DOI:
10.1039/C7RA07230A
(Paper)
RSC Adv., 2017,
7, 38342-38349
DBU-mediated [4 + 2] annulations of donor–acceptor cyclopropanes with 3-aryl-2-cyanoacrylates for the synthesis of fully substituted anilines†
Received
30th June 2017
, Accepted 29th July 2017
First published on 3rd August 2017
Abstract
A facile synthesis of fully substituted anilines by the DBU-mediated [4 + 2] annulation of donor–acceptor 1,1-dicyano-cyclopropanes with 3-aryl-2-cyanoacrylate has been developed. This reaction involves a highly efficient multiple domino sequence consisting of ring opening of donor–acceptor cyclopropanes, regioselective intermolecular Michael addition, intramolecular nucleophilic addition and aromatization as key unit steps. This transformation provides a straightforward synthetic protocol for constructing fully substituted aniline derivatives. The structure of two typical products was confirmed by X-ray crystallography.
Polysubstituted benzenes are very valuable intermediates in the synthesis of numerous natural products and synthetic pharmaceuticals.1 Among polysubstituted benzene derivatives, those containing nitrogen-substituted benzene derivatives as a privileged scaffold have attracted more attention recently and serve as the central pharmacophore in drug discovery. These molecules were investigated extensively as neuraminidase inhibitors,2 antimicrobial agents,3 and biochemical fluorescent agents.4
As a result of the unique photophysical and electronic properties, polysubstituted benzenes also were utilized as a versatile synthetic building block in materials science and continue to draw the interest of a large segment of the scientific community.5 Researchers have shown that polysubstituted benzenes have important fluorescence properties in solution and in the solid state which can play a significant role in optical and electronic devices such as organic lasers, light-emitting diodes (OLEDs), and organic field-effect transistors (OFET).6 Additionally, polysubstituted benzene derivatives also were used to construct the molecular architecture of MOF family and liquid crystals.7 Given their immense pharmaceutical and material usefulness, the development of efficient syntheses of these polysubstituted benzene derivatives has thus attracted many medicinal and material chemists for decades. Traditionally, there were several methods for the preparation of the polysubstituted benzene derivatives including electrophilic or nucleophilic substitutions of benzene ring, coupling reactions catalyzed by transition metals,8 and metalation functionalization reactions.9
Later, cycloaddition or annulation of suitable acyclic precursors has drawn great attention for the structure of polysubstituted benzenes such as the [3 + 2 + 1] Dötz reaction,10 [3 + 3] cyclocondensation,11 [4 + 2] annulations,12 [5 + 1] benzannulation of alkenoyl ketene-acetals and nitroalkane.13 Recently, Xin and co-workers described an appealing strategy to directly construct polysubstituted benzenes via sequential Michael addition, Knoevenagel condensation and nucleophilic cyclization reactions of chalcones with active methylene compounds in guanidinium ionic liquids.14 In the same way, using L-proline as a catalyst in an ionic liquid, the sequential Michael addition and cyclocondensation of arylethylidenemalononitriles with arylidenemalononitriles also afforded polyfunctionalized benzenes.15 In addition, multicomponent reactions as an efficient synthetic strategy have drawn considerable attention over the past decades, because complex products are formed in a one-pot reaction and diversity can be simply attained by relatively simple starting materials. One-pot multicomponent reactions for the construction of structurally and stereochemically diverse polysubstituted benzenes has been developed using varies catalysts such as biodegradable cellulose sulfuric acid,4 reusable silica nanoparticles,16 N,N-dimethyl-4-aminopyridine (DMAP).17
In recent years, as useful three-carbon synthons, cyclopropanes with donor and acceptor substituents in the vicinal positions (D–A cyclopropanes) have drawn considerable attention from synthetic chemists. D–A cyclopropanes easily underwent a variety of ring-opening reactions under the influence of a variety of conditions to form 1,3-dipolarophiles, which were employed to react with a variety of dipolarophiles such as carbonyls, alkenes, imines, nitriles, and nitrones to construct varied important heterocycles.18 In addition, D–A cyclopropanes as appropriate candidates also underwent cyclodimerization in the absence of dipolarophiles or other reagents.19 Very recently, our group disclosed an efficient and straightforward synthetic protocol for the preparation of fully substituted benzenes via a [3 + 3]-cyclodimerization reaction of 1-cyanocyclopropane 1-esters.11c
Owing to that 2,6-dicyanoanilines belong to typical acceptor–donor–acceptor (A–D–A) systems, which are the basis for artificial photosynthetic systems, materials possessing semiconducting or nonlinear properties, and molecular electronic devices.20 The development of methodologies for a direct access to highly substituted and specifically functionalized 2,6-dicyanoanilines is a continuing challenge in modern synthetic organic chemistry.
In continuation of our interests in D–A cyclopropanes and constructing cyclic compounds, based on our studies on the reactivity of 2-aroyl-3-aryl-1-cyanocyclopropane-1-carboxyl-ates,21 we envisioned that the reaction of D–A 1,1-dicyanocyclopropanes which supply three carbons from the skeleton of cyclopropane and other carbon from its cyano group with substituted 3-aryl-2-cyanoacrylates may offer an efficient approach to benzene skeletons via [4 + 2] annulations and aromatization. To the best of our knowledge, no example using [4 + 2] annulations of D–A 1,1-dicyanocyclopropanes as starting materials to construct the 2,6-dicyanoaniline core were reported. In this context, the [4 + 2] annulations of D–A 1,1-dicyanocyclopropanes with substituted 3-aryl-2-cyanoacrylates could provide an easy access to highly substituted 2,6-dicyanoaniline frameworks.
Based on our more recent results,21 the donor–acceptor 1,1-dicyanocyclopropanes was easily promoted by basic agents to form a 1,3-dipole, the following 2-aroyl-1,1-dicyano-3-arylprop-2-en-1-ide anion generated via removal of a proton, which could attack an electron-defect double or triple bond as a nucleophilic agent.21 The reaction was carried out firstly by stirring the mixture of 2-benzoyl-3-(4-methoxyphenyl)cyclopropane-1,1-dicarbonitrile (1a), ethyl 2-cyano-3-(m-tolyl)acrylate (2a) and Et3N in dichloromethane at 20 °C for 24 h, the desired product was not obtained (Table 1, entry 1). Then, reaction of cyclopropane-1,1-dicarbonitrile 1a and 2-cyanoacrylate 2a using Et3N in dichloromethane resulted in the formation of 2,6-dicyanoaniline 3a in only 29% at 40 °C after 24 h (Table 1, entry 2). Exploring the effect of various bases, DBU, DABCO, piperidine, and NaOH were employed under similar reaction conditions (entries 3–6). Pleasingly, DBU and DABCO gave decent yields of 2,6-dicyanoaniline 3a (entries 3–4). Using piperidine as a base, only 5% of 2,6-dicyanoaniline 3a were obtained (entry 5), and in the presence of NaOH, no reaction took place (entry 6). Considering the replacement of dichloromethane by 1,2-DCE, toluene, and DMF for the raising of reaction temperature, the changing the solvent to 1,2-DCE instead of dichloromethane at 40 °C, 70 °C and 80 °C, respectively, did not have a significant improvement (entries 7–9), however, the results indicated the important role of the reaction temperature, the higher temperature was propitious to the formation of 2,6-dicyanoaniline 3a. The aforementioned experiments showed that both toluene and DMF play crucial roles in generating the 1,3-dipole from donor–acceptor cyclopropanes. In order to improve the yield of product 3a, switching the solvent to toluene or DMF did not give the best yields of the desired product 3a under high-temperature conditions, indicating the important role of the solvent and reaction temperature (entries 10–14). It was worth mentioning at this point that in DMF, the desired product 3a was obtained hardly any, but the formation of 3,6-bisbenzoyl-4,5-bis(4-methoxyphenyl)phthalonitrile was observed via [3 + 3] dimerization of cyclopropane-1,1-dicarbonitrile 1a, which was consistent with our previous reports.11c However, higher loading of DBU (to 1.25 equiv.) under otherwise identical conditions did not significantly improve the yield of 2,6-dicyanoaniline 3a, the reaction time was shortened slightly (entry 15). Subsequently, reducing the amount of DBU from 1.0 equiv. to 0.75 equiv. gave the expected product 3a in 85% yield (Table 1, entry 16), lower than that yield of product 3a using 1.0 equiv. DBU, furthermore the longer reaction time was needed.
Table 1 Optimization of reaction conditions in the synthesis of 3aa
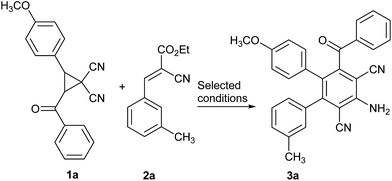
|
Entry |
Base |
Solvent |
T (°C) |
t (h) |
Yieldb (%) |
Reaction conditions: 1 mmol of 1a, 1.2 equiv. of 2a, appropriate basic reagent, 15 mL solvent. Isolated yield. |
1 |
Et3N (1.0) |
DCM |
20 |
24 |
0 |
2 |
Et3N (1.0) |
DCM |
40 |
24 |
29 |
3 |
DBU (1.0) |
DCM |
40 |
10 |
90 |
4 |
DABCO (1.0) |
DCM |
40 |
12 |
75 |
5 |
Piperidine (1.0) |
DCM |
40 |
24 |
<5 |
6 |
NaOH (1.0) |
DCM |
40 |
12 |
0 |
7 |
DBU (1.0) |
1,2-DCE |
40 |
12 |
48 |
8 |
DBU (1.0) |
1,2-DCE |
70 |
10 |
75 |
9 |
DBU (1.0) |
1,2-DCE |
80 |
10 |
86 |
10 |
DBU (1.0) |
Toluene |
40 |
12 |
43 |
11 |
DBU (1.0) |
Toluene |
100 |
10 |
85 |
12 |
DBU (1.0) |
Toluene |
110 |
9 |
83 |
13 |
DBU (1.0) |
DMF |
40 |
24 |
Trace |
14 |
DBU (1.0) |
DMF |
100 |
24 |
Trace |
15 |
DBU (1.25) |
DCM |
40 |
9 |
90 |
16 |
DBU (0.75) |
DCM |
40 |
14 |
85 |
A series of experiments revealed that the optimal results were obtained when the reaction of 2-benzoyl-3-(4-methoxyphenyl)-cyclopropane-1,1-dicarbonitrile (1a) and 1.2 equiv. ethyl 2-cyano-3-(m-tolyl)acrylate (2a) together with 1.0 equiv. DBU was carried out in dichloromethane, the resultant mixture was stirred for 10 h at 40 °C, whereby the yield of product 3a reached 90% (Table 1, entry 3).
Under the optimized conditions as described in entry 3, Table 1, the generality of the reaction was examined in Table 2. The reaction tolerates different substituents on the 1,1-dicyanocyclopropanes and substituted 3-aryl-2-cyanoacrylates, generally, 1,1-dicyanocyclopropanes with a range of substituents at aryl such as methoxy, methyl, chloro, and bromo groups all worked well to give 2,6-dicyanoaniline derivatives. Substrate 3-aryl-2-cyanoacrylates at aryl with electron-withdrawing group such as chloro, bromo, fluoro and ester gave the products in higher yields than the aryl with electron-donating methoxy and methyl. The electronic properties and positions of the substituents on the benzene ring of 3-aryl-2-cyanoacrylates had a slight effect on the reaction. Additionally, substrate 3-aryl-2-cyanoacrylate with a heterocycle such as thiophene also worked well to give the corresponding products. The molecular structures of all 2,6-dicyanoanilines 3a–x were elucidated from their spectroscopic analyses as described herein for 3a. In the IR spectrum of 3a, two sharp absorption bands at 2221 and 1665 cm−1 could be related to CN and PhCOAr stretching frequencies. The high-resolution mass spectrum of 3a displayed the molecular ion peak at m/z = 466.1530 [(M + Na)+], which is in good agreement with the proposed structure. The 1H NMR spectrum of 3a exhibited three sharp singlet signals at 5.31 ppm (bs, 2H), 3.52 ppm (s, 3H), 2.17 ppm (s, 3H) for Ar–NH2, ArOCH3 and ArCH3, respectively. Characteristic 1H chemical shift of Ar–NH2, ArOCH3 and ArCH3 unequivocally indicated the exclusive chemical environment of 2,6-dicyanoaniline 3a protons. The 1H-decoupled 13C NMR spectrum of 3a showed 24 distinct signals in agreement with the suggested structure. The important peaks were related to the ArCO, COCH3, and PhCH3 groups which appeared at = 193.4, 54.1, and 20.4 ppm (see ESI†).
Table 2 Scope with respect to D–A cyclopropanes and 2-cyanoacrylatesa
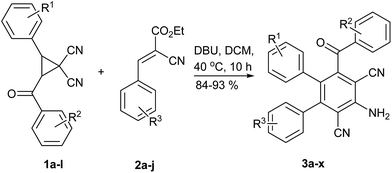
|
Entry |
R1 |
R2 |
R3 |
Yieldb (%) |
Reaction conditions: substituted 1,1-dicyanocyclopropanes 1a–k (1 mmol), substituted 3-aryl-2-cyanoacrylates 2a–k (1.2 mmol), DBU (152 mg, 1.0 mmol), dichloromethane (15 mL), 40 °C, 10 h. Isolated yield. Ethyl-2-cyano-3-(thiophen-2-yl)acrylate was used as a substrate 3-aryl-2-cyanoacrylate. |
1 |
p-CH3O |
H |
m-CH3 |
90 (3a) |
2 |
m-CH3 |
p-Br |
m-CH3 |
90 (3b) |
3 |
m-CH3 |
p-Br |
p-CH3O |
87 (3c) |
4 |
m-CH3 |
p-Br |
p-Cl |
91 (3d) |
5 |
m-CH3 |
p-Br |
p-Br |
92 (3e) |
6 |
m-CH3 |
p-Br |
o-CH3 |
89 (3f) |
7 |
p-Cl |
H |
m-CH3 |
89 (3g) |
8 |
p-Cl |
H |
p-Br |
92 (3h) |
9 |
p-CH3O |
H |
p-Cl |
90 (3i) |
10 |
p-CH3O |
p-Br |
p-Cl |
88 (3j) |
11 |
m-Cl |
m-CH3 |
m-Cl |
93 (3k) |
12 |
m-Cl |
m-CH3 |
p-Br |
92 (3l) |
13 |
p-Cl |
m-CH3 |
p-CH3 |
89 (3m) |
14 |
m-CH3 |
H |
m-Br |
91 (3n) |
15 |
m-CH3 |
H |
Thiophen-2- |
84 (3o) |
16 |
m-CH3 |
H |
m-Cl |
89 (3p) |
17 |
m-CH3 |
m-CH3 |
m-Cl |
91 (3q) |
18 |
p-CH3O |
m-CH3 |
o-CH3 |
88 (3r) |
19 |
m-CH3 |
m-CH3 |
p-Cl |
90 (3s) |
20 |
m-Clc |
m-CH3 |
Thiophen-2- |
86 (3t) |
21 |
p-Cl |
H |
3,5-Cl2C6H4 |
93 (3u) |
22 |
m-CH3 |
H |
p-PhO |
88 (3v) |
23 |
p-CO2CH3 |
m-CH3O |
m-CH3 |
84 (3w) |
24 |
p-F |
m-CH3O |
m-CH3 |
89 (3x) |
Final confirmation for the formation of the reaction products was obtained by X-ray crystal structure analysis of compounds 3b and 3i. The structures of 3b and 3i was unambiguously solved by X-ray crystallography (Fig. 1).22 X-ray crystallographic analysis determined that products 3b and 3i possess two aryl and an aroyl contiguous substituents at C(3), C(4) and C(5) of 2,6-dicyanoaniline core.
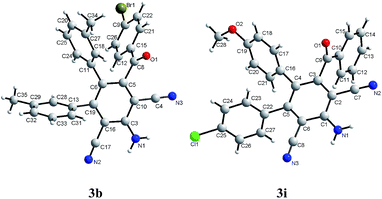 |
| Fig. 1 Molecular structure of 2,6-dicyanoanilines 3b and 3i. | |
Based on the above experimental results, a possible mechanism is proposed in Scheme 1. Initially, the cyclopropane was activated by base generated a zwitterions [A], which formed easily 2-aroyl-1,1-dicyano-3-arylprop-2-en-1-ide [B].21 The Michael addition of 2-aroyl-1,1-dicyano-3-arylprop-2-en-1-ide [B] to the substrate ethyl 3-aryl-2-cyanoacrylate afforded the intermediate 5-aroyl-2,6,6-tricyano-1-ethoxy-1-oxo-3,4-diarylhex-5-en-2-ide [C]. Subsequently, the intramolecular nucleophilic addition of intermediate [C] to C6-cyano group formed the intermediate 6′-aroyl-3′,5′-dicyano-4′-imino-1′,2′,3′,4′-tetrahydro-[1,1′:2′,1′′-terphenyl]-3′-carboxylate [D] again, which transferred easily to the intermediate enamine ethyl 4′-amino-6′-aroyl-3′,5′-dicyano-2′,3′-dihydro-[1,1′:2′,1′′-terphenyl]-3′-carboxylate [E] in the presence of base DBU via 1,5-H shift. The following base DBU-promoted the tautomerism of the intermediate [E] gave the intermediate 4′-amino-2′,3′-dihydro-[1,1′:2′,1′′-terphenyl]-3′-carboxylate [F]. The fully substituted benzenes were finally obtained through the aromatization of the intermediate 4′-amino-2′,3′-dihydro-[1,1′:2′,1′′-terphenyl]-3′-carboxylate [F] by removal of ethyl formate in the presence of base DBU.11c,23
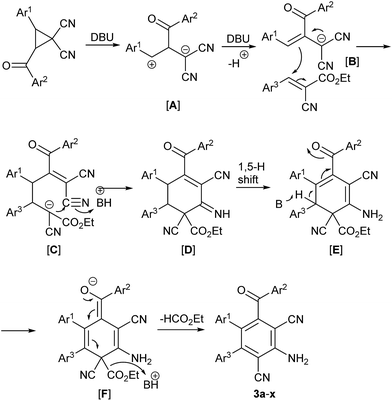 |
| Scheme 1 Tentative reaction mechanism. | |
Conclusions
In conclusion, we have developed an efficient DBU-mediated [4 + 2] annulations of donor–acceptor 1,1-dicyanocyclopropanes with ethyl 3-aryl-2-cyanoacrylate for the preparation of fully substituted anilines which are a wide range of structurally interesting and pharmacologically significant compounds. This reaction involves a highly efficient multiple domino sequence consisting of ring opening of donor–acceptor cyclopropanes, regioselective intermolecular Michael addition, intramolecular nucleophilic addition and aromatization as key unit steps. The reaction appears to be general for a variety of 1,1-dicyanocyclopropanes and 3-aryl-2-cyanoacrylate and tolerates the presence of aromatic moieties with electron-withdrawing and electron-donating substituents. It should be pointed out that this transformation presents a novel synthetic protocol, which is complementary and superior to previous synthetic methods for the formation of fully substituted aniline derivatives.
Experimental section
All melting points were determined in a Yanaco melting point apparatus and are uncorrected. IR spectra were recorded in a Nicolet FT-IR 5DX spectrometer. The 1H NMR (400 MHz) and 13C NMR (100 MHz) spectra were recorded in a Bruker AV-400 spectrometer with TMS as internal reference in CDCl3 solutions. The J values are given in hertz. Only discrete or characteristic signals for the 1H NMR are reported. High-resolution ESI mass spectra were obtained on a UHR-TOF maXis (ESI) mass spectrometer. X-ray crystallographic analysis was performed with a SMART APEX-II diffractometer. Flash chromatography was performed on silica gel (230–400 mesh) eluting with ethyl acetate–hexanes mixture. All reactions were monitored by thin layer chromatography (TLC). All reagents and solvents were purchased from commercial sources and purified commonly before used.
The standard procedure for the synthesis of fully substituted anilines via [4 + 2] reaction between substituted 2-aroyl-3-arylcyclopropane-1,1-dicarbonitriles and 3-aryl-2-cyanoacrylates as follows.
To the mixture of 2-aroyl-3-arylcyclopropane-1,1-dicarbonitriles 1a–k (1.0 mmol) and 3-aryl-2-cyanoacrylates 2a–k (1.2 mmol) in dichloromethane (15 mL) was added DBU (152 mg, 1.0 mmol) at room temperature, and the resultant mixture was stirred at room temperature for 5 min. The resulting mixture was stirred at 40 °C for 10 h, and the completion of reaction was confirmed by TLC (hexanes/EtOAc, 6/1). Subsequently, the resultant mixture was added with water (10 mL) and the water phase was extracted with dichloromethane (15 mL). The combined organic phase was washed with water (5 mL) and brine (5 mL), and dried over anhydrate sodium sulfate. After removal of dichloromethane, the crude product was purified by flash chromatography (silica gel, EtOAc/hexanes, 1/10) to give the desirable products 3a–x.
4′-Amino-6′-benzoyl-4-methoxy-3′′-methyl-[1,1′:2′,1′′-terphenyl]-3′,5′-dicarbonitrile (3a)
Yellow solid, yield: 90%; mp 205.0–205.8 °C (EA/PE); IR (KBr, cm−1): ν 3421, 3323, 3236, 2221, 1665, 1639, 1481, 1255, 931, 825, 751; 1H NMR (400 MHz, CDCl3) δ (ppm): 7.53 (d, J = 6.6 Hz, 2H), 7.40 (s, 1H), 7.24 (s, 2H), 7.13–6.92 (m, 2H), 6.92–6.71 (m, 2H), 6.59 (s, 1H), 6.36 (s, 2H), 5.31 (s, 2H), 3.52 (s, 3H), 2.17 (s, 3H); 13C NMR (100 MHz, CDCl3) δ (ppm): 193.4, 157.8, 150.2, 149.9, 147.3, 137.0, 135.1, 134.5, 133.3, 131.2, 129.2, 128.7, 128.1, 127.7, 127.2, 126.3, 125.7, 114.3, 113.4, 112.3, 98.6, 93.1, 54.1, 20.4; HR-MS (ESI) calcd for C29H21N3O2 [(M + Na)+]: 466.1531; found: 466.1530.
4′-Amino-6′-(4-bromobenzoyl)-3,3′′-dimethyl-[1,1′:2′,1′′-terphenyl]-3′,5′-dicarbonitrile (3b)
White solid, yield: 90%; mp 229.9–230.5 °C (EA/PE); IR (KBr, cm−1): ν 3441, 3332, 3231, 2221, 1673, 1649, 1457, 1253, 1201, 1174, 900, 835, 812; 1H NMR (400 MHz, CDCl3) δ (ppm): 7.37 (s, 4H), 7.10–6.92 (m, 2H), 6.84 (s, 2H), 6.76–6.59 (m, 2H), 6.47 (s, 2H), 5.35 (s, 2H), 2.15 (s, 3H), 1.93 (s, 3H); 13C NMR (100 MHz, CDCl3) δ (ppm): 193.1, 150.9, 150.8, 147.2, 137.8, 137.8, 137.3, 135.6, 134.6, 134.2, 131.8, 131.5, 130.7, 129.9, 129.4, 128.2, 127.9, 127.8, 127.5, 126.4, 115.1, 114.1, 110.0, 99.6, 93.8, 21.2, 20.9; HR-MS (ESI) calcd for C29H20BrN3O [(M + Na)+]: 528.0687; found: 528.0692.
4′-Amino-6′-(4-bromobenzoyl)-4′′-methoxy-3-methyl-[1,1′:2′,1′′-terphenyl]-3′,5′-dicarbonitrile (3c)
Yellow solid, yield: 87%; mp 226.8–227.4 °C (EA/PE); IR (KBr, cm−1): ν 3453, 3336, 3203, 2215, 1678, 1638, 1446, 1244, 1232, 1170, 900, 816, 822; 1H NMR (400 MHz, CDCl3) δ (ppm): 7.43 (s, 3H), 7.25 (s, 1H), 7.03 (d, J = 7.9 Hz, 2H), 6.83–6.70 (m, 4H), 6.54 (s, 1H), 6.50 (d, J = 6.1 Hz, 1H), 5.37 (s, 2H), 3.75 (s, 3H), 2.01 (s, 3H); 13C NMR (100 MHz, CDCl3) δ (ppm): 192.1, 158.9, 149.9, 149.5, 146.2, 136.4, 133.8, 133.2, 130.8, 130.5, 129.9, 129.7, 128.7, 128.4, 127.2, 126.9, 126.7, 114.2, 113.1, 112.6, 98.7, 92.7, 54.1, 20.0; HR-MS (ESI) calcd for C29H20BrN3O2 [(M + Na)+]: 544.0637; found: 544.0639.
4′-Amino-6′-(4-bromobenzoyl)-4′′-chloro-3-methyl-[1,1′:2′,1′′-terphenyl]-3′,5′-dicarbonitrile (3d)
Yellow solid, yield: 91%; mp 213.5–214.0 °C (EA/PE); IR (KBr, cm−1): ν 3447, 3338, 3239, 2225, 1671, 1647, 1419, 1258, 928, 829, 759; 1H NMR (400 MHz, CDCl3) δ (ppm): 7.42–7.29 (m, 4H), 7.19–7.10 (m, 2H), 6.99 (d, J = 7.7 Hz, 2H), 6.81–6.69 (m, 2H), 6.53–6.37 (m, 2H), 5.37 (s, 2H), 1.96 (s, 3H); 13C NMR (100 MHz, CDCl3) δ (ppm): 192.3, 150.3, 148.7, 146.9, 137.1, 134.6, 133.7, 133.6, 133.5, 131.3, 130.8, 130.1, 130.1, 129.5, 129.0, 127.9, 127.3, 127.2, 114.2, 113.3, 98.9, 94.4, 20.4; HR-MS (ESI) calcd for C28H17BrClN3O [(M + Na)+]: 548.0141; found: 548.0140.
4′-Amino-4′′-bromo-6′-(4-bromobenzoyl)-3-methyl-[1,1′:2′,1′′-terphenyl]-3′,5′-dicarbonitrile (3e)
White solid, yield: 92%; mp 222.5–223.6 °C (EA/PE); IR (KBr, cm−1): ν 3449, 3353, 3227, 2220, 1679, 1635, 1447, 1248, 901, 852, 758; 1H NMR (400 MHz, CDCl3) δ (ppm): 7.48–7.34 (m, 6H), 6.98 (d, J = 7.6 Hz, 2H), 6.78–6.68 (m, 2H), 6.62–6.41 (m, 2H), 5.41 (s, 2H), 2.02 (s, 3H); 13C NMR (100 MHz, CDCl3) δ (ppm): 192.8, 150.8, 149.2, 147.5, 137.6, 134.6, 134.2, 134.0, 131.8, 131.4, 131.4, 130.9, 130.6, 129.6, 129.5, 128.5, 127.8, 127.8, 123.5, 114.8, 113.8, 99.3, 94.4, 21.0; HR-MS (ESI) calcd for C28H18Br2N3O [(M + Na)+]: 593.9616; found: 593.9630.
4′-Amino-6′-(4-bromobenzoyl)-2′′,3-dimethyl-[1,1′:2′,1′′-terphenyl]-3′,5′-dicarbonitrile (3f)
Yellow solid, yield: 89%; mp 231.6–232.4 °C (EA/PE); IR (KBr, cm−1): ν 3419, 3356, 3227, 2210, 1674, 1656, 1438, 1235, 910, 873, 782; 1H NMR (400 MHz, CDCl3) δ (ppm): 7.37 (s, 4H), 7.15–7.06 (m, 1H), 7.14–7.01 (m, 3H), 6.70–6.68 (m, 2H), 6.48 (s, 2H), 5.32 (s, 2H), 1.97 (s, 3H), 1.92 (s, 3H); 13C NMR (100 MHz, CDCl3) δ (ppm): 193.1, 151.0, 150.6, 147.1, 137.2, 135.4, 135.0, 134.4, 134.2, 131.8, 130.6, 130.2, 129.9, 129.4, 129.3, 129.1, 128.4, 127.4, 125.5, 114.5, 114.0, 100.2, 94.4, 21.0, 19.7; HR-MS (ESI) calcd for C29H20BrN3O [(M + Na)+]: 528.0687; found: 528.0696.
4′-Amino-6′-benzoyl-4-chloro-3′′-methyl-[1,1′:2′,1′′-terphenyl]-3′,5′-dicarbonitrile (3g)
Yellow solid, yield: 89%; mp 210.5–211.2 °C (EA/PE); IR (KBr, cm−1): ν 3448, 3325, 3226, 2219, 1672, 1638, 1445, 1249, 926, 863, 763; 1H NMR (400 MHz, CDCl3) δ (ppm): 7.53 (d, J = 7.6 Hz, 2H), 7.44 (dd, J = 6.9 Hz and 6.9 Hz, 1H), 7.27 (dd, J = 7.3 Hz and 7.3 Hz, 2H), 7.09–6.96 (m, 2H), 6.82 (d, J = 8.4 Hz, 4H), 6.64 (d, J = 7.0 Hz, 2H), 5.38 (s, 2H), 2.17 (s, 3H); 13C NMR (100 MHz, CDCl3) δ (ppm): 192.8, 150.0, 149.8, 146.9, 137.0, 134.4, 134.1, 133.4, 132.5, 132.5, 131.0, 128.9, 128.7, 128.5, 127.6, 127.2, 126.9, 125.4, 113.9, 113.0, 98.5, 93.0, 20.2; HR-MS (ESI) calcd for C28H18ClN3O [(M + Na)+]: 470.1036; found: 470.1039.
4′-Amino-6′-benzoyl-4′′-bromo-4-chloro-[1,1′:2′,1′′-terphenyl]-3′,5′-dicarbonitrile (3h)
Yellow solid, yield: 92%; mp 250.2–250.8 °C (EA/PE); IR (KBr, cm−1): ν 3441, 3332, 3225, 2216, 1670, 1638, 1437, 1244, 909, 846, 745; 1H NMR (400 MHz, CDCl3) δ (ppm): 7.53 (d, J = 8.0 Hz, 2H), 7.45 (dd, J = 7.2 Hz and 7.2 Hz, 1H), 7.34 (d, J = 8.0 Hz, 2H), 7.28 (dd, J = 7.6 Hz and 7.6 Hz, 2H), 6.91 (d, J = 7.7 Hz, 2H), 6.85 (d, J = 8.0 Hz, 2H), 6.62 (d, J = 7.2 Hz, 2H), 5.39 (s, 2H); 13C NMR (100 MHz, CDCl3) δ (ppm): 192.5, 150.0, 148.2, 147.3, 134.0, 133.5, 133.4, 132.9, 132.0, 131.0, 130.7, 129.9, 128.5, 127.7, 127.2, 126.9, 122.7, 113.7, 112.7, 108.9, 98.2, 93.6; HR-MS (ESI) calcd for C27H15BrClN3O [(M + Na)+]: 533.9985; found: 533.9982.
4′-Amino-6′-benzoyl-4′′-chloro-4-methoxy-[1,1′:2′,1′′-terphenyl]-3′,5′-dicarbonitrile (3i)
Yellow solid, yield: 90%; mp 244.4–245.0 °C (EA/PE); IR (KBr, cm−1): ν 3449, 3336, 3236, 2225, 1671, 1634, 1442, 1264, 931, 843, 749; 1H NMR (400 MHz, CDCl3) δ (ppm): 7.53 (d, J = 7.7 Hz, 2H), 7.36 (dd, J = 7.3 Hz and 7.3 Hz, 1H), 7.20 (dd, J = 7.5 Hz and 7.5 Hz, 2H), 7.16 (d, J = 8.1 Hz, 2H), 6.99 (d, J = 7.2 Hz, 2H), 6.58 (d, J = 6.8 Hz, 2H), 6.38 (d, J = 8.0 Hz, 2H), 5.36 (s, 2H), 3.53 (s, 3H); 13C NMR (100 MHz, CDCl3) δ (ppm): 193.1, 157.9, 150.0, 148.6, 147.6, 134.3, 134.2, 133.6, 133.4, 131.1, 130.0, 128.7, 128.3, 127.7, 125.8, 114.2, 113.2, 112.5, 98.3, 93.6, 54.1; HR-MS (ESI) calcd for C28H18ClN3O2 [(M + Na)+]: 486.0985; found: 486.0991.
4′-Amino-6′-(4-bromobenzoyl)-4′′-chloro-4-methoxy-[1,1′:2′,1′′-terphenyl]-3′,5′-dicarbonitrile (3j)
Yellow solid, yield: 88%; mp 236.8–237.2 °C (EA/PE); IR (KBr, cm−1): ν 3432, 3333, 3237, 2223, 1675, 1649, 1482, 1254, 941, 826, 750; 1H NMR (400 MHz, CDCl3) δ (ppm): 7.39 (s, 4H), 7.17 (d, J = 8.8 Hz, 2H), 6.98 (d, J = 6.7 Hz, 2H), 6.57 (d, J = 6.7 Hz, 2H), 6.41 (d, J = 7.9 Hz, 2H), 5.35 (s, 2H), 3.56 (s, 3H); 13C NMR (100 MHz, CDCl3) δ (ppm): 192.9, 158.8, 150.7, 149.5, 147.7, 135.1, 134.2, 133.9, 131.9, 131.9, 130.7, 129.7, 129.1, 128.6, 126.4, 114.8, 113.8, 113.5, 99.4, 93.2, 55.0; HR-MS (ESI) calcd for C28H17BrClN3O2 [(M + Na)+]: 564.0090; found: 564.0110.
4′-Amino-3,4′′-dichloro-6′-(3-methylbenzoyl)-[1,1′:2′,1′′-terphenyl]-3′,5′-dicarbonitrile (3k)
Yellow solid, yield: 93%; mp 214.9–215.2 °C (EA/PE); IR (KBr, cm−1): ν 3452, 3323, 3236, 2212, 1664, 1648, 1478, 1251, 906, 819, 744; 1H NMR (400 MHz, CDCl3) δ (ppm): 7.35 (s, 1H), 7.30 (d, J = 7.7 Hz, 1H), 7.25 (d, J = 7.5 Hz, 1H), 7.22–7.14 (dd, J = 9.2 Hz and 6.8 Hz, 3H), 6.99 (d, J = 7.7 Hz, 2H), 6.89 (d, J = 8.0 Hz, 1H), 6.81 (dd, J = 7.7 Hz and 7.7 Hz, 1H), 6.67 (s, 1H), 6.58 (d, J = 7.2 Hz, 1H), 5.39 (s, 2H); 13C NMR (100 MHz, CDCl3) δ (ppm): 193.5, 151.0, 149.1, 148.4, 138.6, 136.4, 135.4, 135.3, 135.0, 133.9, 133.7, 130.7, 130.6, 129.6, 129.1, 128.9, 128.7, 128.5, 127.9, 127.8, 126.9, 114.7, 113.8, 99.2, 94.6, 21.5; HR-MS (ESI) calcd for C28H17Cl2N3O [(M + Na)+]: 504.0646; found: 504.0645.
4′-Amino-4′′-bromo-3-chloro-6′-(3-methylbenzoyl)-[1,1′:2′,1′′-terphenyl]-3′,5′-dicarbonitrile (3l)
White solid, yield: 92%; mp 227.5–228.7 °C (EA/PE); IR (KBr, cm−1): ν 3434, 3331, 3238, 2223, 1670, 1643, 1449, 1216, 939, 825, 769; 1H NMR (400 MHz, CDCl3) δ (ppm): 7.36 (s, 2H), 7.30 (d, J = 7.7 Hz, 1H), 7.26 (d, J = 7.6 Hz, 1H), 7.22–7.09 (m, 2H), 6.96–6.85 (m, 3H), 6.81 (dd, J = 7.6 Hz and 7.6 Hz, 1H), 6.68 (s, 1H), 6.58 (d, J = 7.1 Hz, 1H), 5.38 (s, 2H), 2.25 (s, 3H); 13C NMR (100 MHz, CDCl3) δ (ppm): 192.7, 150.3, 148.3, 147.6, 137.8, 135.6, 134.6, 134.2, 133.6, 133.0, 130.9, 130.1, 129.9, 128.9, 128.3, 128.1, 127.7, 127.1, 126.2, 122.9, 113.9, 113.0, 98.3, 93.9, 20.3; HR-MS (ESI) calcd for C28H17BrClN3O [(M + Na)+]: 548.0141; found: 548.0148.
4′-Amino-4-chloro-4′′-methyl-6′-(3-methylbenzoyl)-[1,1′:2′,1′′-terphenyl]-3′,5′-dicarbonitrile (3m)
Yellow solid, yield: 89%; mp 214.6–215.4 °C (EA/PE); IR (KBr, cm−1): ν 3456, 3338, 3235, 2213, 1662, 1639, 1419, 1276, 925, 825, 751; 1H NMR (400 MHz, CDCl3) δ (ppm): 7.39 (s, 1H), 7.32 (d, J = 7.6 Hz, 1H), 7.27 (d, J = 7.3 Hz, 1H), 7.22–7.13 (m, 1H), 7.01 (d, J = 7.7 Hz, 2H), 6.93 (d, J = 7.3 Hz, 2H), 6.85 (d, J = 7.9 Hz, 2H), 6.66 (d, J = 7.4 Hz, 2H), 5.38 (s, 2H), 2.27 (s, 3H), 2.24 (s, 3H); 13C NMR (100 MHz, CDCl3) δ (ppm): 194.0, 151.1, 150.8, 148.1, 139.0, 138.6, 135.3, 135.1, 133.7, 133.5, 132.6, 132.0, 129.6, 129.2, 129.0, 128.5, 127.9, 127.1, 115.1, 114.0, 99.5, 93.9, 21.2, 21.1; HR-MS (ESI) calcd for C29H20ClN3O [(M + Na)+]: 484.1193; found: 484.1193.
4′-Amino-6′-benzoyl-3′′-bromo-3-methyl-[1,1′:2′,1′′-terphenyl]-3′,5′-dicarbonitrile (3n)
Yellow solid, yield: 91%; mp 232.6–233.3 °C (EA/PE); IR (KBr, cm−1): ν 3447, 3330, 3230, 2221, 1676, 1647, 1449, 1249, 969, 846, 740; 1H NMR (400 MHz, CDCl3) δ (ppm): 7.51 (d, J = 7.1 Hz, 2H), 7.40 (d, J = 6.8 Hz, 1H), 7.32 (d, J = 7.7 Hz, 1H), 7.29–7.16 (m, 3H), 7.04 (dd, J = 7.3 Hz and 7.3 Hz, 1H), 6.99 (s, 1H), 6.72 (d, J = 7.1 Hz, 1H), 6.69 (s, 1H), 6.49 (s, 2H), 5.37 (s, 2H), 1.93 (s, 3H); 13C NMR (100 MHz, CDCl3) δ (ppm): 192.7, 149.8, 147.6, 147.2, 136.8, 136.4, 134.3, 133.2, 133.1, 131.3, 130.8, 130.4, 128.6, 128.4, 127.4, 127.4, 127.0, 126.8, 126.6, 121.0, 113.8, 112.9, 98.1, 93.6, 19.9; HR-MS (ESI) calcd for C28H18BrN3O [(M + Na)+]: 514.0531; found: 514.0528.
4-Amino-2-benzoyl-3′-methyl-6-(thiophen-2-yl)-[1,1′-biphenyl]-3,5-dicarbonitrile (3o)
White solid, yield: 84%; mp 245.2–245.9 °C (EA/PE); IR (KBr, cm−1): ν 3452, 3326, 3239, 2206, 1674, 1643, 1472, 1272, 932, 859, 709; 1H NMR (400 MHz, CDCl3) δ (ppm): 7.51 (d, J = 8.2 Hz, 2H), 7.41 (dd, J = 6.9 Hz and 6.9 Hz, 1H), 7.25 (dd, J = 6.9 Hz and 6.9 Hz, 3H), 7.04–7.02 (m, 1H), 6.89–6.84 (m, 1H), 6.88 (dd, J = 7.5 Hz and 7.5 Hz, 2H), 6.54 (d, J = 11.6 Hz, 2H), 5.34 (s, 2H), 1.95 (s, 3H); 13C NMR (100 MHz, CDCl3) δ (ppm): 192.7, 149.9, 147.0, 142.3, 136.4, 135.0, 134.3, 133.6, 133.1, 130.5, 129.3, 129.1, 128.4, 128.2, 127.5, 127.4, 126.8, 126.6, 125.7, 114.2, 113.0, 105.6, 98.5, 93.3, 20.0; HR-MS (ESI) calcd for C26H17N3OS [(M + Na)+]: 442.0990; found: 442.0989.
4′-Amino-6′-benzoyl-3′′-chloro-3-methyl-[1,1′:2′,1′′-terphenyl]-3′,5′-dicarbonitrile (3p)
Yellow solid, yield: 89%; mp 232.9–233.6 °C (EA/PE); IR (KBr, cm−1): ν 3455, 3319, 3233, 2209, 1671, 1645, 1489, 1259, 903, 829, 752; 1H NMR (400 MHz, CDCl3) δ (ppm): 7.52 (d, J = 7.9 Hz, 2H), 7.41 (dd, J = 7.4 Hz and 7.4 Hz, 1H), 7.25 (dd, J = 7.7 Hz and J = 7.7 Hz, 2H), 7.18 (d, J = 2.9 Hz, 1H), 7.11 (dd, J = 7.8 Hz and 7.8 Hz, 1H), 7.06 (s, 1H), 6.94 (d, J = 7.5 Hz, 1H), 6.76–6.64 (m, 2H), 6.53–6.42 (m, 2H), 5.34 (s, 2H), 1.93 (s, 3H); 13C NMR (100 MHz, CDCl3) δ (ppm): 192.8, 149.8, 147.8, 147.2, 136.6, 136.4, 134.3, 133.2, 133.1, 133.0, 130.4, 128.4, 127.9, 127.4, 127.3, 126.8, 126.6, 126.6, 113.8, 113.0, 98.1, 93.6, 19.9; HR-MS (ESI) calcd for C27H15ClN3O [(M + Na)+]: 470.1036; found: 470.1034.
4′-Amino-3′′-chloro-3-methyl-6′-(3-methylbenzoyl)-[1,1′:2′,1′′-terphenyl]-3′,5′-dicarbonitrile (3q)
Yellow solid, yield: 91%; mp 206.9–207.6 °C (EA/PE); IR (KBr, cm−1): ν 3447, 3328, 3239, 2226, 1668, 1645, 1471, 1250, 966, 824, 728; 1H NMR (400 MHz, CDCl3) δ (ppm): 7.34 (s, 1H), 7.30 (d, J = 7.7 Hz, 1H), 7.24–7.04 (m, 5H), 6.94 (d, J = 7.4 Hz, 1H), 6.78–6.62 (m, 2H), 6.47 (d, J = 10.3 Hz, 2H), 5.35 (s, 2H), 2.23 (s, 3H), 1.94 (s, 3H); 13C NMR (100 MHz, CDCl3) δ (ppm): 193.3, 150.2, 148.2, 147.8, 137.8, 137.1, 136.8, 134.7, 134.4, 133.8, 133.5, 130.9, 129.1, 129.0, 128.9, 128.8, 128.3, 127.8, 127.7, 127.2, 127.0, 127.0, 126.4, 114.2, 113.4, 98.5, 94.1, 20.5, 20.4; HR-MS (ESI) calcd for C29H20ClN3O [(M + Na)+]: 484.1193; found: 484.1191.
4′-Amino-4-methoxy-2′′-methyl-6′-(3-methylbenzoyl)-[1,1′:2′,1′′-terphenyl]-3′,5′-dicarbonitrile (3r)
White solid, yield: 88%; mp 196.2–197.1 °C (EA/PE); IR (KBr, cm−1): ν 3435, 3330, 3235, 2224, 1661, 1639, 1459, 1249, 904, 853, 736; 1NMR (400 MHz) δ (ppm): 7.37 (s, 1H), 7.31 (d, J = 7.4 Hz, 1H), 7.24–7.17 (m, 2H), 7.13 (dd, J = 7.5 Hz and 7.5 Hz, 1H), 6.97 (d, J = 7.4 Hz, 2H), 6.70 (d, J = 8.4 Hz, 2H), 6.60 (d, J = 7.2 Hz, 2H), 6.38 (d, J = 7.9 Hz, 2H), 5.29 (s, 2H), 3.69 (s, 3H), 3.54 (s, 3H), 2.23 (s, 3H); 13C NMR (100 MHz, CDCl3) δ (ppm): 193.5, 158.9, 157.7, 149.9, 149.8, 147.5, 137.6, 134.4, 134.2, 131.1, 130.1, 130.1, 128.9, 128.5, 127.5, 127.3, 126.5, 126.3, 114.6, 113.4, 112.8, 112.4, 109.2, 98.6, 92.9, 54.3, 54.1, 20.3; HR-MS (ESI) calcd for C30H23N3O2 [(M + Na)+]: 480.1688; found: 480.1686.
4′-Amino-4′′-chloro-3-methyl-6′-(3-methylbenzoyl)-[1,1′:2′,1′′-terphenyl]-3′,5′-dicarbonitrile (3s)
Yellow solid, yield: 90%; mp 198.5–199.4 °C (EA/PE); IR (KBr, cm−1): ν 3456, 3339, 3237, 2218, 1676, 1656, 1479, 1259, 921, 829, 729; 1H NMR (400 MHz, CDCl3) δ (ppm): 7.34 (s, 1H), 7.30 (d, J = 7.7 Hz, 1H), 7.24–7.18 (m, 1H), 7.18–7.09 (m, 3H), 7.00 (d, J = 7.9 Hz, 2H), 6.76–6.65 (m, 2H), 6.50–6.42 (m, 2H), 5.37 (s, 2H), 2.22 (s, 3H), 1.93 (s, 3H); 13C NMR (100 MHz, CDCl3) δ (ppm): 193.2, 150.1, 148.3, 147.6, 137.5, 136.6, 134.4, 134.2, 133.7, 133.6, 130.7, 130.0, 128.9, 128.7, 127.6, 127.5, 127.0, 126.9, 126.2, 114.2, 113.2, 98.3, 93.7, 20.3, 20.2; HR-MS (ESI) calcd for C29H20ClN3O [(M + Na)+]: 484.1193; found: 484.1187.
4-Amino-3′-chloro-2-(3-methylbenzoyl)-6-(thiophen-2-yl)-[1,1′-biphenyl]-3,5-dicarbonitrile (3t)
White solid, yield: 86%; mp 246.8–247.4 °C (EA/PE); IR (KBr, cm−1): ν 3449, 3328, 3236, 2227, 1669, 1643, 1461, 1229, 946, 859, 746; 1H NMR (400 MHz, CDCl3) δ (ppm): 7.43–7.34 (m, 4H), 7.28–7.24 (m, 1H), 7.07–7.02 (m, 1H), 6.92–6.86 (m, 1H), 6.80 (dd, J = 7.8 Hz and 7.8 Hz, 2H), 6.60–6.50 (m, 2H), 5.34 (s, 2H), 2.00 (s, 3H); 13C NMR (100 MHz, CDCl3) δ (ppm): 191.4, 149.7, 146.0, 142.1, 136.3, 134.6, 133.2, 132.8, 130.6, 130.2, 129.4, 129.1, 128.7, 128.3, 128.1, 127.5, 126.5, 126.4, 125.5, 113.9, 112.7, 98.4, 92.9, 19.8; HR-MS (ESI) calcd for C26H16ClN3OS [(M + Na)+]: 476.0600; found: 476.0613.
4′-Amino-6′-benzoyl-3′′,4,5′′-trichloro-[1,1′:2′,1′′-terphenyl]-3′,5′-dicarbonitrile (3u)
Yellow solid, yield: 93%; mp 197.6–198.5 °C (EA/PE); IR (KBr, cm−1): ν 3448, 3334, 3235, 2221, 1677, 1646, 1468, 1253, 926, 825, 740; 1H NMR (400 MHz, CDCl3) δ (ppm): 7.52 (d, J = 7.4 Hz, 2H), 7.45 (dd, J = 7.4 Hz and 7.4 Hz, 1H), 7.33–7.23 (m, 3H), 7.12–7.05 (m, 1H), 6.89 (d, J = 8.3 Hz, 1H), 6.84 (d, J = 7.8 Hz, 2H), 6.69 (d, J = 7.7 Hz, 2H), 5.36 (s, 2H); 13C NMR (100 MHz, CDCl3) δ (ppm): 192.3, 149.7, 147.3, 145.8, 135.0, 134.0, 133.6, 133.2, 132.4, 132.3, 131.6, 130.3, 128.7, 128.5, 127.7, 127.1, 126.4, 113.0, 112.6, 98.8, 94.3; HR-MS (ESI) calcd for C27H14Cl3N3O [(M + Na)+]: 524.0100; found: 524.0096.
4′-Amino-6′-benzoyl-3-methyl-4′′-phenoxy-[1,1′:2′,1′′-terphenyl]-3′,5′-dicarbonitrile (3v)
Yellow solid, yield: 88%; mp 184.5–185.3 °C (EA/PE); IR (KBr, cm−1): ν 3432, 3321, 3229, 2218, 1672, 1645, 1456, 1249, 1202, 1171, 906, 834, 803; 1H NMR (400 MHz, CDCl3) δ (ppm): δ 7.56 (d, J = 6.8 Hz, 2H), 7.45 (d, J = 6.5 Hz, 1H), 7.34–7.18 (m, 5H), 7.06 (s, 1H), 6.94 (s, 2H), 6.85–6.74 (m, 2H), 6.71 (d, J = 7.0 Hz, 2H), 6.66 (s, 1H), 6.53 (s, 2H), 5.40 (s, 2H), 2.00 (s, 3H); 13C NMR (100 MHz, CDCl3) δ (ppm): 193.9, 156.7, 156.6, 150.8, 149.8, 148.0, 137.5, 137.3, 135.4, 134.7, 134.1, 131.5, 129.8, 129.7, 129.6, 129.4, 128.4, 128.2, 127.8, 127.7, 124.3, 123.3, 119.8, 119.7, 118.6, 114.9, 114.1, 99.3, 94.4, 21.0; HR-MS (ESI) calcd for C34H23N3O2 [(M + Na)+]: 528.1688; found: 528.1692.
Methyl 4′-amino-3′,5′-dicyano-6′-(3-methoxybenzoyl)-3′′-methyl-[1,1′:2′,1′′-terphenyl]-4-carboxylate (3w)
White solid, yield: 84%; mp 229.9–230.5 °C (EA/PE); IR (KBr, cm−1): ν 3443, 3328, 3221, 2219, 1675, 1648, 1453, 1250, 1213, 1171, 907, 832, 820; 1H NMR (400 MHz, CDCl3) δ (ppm): 7.60 (d, J = 8.1 Hz, 2H), 7.27–7.21 (m, 1H), 7.17 (s, 1H), 7.13–7.07 (m, 2H), 7.03 (dd, J = 11.3, 5.1 Hz, 2H), 6.87 (d, J = 7.3 Hz, 4H), 5.42 (s, 2H), 3.79 (s, 3H), 3.76 (s, 3H), 2.21 (s, 3H); 13C NMR (100 MHz, CDCl3) δ (ppm): 193.4, 166.4, 159.8, 151.0, 150.7, 147.8, 140.0, 138.0, 136.4, 135.4, 130.9, 129.9, 129.8, 129.6, 129.0, 128.8, 128.2, 126.4, 122.8, 121.1, 114.9, 113.9, 113.2, 99.6, 94.1, 55.4, 52.0, 21.2; HR-MS (ESI) calcd for C31H23N3O4 [(M + Na)+]: 524.1586; found: 524.1579.
4′-Amino-4-fluoro-6′-(3-methoxybenzoyl)-3′′-methyl-[1,1′:2′,1′′-terphenyl]-3′,5′-dicarbonitrile (3x)
Yellow solid, yield: 89%; mp 229.9–230.5 °C (EA/PE); IR (KBr, cm−1): ν 3439, 3327, 3230, 2225, 1676, 1642, 1449, 1248, 1208, 1172, 918, 832, 815; 1H NMR (400 MHz, CDCl3) δ (ppm): 7.24 (d, J = 8.1 Hz, 1H), 7.18 (s, 1H), 7.12 (dd, J = 7.9 Hz and 7.9 Hz, 2H), 7.08–6.99 (m, 2H), 6.88 (s, 2H), 6.74 (s, 2H), 6.62 (dd, J = 8.2 Hz and 8.2 Hz, 2H), 5.40 (s, 2H), 3.77 (s, 3H), 2.23 (s, 3H); 13C NMR (100 MHz, CDCl3) δ (ppm): 193.7, 161.8 (d, J = 246.8 Hz), 159.8, 151.0, 150.8, 148.1, 138.0, 136.5, 135.6, 132.6, 132.5, 131.0 (d, J = 3.6 Hz), 129.9, 129.6 (d, J = 9.8 Hz), 128.4, 128.1, 126.4, 122.8, 121.0, 114.9, 114.8 (d, J = 21.7 Hz), 114.0, 113.1, 99.5, 94.0, 55.4, 21.2; HR-MS (ESI) calcd for C29H30FN3O2 [(M + Na)+]: 484.1437; found: 484.1432.
Acknowledgements
Financial support of this research by the National Natural Science Foundation of China (NSFC 21173181) is gratefully acknowledged by authors. A project was funded by the Priority Academic Program Development of Jiangsu Higher Education Institutions.
Notes and references
-
(a) D. Astruc, Modern Arene Chemistry, Wiley-VCH, Weinheim, Germany, 2002 CrossRef;
(b) J. Q. Wang, M. Gao, K. D. Miller, G. W. Sledge and Q. H. Zheng, Bioorg. Med. Chem. Lett., 2006, 16, 4102 CrossRef CAS PubMed.
- P. Chand, P. L. Kotian, P. E. Morris, S. Bantia, D. A. Walsh and Y. S. Babu, Bioorg. Med. Chem., 2005, 13, 2665 CrossRef CAS PubMed.
- H. M. Faidallah, K. M. A. Al-Shaikh, T. R. Sobahi, K. A. Khan and A. M. Asiri, Molecules, 2013, 18, 15704 CrossRef CAS PubMed.
- A. Zonouzi, Z. Izakiana, K. Abdic and S. W. Ngd, Helv. Chim. Acta, 2016, 99, 355 CrossRef CAS.
- E. Vishnyakova, G. Chen, B. E. Brinson, L. B. Alemany and W. E. Billups, Acc. Chem. Res., 2017, 50, 1351 CrossRef CAS PubMed.
-
(a) X. Chen, X. Chen, Z. Zhao, P. Lv and Y. Wang, Chin. J. Chem., 2009, 27, 971 CrossRef CAS;
(b) F. Dumur, T.-T. Bui, S. Peralta, M. Lepeltier, G. Wantz, G. Sini, F. Goubard and D. Gigmes, RSC Adv., 2016, 6, 60565 RSC;
(c) D. C. Kim, T. W. Lee, J. E. Lee, K. H. Kim, M. J. Cho, D. H. Choi, Y. D. Han, M. Y. Cho and J. Joo, Macromol. Res., 2009, 17, 491 CrossRef CAS.
-
(a) O. Kozachuk, I. Luz, F. X. Xamena, H. Noei, M. Kauer, H. B. Albada, E. D. Bloch, B. Marler, Y. Wang and M. Muhler, Angew. Chem., Int. Ed., 2014, 53, 7058 CrossRef CAS PubMed;
(b) Y.-H. Ooi, G.-Y. Yeap, C.-C. Han, H.-C. Lin, T. Shinomiya and M. M. Ito, Liq. Cryst., 2014, 41, 1017 CrossRef CAS;
(c) P. Bordat and R. Brown, J. Chem. Phys., 2009, 130, 124501 CrossRef PubMed.
- J. Hassan, M. Sevignon, C. Gozzi, E. Schulz and M. Lemaire, Chem. Rev., 2002, 102, 1359 CrossRef CAS PubMed.
-
(a) V. Snieckus, Chem. Rev., 1990, 90, 879 CrossRef CAS;
(b) J.-P. Wan, Y. Lin, K. Hu and Y. Liu, RSC Adv., 2014, 4, 20499 RSC.
-
(a) K. H. Dotz and P. Tomuschat, Chem. Soc. Rev., 1999, 28, 187 RSC;
(b) H. Wang, J. Huang, W. D. Wulff and A. L. Rheingold, J. Am. Chem. Soc., 2003, 125, 8980 CrossRef CAS PubMed;
(c) A. V. Vorogushin, W. D. Wulff and H. Hansen, J. Am. Chem. Soc., 2002, 124, 6512 CrossRef CAS PubMed.
-
(a) P. Langer and G. Bose, Angew. Chem., Int. Ed., 2003, 42, 4033 CrossRef CAS PubMed;
(b) A. R. Katritzky, J. Li and L. Xie, Tetrahedron, 1999, 55, 8263 CrossRef CAS;
(c) W. Ye, L. Zhou, S. Xue, Y. Li and C. Wang, Synlett, 2015, 26, 1769 CrossRef CAS.
-
(a) L. V. R. Bonaga, H. C. Zhang, A. F. Moretto, H. Ye, D. A. Gauthier, J. Li, G. C. Leo and B. E. Maryanoff, J. Am. Chem. Soc., 2005, 127, 3473 CrossRef CAS PubMed;
(b) S. Saito and Y. Yamamoto, Chem. Rev., 2000, 100, 2901 CrossRef CAS PubMed;
(c) M. J. Lee, K. Y. Lee, S. Gowrisankar and J. N. Kim, Tetrahedron Lett., 2006, 47, 1355 CrossRef CAS;
(d) D. Xue, J. Li, Z. T. Zhang and J. G. Deng, J. Org. Chem., 2007, 72, 5443 CrossRef CAS PubMed;
(e) N. M. Helmy, F. E. M. El-Baih, M. A. Al-Alshaikh and M. S. Moustafa, Molecules, 2011, 16, 298 CrossRef CAS PubMed.
-
(a) X. Bi, D. Dong, Q. Liu, W. Pan, L. Zhau and B. Li, J. Am. Chem. Soc., 2005, 127, 4578 CrossRef CAS PubMed;
(b) O. Barun, S. Nandi, K. Panda, H. Ila and H. Junjappa, J. Org. Chem., 2002, 67, 5398 CrossRef CAS PubMed.
- X. Xin, Y. Wang, W. Xu, Y. Lin, H. Duan and D. Dong, Green Chem., 2010, 12, 893 RSC.
- S. Jain, B. S. Keshwal and D. Rajguru, J. Serb. Chem. Soc., 2012, 77, 1345 CrossRef CAS.
- S. Banerjee, A. Horn, H. Khatri and G. Sereda, Tetrahedron Lett., 2011, 52, 1878 CrossRef CAS.
- S. Bhattacharjee and A. T. Khan, Tetrahedron Lett., 2016, 57, 2994 CrossRef CAS.
-
(a) S. J. Gharpure and L. N. Nanda, Tetrahedron Lett., 2017, 58, 711 CrossRef CAS;
(b) A. K. Pandey, A. Ghosh and P. Banerjee, Isr. J. Chem., 2016, 56, 512 CrossRef CAS;
(c) L. Wang and Y. Tang, Isr. J. Chem., 2016, 56, 463 CrossRef CAS;
(d) Y. Xia, L. L. Lin, F. Z. Chang, X. Fu, X. H. Liu and X. M. Feng, Angew. Chem., Int. Ed., 2015, 54, 13748 CrossRef CAS PubMed;
(e) H. K. Grover, M. R. Emmett and M. A. Kerr, Org. Biomol. Chem., 2015, 13, 655 RSC;
(f) F. Tobias, J. K. Schneider and D. B. Werz, Angew. Chem., Int. Ed., 2014, 53, 5504 CrossRef PubMed;
(g) G. Sathishkannan and K. Srinivasan, Adv. Synth. Catal., 2014, 356, 729 CrossRef CAS;
(h) L. K. B. Garve, P. Barkawitz, P. G. Jones and D. B. Werz, Org. Lett., 2014, 16, 5804 CrossRef CAS PubMed;
(i) M. A. Cavitt, L. H. Phun and S. France, Chem. Soc. Rev., 2014, 43, 804 RSC;
(j) M. Y. Melnikov, E. M. Budynina, O. A. Ivanova and I. V. Trushkov, Mendeleev Commun., 2011, 21, 293 CrossRef CAS;
(k) C. A. Carson and M. A. Kerr, Chem. Soc. Rev., 2009, 38, 3051 RSC.
-
(a) R. A. Novikov, V. P. Timofeev and Y. V. Tomilov, J. Org. Chem., 2012, 77, 5993 CrossRef CAS PubMed;
(b) R. A. Novikov and Y. V. Tomilov, Mendeleev Commun., 2015, 25, 1 CrossRef CAS;
(c) O. A. Ivanova, E. M. Budynina, A. O. Chagarovskiy, I. V. Trushkov and M. Y. Melnikov, J. Org. Chem., 2011, 76, 8852 CrossRef CAS PubMed.
-
(a) H. Kurreck and M. Huber, Angew. Chem., Int. Ed. Engl., 1995, 34, 849 CrossRef CAS;
(b) F. Dumur, N. Gautier, N. Gallego-Planas, Y. Sahin, E. Levillain, N. Mercier and P. Hudhomme, J. Org. Chem., 2004, 69, 2164 CrossRef CAS PubMed;
(c) N. J. Long, Angew. Chem., Int. Ed. Engl., 1995, 34, 21 CrossRef CAS.
-
(a) J. Liu, L. Zhou, W. Ye and C. Wang, Chem. Commun., 2014, 50, 9068 RSC;
(b) C. Tan, W. Ye, J. Yao, J. Liu, S. Xue, Y. Li and C. Wang, RSC Adv., 2015, 5, 26491 RSC;
(c) W. Ye, C. Tan, J. Yao, S. Xue, Y. Li and C. Wang, Adv. Synth. Catal., 2016, 358, 426 CrossRef CAS;
(d) S. Xue, J. Liu and C. Wang, Eur. J. Org. Chem., 2016, 2016, 2450 CrossRef CAS.
- Crystallographic data for 3b and 3i have been deposited with the Cambridge Crystallographic Data Centre with the deposition number CCDC 1542637 and 1547900.†.
-
(a) S. Gowrisankar, D. Y. Park and J. N. Kim, Bull. Korean Chem. Soc., 2005, 26, 1826 CrossRef CAS;
(b) D. V. Dorokhov, D. N. Platonov, K. Yu. Suponitsky and Y. V. Tomilov, Russ. Chem. Bull., Int. Ed., 2011, 60, 345 CrossRef CAS.
Footnote |
† Electronic supplementary information (ESI) available: Reactions conditions and spectra. CCDC 1542637 and 1547900. For ESI and crystallographic data in CIF or other electronic format see DOI: 10.1039/c7ra07230a |
|
This journal is © The Royal Society of Chemistry 2017 |
Click here to see how this site uses Cookies. View our privacy policy here.