Beverly, MA, USA) and anti-β-actin (Santa Cruz Biotechnology, Dallas, TX, USA) antibodies overnight at 4 °C. Then, membranes were washed 3 times with TBST for 5 min and incubated with HRP-conjugated secondary antibodies in 5% non-fat milk in TBST for 1 h at room temperature. Subsequently, membranes were washed 3 times with TBST and incubated with HRP substrate for 3 min. Fluorescence was measured using X-ray films and relative protein levels were quantified with the Image J software.
Statistical analysis. All data were presented as the mean ± SEM of the results from at least three independent repeated experiments and analyzed using the Statistical Package for the Social Sciences or the GraphPad Prism software. When the p value was <0.05, differences were considered statistically significant.
Conclusions
HF-NBD, which targets H2S with good photostability, can activate Nrf2 and induce cell apoptosis. We suggested that HF-NBD could reduce endogenous H2S levels in cells, increase intracellular ROS levels and activate the Nrf2/HO-1 pathway, ultimately inducing apoptosis in HeLa cells. HF-NBD, which consumes endogenous H2S and reducing the its levels in cancer cells, functions as an Nrf2 activator, and may have potential as an anticancer drug with specific targeting to H2S.
Conflicts of interest
There are no conflicts of interest to declare.
Acknowledgements
This work was supported by the National Natural Science Foundation of China (No. 31570834, 91313303, 31270877), the Major Project of Science and Technology of the Shandong Province (No. 2015ZDJS04001 and 2015ZDJS04003), the Shandong Excellent Young Scientist Award Fund (No. BS2013SW001) and the Science and Technology Development Project of the Shandong Province (2014GSF118158).
Notes and references
- W. Small Jr, M. A. Bacon, A. Bajaj, L. T. Chuang, B. J. Fisher, M. M. Harkenrider, A. Jhingran, H. C. Kitchener, L. R. Mileshkin, A. N. Viswanathan and D. K. Gaffney, Cancer, 2017, 123, 2404–2412 CrossRef PubMed.
- B. Mery, J. B. Guy, A. Swalduz, A. Vallard, C. Guibert, H. Almokhles, M. Ben Mrad, R. Rivoirard, A. T. Falk, P. Fournel and N. Magne, Crit. Rev. Oncol. Hematol., 2015, 96, 319–327 CrossRef PubMed.
- X. Wang, Y. Jia, P. Wang, Q. Liu and H. Zheng, Ultrason. Sonochem., 2017, 37, 592–599 CrossRef CAS PubMed.
- H. Peng, Y. Cheng, C. Dai, A. L. King, B. L. Predmore, D. J. Lefer and B. Wang, Angew. Chem., 2011, 50, 9672–9675 CrossRef CAS PubMed.
- N. Sen, J. Mol. Biol., 2017, 429, 543–561 CrossRef CAS PubMed.
- W. Xu, Z. Zeng, J. H. Jiang, Y. T. Chang and L. Yuan, Angew. Chem., 2016, 55, 13658–13699 CrossRef CAS PubMed.
- V. S. Lin, W. Chen, M. Xian and C. J. Chang, Chem. Soc. Rev., 2015, 44, 4596–4618 RSC.
- X. Chen, X. Zhao, H. Cai, H. Sun, Y. Hu, X. Huang, W. Kong and W. Kong, Redox Biol., 2017, 12, 987–1003 CrossRef CAS PubMed.
- S. Longen, K. F. Beck and J. Pfeilschifter, Pharmacol. Res., 2016, 111, 642–651 CrossRef CAS PubMed.
- H. Kimura, Molecules, 2014, 19, 16146–16157 CrossRef PubMed.
- C. Szabo, Nat. Rev. Drug Discovery, 2007, 6, 917–935 CrossRef CAS PubMed.
- C. Szabo, C. Coletta, C. Chao, K. Modis, B. Szczesny, A. Papapetropoulos and M. R. Hellmich, Proc. Natl. Acad. Sci. U. S. A., 2013, 110, 12474–12479 CrossRef CAS PubMed.
- D. Wu, W. Si, M. Wang, S. Lv, A. Ji and Y. Li, Nitric Oxide, 2015, 50, 38–45 CrossRef CAS PubMed.
- K. W. Kang, S. J. Lee and S. G. Kim, Antioxid. Redox Signaling, 2005, 7, 1664–1673 CrossRef CAS PubMed.
- P. Hou, H. Li and S. Chen, Tetrahedron, 2016, 72, 3531–3534 CrossRef CAS.
- L. He, W. Lin, Q. Xu and H. Wei, Chem. Commun., 2015, 51, 1510–1513 RSC.
- S. Singha, D. Kim, H. Moon, T. Wang, K. H. Kim, Y. H. Shin, J. Jung, E. Seo, S. J. Lee and K. H. Ahn, Anal. Chem., 2015, 87, 1188–1195 CrossRef CAS PubMed.
- M. Martinez-Cutillas, V. Gil, N. Mane, P. Clave, D. Gallego, M. T. Martin and M. Jimenez, Pharmacol. Res., 2015, 93, 52–63 CrossRef CAS PubMed.
- D. D. Jandial, C. A. Blair, S. Zhang, L. S. Krill, Y. B. Zhang and X. Zi, Curr. Cancer Drug Targets, 2014, 14, 181–200 CrossRef CAS PubMed.
- N. M. Khan, A. Haseeb, M. Y. Ansari, P. Devarapalli, S. Haynie and T. M. Haqqi, Free Radical Biol. Med., 2017, 106, 288–301 CrossRef CAS PubMed.
- Y. Lavrovsky, M. L. Schwartzman, R. D. Levere, A. Kappas and N. G. Abraham, Proc. Natl. Acad. Sci. U. S. A., 1994, 91, 5987–5991 CrossRef CAS.
- J. Kim, J. Kim and J. S. Bae, Exp. Mol. Med., 2016, 48, e269 CrossRef CAS PubMed.
- D. Trachootham, J. Alexandre and P. Huang, Nat. Rev. Drug Discovery, 2009, 8, 579–591 CrossRef CAS PubMed.
- P. D. Ray, B. W. Huang and Y. Tsuji, Cell. Signalling, 2012, 24, 981–990 CrossRef CAS PubMed.
- R. Johar, R. Sharma, A. Kaur and T. K. Mukherjee, Anti-Cancer Agents Med. Chem., 2015, 16, 190–199 CrossRef PubMed.
- J. J. Landry, P. T. Pyl, T. Rausch, T. Zichner, M. M. Tekkedil, A. M. Stutz, A. Jauch, R. S. Aiyar, G. Pau, N. Delhomme, J. Gagneur, J. O. Korbel, W. Huber and L. M. Steinmetz, G3: Genes, Genomes, Genet., 2013, 3, 1213–1224 Search PubMed.
- T. Aki, T. Funakoshi and K. Uemura, Toxicology, 2015, 333, 118–126 CrossRef CAS PubMed.
- K. McCall, Curr. Opin. Cell Biol., 2010, 22, 882–888 CrossRef CAS PubMed.
- M. G. Kemp, J. Cell Death, 2017, 9 DOI:10.1177/1179670716685085.
- C. Tapeinos, A. Larranaga, J. R. Sarasua and A. Pandit, Nanomedicine, 2017 DOI:10.1016/j.nano.2017.03.022.
- X. Zhao, W. Dong, Y. Gao, D. S. Shin, Q. Ye, L. Su, F. Jiang, B. Zhao and J. Miao, Sci. Rep., 2017, 7, 3919 CrossRef PubMed.
- Z. Guo, C. S. Li, C. M. Wang, Y. J. Xie and A. L. Wang, Mol. Med. Rep., 2015, 12, 2128–2134 CrossRef CAS PubMed.
- H. Gong, Z. Chen, X. Zhang, Y. Li, J. Zhang, Y. Chen, Y. Ding, G. Zhang, C. Yang, Y. Zhu and Y. Zou, Int. J. Mol. Sci., 2015, 16, 12482–12498 CrossRef CAS PubMed.
- C. Y. Tsai, S. Y. Wen, M. A. Shibu, Y. C. Yang, H. Peng, B. Wang, Y. M. Wei, H. Y. Chang, C. Y. Lee, C. Y. Huang and W. W. Kuo, Int. J. Cardiol., 2015, 195, 300–310 CrossRef PubMed.
- K. Ma, Y. Liu, Q. Zhu, C. H. Liu, J. L. Duan, B. K. Tan and Y. Z. Zhu, PLoS One, 2011, 6, e20525 Search PubMed.
|
This journal is © The Royal Society of Chemistry 2017 |
Click here to see how this site uses Cookies. View our privacy policy here.