DOI:
10.1039/C7RA08086G
(Paper)
RSC Adv., 2017,
7, 37739-37742
Photocatalytic direct C–S bond formation: facile access to 3-sulfenylindoles via metal-free C-3 sulfenylation of indoles with thiophenols†
Received
22nd July 2017
, Accepted 25th July 2017
First published on 31st July 2017
Abstract
An efficient and convenient photocatalytic direct C-3 sulfenylation of indoles with thiophenols has been developed for the construction of 3-sulfenylations. This protocol employs thiophenols as the sulfenylating agents, inexpensive rose bengal as the photocatalyst, and shows mild conditions, readily available starting materials, high atom and step atom economy, and environmental advantages. The representative method provides a straightforward approach for C–S bond formation, and has potential applications in the chemical and pharmaceutical industries.
Introduction
3-Substituted indoles compose an important skeleton in natural products, agrochemicals, and functional materials.1 More specifically, 3-sulfenylindoles have been explored for their therapeutic worth in many fields, such as anti-bacterial infection2 and anti-allergy agents.3 Because of the importance of such compounds, numerous synthetic approaches of 3-sulfenylindoles have been successfully established in previous literature.4,5 Importantly, direct functionalization of indoles at the C-3 position is a valuable approach for the effective preparation of 3-sulfenylindoles. So far, a variety of sulfenylating agents such as arylsulfenyl halides,6 arylsulfonyl chlorides,7 sulfonyl hydrazides,8 diaryl disulfides,9 N-thiophthalimides,10 O,S-acetals,11 arylsulfoniumsalts,12 sulfinic acids,13 Bunte salts,14 and thiols15 have also been employed for C3-sulfenylation of indoles. Although many 3-sulfenylindoles can be obtained using these methods, most of the reactions mentioned above suffered from one or more drawback such as stringent conditions (transition metals (VO(acac)2),16a iodine,7c strong base,16b,c high temperature or extreme low temperature16d) and unavailable starting materials (Scheme 1a). Therefore, the development of simple and efficient procedures for the acquisition of 3-sulfenylindoles from easily available starting materials under mild conditions continues to attract the interest of organic chemists because of the remarkable application value of the target products.
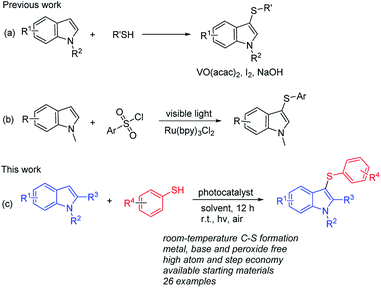 |
| Scheme 1 Synthesis of 3-thioindole with different pairs. | |
For the past several years, more and more chemists gave their attentions to the photocatalytic organic reaction for the synthesis of useful products.17 The metal complexes ([Ru(bpy)3]2+, fac-[Ir(ppy)3]+) and organic dyes (eosin Y, rose bengal) have been explored in photocatalytic radical reaction. Zheng's group successfully developed a visible light mediated C-3 sulfenylation of indole derivatives using [Ru(bpy)3]Cl2 as the photocatalyst (Scheme 1b).7b Herein, we wish to present a simple and efficient synthesis method for the direct sulfenylation of indoles with various thiophenols in the presence of inexpensive and environmentally friendly photocatalyst under air conditions (Scheme 1c). The present protocol provides a convenient and highly attractive approach to a variety of 3-sulfenylindoles in good to excellent yields, and does not require any metal catalyst, base, or peroxide oxidants.
Results and discussion
We started our investigation of light-mediated indole sulfenylation reaction using 1-methyl-1H-indole (1a) and 4-methyl-benzenethiol (2a) as the model substrates in the presence of 1 mol% Ru(phen)3Cl2 in CH3CN. The reaction was carried out open to air, under irradiation with a 5 W compact 415 nm lamp. The desired product 3-((4-methylphenyl)thio)-1-methyl-1H-indole (3aa) was obtained in 47% yield after 12 h (Table 1, entry 1). Inspired by this result, systematic investigation has been conducted to enhance the efficiency of the reaction. Initial attempt on changing photocatalysts was found to be effective to enhance the yield of 3aa, and using rose bengal as the photocatalyst led to significant improvement by giving 3aa with 77% yield (Table 1, entries 2–5). Next, a number of solvents were screened, such as DMF, DMSO, toluene, CH2Cl2 and EtOH (Table 1, entries 6–10). To our delight, 3aa was obtained in 85% yield in CH2Cl2 after 12 h (Table 1, entry 9). No product was formed in the absence of photocatalysts (Table 1, entry 11). Furthermore, no product is produced when additional 1 eq. base was added (Table 1, entry 12). To our delight, 3aa was obtained in 78% yield with 10 W 415 nm lamp after 12 h (Table 1, entry 13). When the reaction was performed on nitrogen or vacuum atmospheres (Table 1, entries 14 and 15), no desired product 3aa was formed. Further control experiment indicated that no reaction took place at all in the absence of light, which clearly showed that photoinduced irradiation was necessary for this transformation (Table 1, entry 16). Gram scale reaction was performed under the optimized reaction conditions to afford the 3aa in 72% isolated yields.
Table 1 Selected optimization studiesa
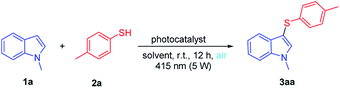
|
Entry |
Photocatalyst |
Solvent |
Yieldb (%) |
Reaction conditions: 1a (0.10 mmol), 2a (0.12 mmol), catalyst (1 mol%), solvent (2.0 mL), open to air under a 5 W 415 nm lamp at room temperature for 12 h. GC yields based on 1a with n-dodecane as an internal standard. Reaction condition was added 1 eq. K2CO3. under 10 W 415 nm lamp promoted. under nitrogen atmosphere. in a vacuum. in the dark. The reaction was performed with 1a (10 mmol), 2a (12 mmol), rose bengal (1 mol%), in 5 mL CH2Cl2, open to air under a 5 W 415 nm lamp at room temperature for 12 h. |
1 |
Ru(phen)3Cl2 |
CH3CN |
47 |
2 |
fac-Ir(ppy)3 |
CH3CN |
35 |
3 |
Eosin B |
CH3CN |
13 |
4 |
Eosin Y |
CH3CN |
11 |
5 |
Rose bengal |
CH3CN |
77 |
6 |
Rose bengal |
DMF |
18 |
7 |
Rose bengal |
DMSO |
Trace |
8 |
Rose bengal |
Toluene |
68 |
9 |
Rose bengal |
CH2Cl2 |
85 |
10 |
Rose bengal |
EtOH |
50 |
11 |
— |
CH2Cl2 |
n.d. |
12c |
Rose bengal |
CH2Cl2 |
n.d. |
13d |
Rose bengal |
CH2Cl2 |
78 |
14e |
Rose bengal |
CH2Cl2 |
n.d. |
15f |
Rose bengal |
CH2Cl2 |
n.d. |
16g |
Rose bengal |
CH2Cl2 |
n.d. |
17h |
Rose bengal |
CH2Cl2 |
72 |
Under the optimized reaction conditions, the scope of the substrates was investigated. Firstly, the reactions of 1-methyl-1H-indole (1a) with a variety of thiophenols (2) were examined and the results were shown in Scheme 2. Thiophenols bearing an electron-donating group or an electron-withdrawing group at p-, m-, o-position reacted smoothly to give the corresponding products in moderate yields 3aa–3aj. However, the electron-withdrawing group on thiophenols afforded lower yields, which attributed to the worse activities of the corresponding thioyl radical intermediates. Moreover, some difunctionalities substituent thiophenols such as 3,4-dimethylbenzenethiol (2k), 2-chloro-4-fluorobenzenethiol (2l), 2,5-dichlorobenzenethiol (2m) were compatible with this reaction leading to the products 3ak–3am, which are the potential substrates for further transformations. Notably, 3,5-bis(trifluoromethyl)benzenethiol (2n) and 2,3,4,5,6-pentafluorobenzenethiol (2o) were suitable for this reaction, with the desired products obtained in moderate yields (3an, 3ao). Moreover, as expected, 2-naphthalenethiol (2p) was also tolerated in this process to afford desired product 3ap obtained in 38% yield.
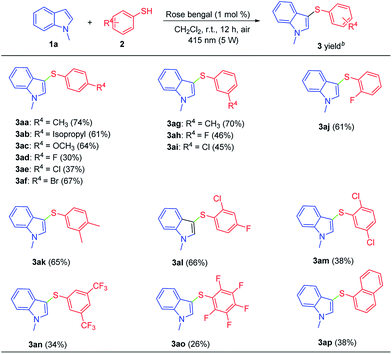 |
| Scheme 2 Substrate scope of thiophenols. aAll reactions were performed with 1a (0.10 mmol), 2 (0.12 mmol), and rose bengal (1 mol%) in 2.0 mL of CH2Cl2, open to air under a 5 W 415 nm lamp at room temperature for 12 h. bIsolated yields (based on 1a). | |
The scope of the indoles substrates is also crucial for the extensive utility of this direct sulfenylation of indoles transformation (Scheme 3). The listed results demonstrate that various indole derivatives (1) reacted smoothly with 4-methylbenzenethiol (2a) to afford the corresponding 3-sulfenylindoles (3) in good yields (Scheme 3). Either electron-withdrawing group or electron-donating group at C-4, C-5, C-6, C-7 position of indoles were reacted with 2a smoothly to give the corresponding products in moderate to good yields 3ba–3ha. However, 4-nitro-1H-indole (1i) failed to react with 2a and no desired product was detected. 5-Bromo-1-methyl-1H-indole (1j) with 2a was examined, providing 3ja in 22% yield. Satisfactorily, 1,2-dimethyl-1H-indole (1k) and 1-methyl-2-phenyl-1H-indole (1l) were also underwent the reaction with 2a to afford the desired products 3ka and 3la in 71% and 52% yields.
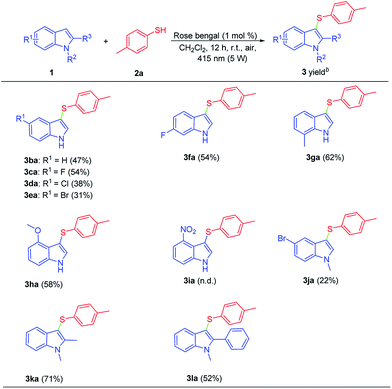 |
| Scheme 3 Substrate scope of indoles. aUnless otherwise noted, all reactions were performed with 1 (0.10 mmol), 2a (0.12 mmol), and rose bengal (1 mol%) in 2.0 mL of CH3CN, open to air under a 5 W 415 nm lamp at room temperature for 12 h. bIsolated yields (based on 1a). | |
Several control experiments were conducted to gain more insight into the mechanism of this unique reaction and the results are presented in Scheme 4. When 3 equivalents of 2,2,6,6-tetramethylpiperidyl-1-oxyl (TEMPO) or 1,1-diphenylethylene (DPE), a radical scavenger, were added to the reaction, no desired product 3aa obtained, however, we detected 1-methyl-3-((2,2,6,6-tetramethylpiperidin-1-yl)oxy)-1H-indole (4), 2,2,6,6-tetramethyl-1-((p-tolylthio)oxy)piperidine (5) and (2,2-diphenylethyl)(4-methylphenyl)sulfane (6) by GC-MS (Scheme 4a and b). Moreover, when 1-methyl-1H-indole reacted with disulfides (7) under standard conditions, the corresponding product 3aa was obtained in 30% yields (Scheme 4c).
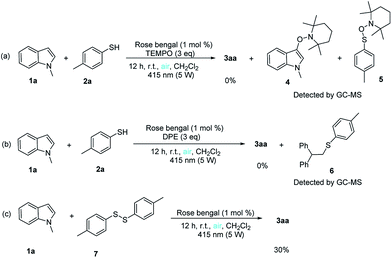 |
| Scheme 4 Mechanistic studies. | |
On the basis of above results, a possible mechanism is proposed in Scheme 5.9b,c First, rose bengal (RB) is excited under light irradiation to produce its excited state species RB*, which interacts with O2 to generate 1O2 via the energy transfer. In this process, the excited state RB* returns to its ground state (RB).18 Subsequently, the generated 1O2 abstracts a hydrogen atom from thiophenol 1 to afford the thioyl radical A. Next, the resulting thioyl radical A interacts with 2 to produce the radical intermediate C. Finally, C is oxidized to the intermediate D, which affords the product 3 via deprotonation. Aerobic oxygen probably plays a crucial role to complete the photoredox cycle by oxidation of the RB radical anion to the ground state (Table 1, entries 14 and 15).18
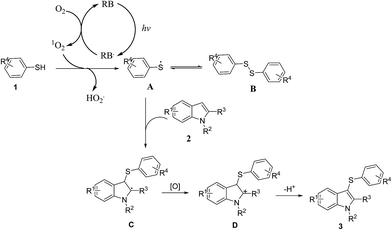 |
| Scheme 5 Proposed mechanism. | |
Conclusions
In conclusion, we have successfully accomplished attractive strategy for direct assembly of 3-sulfenylindoles via photocatalytic direct C-3 sulfenylation of indoles with thiophenols at mild conditions. A variety of indoles and thiophenols have been efficiently transformed to structurally diverse products at room temperature. The reaction uses an inexpensive and readily available organic dye, rose bengal as the photocatalyst and air as the terminal oxidant. Moreover, no heavy-metal waste was generated during the process. It is a practical and environmentally-benign protocol that could prove useful to the chemical and pharmaceutical industries.
Experimental
General information
Melting points were measured using a melting point instrument and are uncorrected. 1H and 13C NMR spectra were recorded on a 400 MHz NMR spectrometer. IR spectra were obtained with an infrared spectrometer on either potassium bromide pellets or liquid films between two potassium bromide pellets. GC-MS data were obtained using electron ionization. HRMS was conducted on a high-resolution mass spectrometer. TLC was performed using commercially available 100–400 mesh silica gel plates (GF254). The photoreaction instrument (WP-TEC-1020L) was purchased from WATTCAS, China. Unless otherwise noted, purchased chemicals were used without further purification.
General procedure for the synthesis of 3-sulfenylindoles derivatives
A mixture of indoles (0.10 mmol), benzenethiols (0.12 mmol), and rose bengal (1 mol%) was stirred in CH2Cl2 (2.0 mL) under a 5 W 415 nm lamp in the photoreaction instrument at room temperature for 12 h. After completion of the reaction (monitored by TLC), water (10 mL) was added to the reaction mixture, and the resulting mixture was extracted with ethyl acetate. The combined organic layers were then dried over MgSO4, filtered, and then concentrated in vacuum. The residue was purified by flash chromatography on silica gel to give the desired product (using the mixture of petroleum ether and ethyl acetate as eluents).
Acknowledgements
We are grateful to the National Natural Science Foundation of China (21602031 and 21662002), the Advanced Innovation Research Teams Project of Jiangxi Province (20133BCB24011), and the Jiangxi Natural Science Foundation (20171BAB203010, 20141BBG70070 and 20151BAB203011).
Notes and references
- For reviews, see:
(a) C. D. Funk, Nat. Rev. Drug Discovery, 2005, 4, 664–672 CrossRef CAS PubMed;
(b) R. Ragno, A. Coluccia, G. La Regina, G. De Martino, F. Piscitelli, A. Lavecchia, E. Novellino, A. Bergamini, C. Ciaprini, A. Sinistro, G. Maga, E. Crespan, M. Artico and R. Silvestri, J. Med. Chem., 2006, 49, 3172–3184 CrossRef CAS PubMed;
(c) G. La Regina, M. C. Edler, A. Brancale, S. Kandil, A. Coluccia, F. Piscitelli, E. Hamel, G. De Martino, R. Matesanz, J. F. Diaz, A. I. Scovassi, E. Prosperi, A. Lavecchia, E. Novellino, M. Artico and R. Silvestri, J. Med. Chem., 2007, 50, 2865–2874 CrossRef CAS PubMed.
- S. S. Khandekar, D. R. Gentry, G. S. Van Aler, P. Warren, H. Xiang, C. Silverman, M. L. Doyle, P. A. Chambers, A. K. Konstantinidis, M. Brandt, R. A. Daines and J. T. Lonsdale, J. Biol. Chem., 2001, 276, 30024–30030 CrossRef CAS PubMed.
- P. C. Unangst, D. T. Connor, S. R. Stabler, R. J. Weikert, M. E. Carethers, J. A. Kennedy, D. O. Thueson, J. C. Chestnut, R. L. Adolphson and M. C. Conroy, J. Med. Chem., 1989, 32, 1360–1366 CrossRef CAS PubMed.
- J. Li, C. Li, S. Yang, Y. An, W. Wu and H. Jiang, J. Org. Chem., 2016, 81, 7771–7783 CrossRef CAS PubMed.
- J. Li, C. Li, S. Yang, Y. An, W. Wu and H. Jiang, J. Org. Chem., 2016, 81, 2875–2887 CrossRef CAS PubMed.
- P. Hamel and P. Préville, J. Org. Chem., 1996, 61, 1573–1577 CrossRef CAS PubMed.
- For reviews, see:
(a) Q. Wu, D. Zhao, X. Qin, J. Lan and J. You, Chem. Commun., 2011, 47, 9188–9190 RSC;
(b) M. Chen, Z.-T. Huang and Q.-Y. Zheng, Chem. Commun., 2012, 48, 11686–11688 RSC;
(c) G. Kumaraswamy, R. Raju and V. Narayanarao, RSC Adv., 2015, 5, 22718–22723 RSC.
- X. Li, Y. Xu, W. Wu, C. Jiang, C. Qi and H. Jiang, Chem.–Eur. J., 2014, 20, 7911–7915 CrossRef CAS PubMed.
- For reviews, see:
(a) C. C. Browder, M. O. Mitchell, R. L. Smith and G. el-Sylayman, Tetrahedron Lett., 1993, 34, 6245–6246 CrossRef CAS;
(b) W. Ge and Y. Wei, Green Chem., 2012, 14, 2066–2070 RSC;
(c) P. Sang, Z. Chen, J. Zou and Y. Zhang, Green Chem., 2013, 15, 2096–2100 RSC;
(d) X. Zhou and X. Li, RSC Adv., 2014, 4, 1241–1245 RSC.
- T. Hostier, V. Ferey, G. Ricci, D. G. Pardo and J. Cossy, Chem. Commun., 2015, 51, 13898–13901 RSC.
- M. Matsugi, K. Gotanda, K. Murata and Y. Kita, Chem. Commun., 1997, 15, 1387–1388 RSC.
- H. Rao, P. Wang, J. Wang, Z. Li, X. Sun and S. Cao, RSC Adv., 2014, 4, 49165–49169 RSC.
- C.-R. Liu and L.-H. Ding, Org. Biomol. Chem., 2015, 13, 2251–2254 CAS.
- H. Qi, T. Zhang, K. Wan and M. Luo, J. Org. Chem., 2016, 81, 4262–4268 CrossRef CAS PubMed.
- X. Liu, H. Cui, D. Yang, S. Dai, G. Zhang, W. Wei and H. Wang, Catal. Lett., 2016, 146, 1743–1748 CrossRef CAS.
- For reviews, see:
(a) Y. Maeda, M. Koyabu, T. Nishimura and S. Uemura, J. Org. Chem., 2004, 69, 7688–7693 CrossRef CAS PubMed;
(b) Y. Liu, Y. Zhang, C. Hu, J.-P. Wan and C. Wen, RSC Adv., 2014, 4, 35528–35530 RSC;
(c) X. Zhang, X. Zhou, H. Xiao and X. Li, RSC Adv., 2013, 3, 22280–22284 RSC;
(d) K. M. Schlosser, A. P. Krasutsky, H. W. Hamilton, J. E. Reed and K. Sexton, Org. Lett., 2004, 5, 819–821 CrossRef PubMed.
- For reviews, see:
(a) J.-R. Chen, X.-Q. Hu, L.-Q. Lu and W.-J. Xiao, Chem. Soc. Rev., 2016, 45, 2044–2056 RSC;
(b) J.-R. Chen, X.-Q. Hu, L.-Q. Lu and W.-J. Xiao, Acc. Chem. Res., 2016, 49, 1911–1923 CrossRef CAS PubMed;
(c) J. C. Tellis, C. B. Kelly, D. N. Primer, M. Jouffroy, N. R. Patel and G. A. Molander, Acc. Chem. Res., 2016, 49, 1429–1439 CrossRef CAS PubMed;
(d) I. Ghosh, L. Marzo, R. Shaikh and B. König, Acc. Chem. Res., 2016, 49, 1566–1577 CrossRef CAS PubMed;
(e) K. Nakajima, Y. Miyake and Y. Nishibayashi, Acc. Chem. Res., 2016, 49, 1946–1956 CrossRef CAS PubMed;
(f) S. A. Morris, J. Wang and N. Zheng, Acc. Chem. Res., 2016, 49, 1957–1968 CrossRef CAS PubMed;
(g) T. Koike and M. Akita, Acc. Chem. Res., 2016, 49, 1937–1945 CrossRef CAS PubMed;
(h) K. A. Margrey and D. A. Nicewicz, Acc. Chem. Res., 2016, 49, 1997–2006 CrossRef CAS PubMed;
(i) Y.-Y. Liu, X.-Y. Yu, J.-R. Chen, M.-M. Qiao, X. Qi, D.-Q. Shi and W.-J. Xiao, Angew. Chem., Int. Ed., 2017, 56, 9527–9531 CrossRef CAS PubMed;
(j) U. K. Sharma, H. P. L. Gemoets, F. Schröder, T. Noël and E. V. van der Eycken, ACS Catal., 2017, 7, 3818–3823 CrossRef CAS;
(k) W.-J. Xiao, J.-R. Chen, D.-M. Yan and Q. Wei, ChemPhotoChem, 2017, 1, 148–158 CrossRef.
-
(a) Q. Shi, P. Li, Y. Zhang and L. Wang, Org. Chem. Front., 2017, 4, 1322–1330 RSC;
(b) W. Fan, Q. Yang, F. Xu and P. Li, J. Org. Chem., 2014, 79, 10588–10592 CrossRef CAS PubMed.
Footnotes |
† Electronic supplementary information (ESI) available. See DOI: 10.1039/c7ra08086g |
‡ W. G. and W. T. contributed equally. |
|
This journal is © The Royal Society of Chemistry 2017 |
Click here to see how this site uses Cookies. View our privacy policy here.