DOI:
10.1039/C7RA08482J
(Paper)
RSC Adv., 2017,
7, 44366-44370
Copper-catalyzed selective C–N bond formation with 2-amino, 2-hydroxy and 2-bromo-5-halopyridine†
Received
1st August 2017
, Accepted 31st August 2017
First published on 15th September 2017
Abstract
A copper-catalyzed 1,2-diol amination at the electron-rich C-5 position of unprotected 2-amino/2-hydroxy-5-halopyridine provided excellent yields. Selective amination preferably at C-5 in 2-bromo-5-iodopyridine was achieved under the same conditions. The selective, generally mild and economical coupling reaction at C-5 position described herein could be achieved with amines, heterocycles and amides.
Introduction
Numerous pyridine-derived compounds, isolated from natural sources, possess a broad spectrum of therapeutic activity, such as antibacterial, antiviral, anticancer, antifungal as well as anti-inflammatory.1,2 Substituted aminopyridines could be used as a synthetic handle to prepare various heterocyclic cores, such as pyridopyrimidine, imidazopyridine, triazolopyridine and quinazoline, which are of great significance for modifying the biological function of various, already known, drug-like molecules (Fig. 1).3 The formation of C–N bonds has been of great interest as a method to introduce nitrogen and subsequently derivatize molecules. Ever since the first report of the introduction of C–N bonds by Ullmann and Goldberg,4 there has been substantial improvement upon the reported process through usage of metal complexes comprising various ligands. The efficient N-arylation of aryl halides catalyzed by palladium (Pd) and copper (Cu) has been developed independently by Buchwald and Hartwig using suitable diamine or phosphine ligands.5,6 However, there are still limitations to the existing methods. Most of the studies related to C–N bond formation have been reported on halobenzene or substituted halobenzene.7,8 The selective and efficient synthesis of aminopyridines containing unprotected amino, hydroxyl or bromo groups at the C-2 position is still challenging, thus limiting its scope for various substrates.9,10
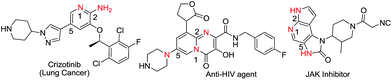 |
| Fig. 1 Biologically active agents containing the 2,5-disubstituted pyridine moiety. | |
2-Amino-, 2-hydroxy- or 2-bromo-5-iodopyridine offers an interesting model to study C–N bond formation. The C-5 position of 2-aminopyridine is more electron-rich, thus C–X bond polarization is not sufficient to attain the usual SNAr reaction. At the C-2 position, the compounds have an unprotected –NH2/–OH or bromo group, which makes C–N bond formation at the C-5 position very challenging. In the literature, there are reports of such transformations where the –NH2 group was first alkylated11 or protected with a Boc group and after obtaining the low yielding C–N bond formation the protection group was subsequently deprotected.12 For the transformation of 2-hydroxypyridine derivatives, either the hydroxyl group or the nitrogen of pyridine was first protected with a benzyl group and subsequently, at a later stage, a deprotection step was carried out.13 Protection–deprotection chemistry not only increases the number of steps but also generates undesirable by-products. Selective amination at the C-5 position of 2,5-dihalopyridine is still regarded as a non-trivial synthetic challenge. We have established that by changing the reactivity of the carbon–halo bond at C-5, selective amination could be achieved at either the C-2 or C-5 position of 2,5-dihalopyridine. Herein, we report a systematic study of Pd- and Cu-catalyzed 1,2-diol aryl amination at the C-5 position of 2-amino-5-halopyridine and thereafter extended the study to 2-hydroxy-5-halopyridine and 2-bromo-5-iodopyridine to develop a highly selective, operationally simple, high yielding and economically attractive method. This approach has several advantages as it not only eliminates the use of a phosphine ligand and expensive organic reagents but also unnecessary derivatization and its subsequent generation of waste by-products.
Results and discussion
The present study is based on our on-going research towards the development of efficient scalable methodology used for library synthesis of various privileged scaffolds containing substituted aminopyridines. We aimed to study Pd- or Cu-catalyzed reactions to form C–N bond in these systems.14 To check the efficiency of various Pd-catalysts, ligands and bases in the amination reaction at the C-5 position of 2-aminopyridine, a prototype reaction was set-up using 2-amino-5-iodopyridine and morpholine with Pd2(dba)3 (10 mol%) used as the catalyst and BINAP (20 mol%) as the ligand in THF/toluene at 110 °C. The reaction did not proceed at all even after 24 h. Furthermore, changing from conventional heating to microwave conditions did not yield the expected product either. Next, we screened the effect of bulky monodentate/bidentate phosphine ligands and bulky bases. The reaction proceeded with high yield only when LiHMDS was used as the base along with Pd2(dba)3 and XPhos used as the catalyst and ligand, respectively.10 We tried to change the catalyst from Pd2(dba)3 to Pd(dppf)Cl2 or Pd(OAc)2 keeping the other conditions unchanged, but the reaction either did not proceed or yielded the product in trace amount. The reaction with other bases such as NaOtBu, Cs2CO3 or K2CO3, in place of LiHMDS, did not proceed barring a few cases that yielded the product in trace amounts. The use of LiHMDS with Pd2(dba)3 seems to be the pivotal factor in the Pd-catalyzed C–N bond formation (Table 1). The disadvantages associated with the above Buchwald conditions depict the highly specific usage of a bulky base along with a definite catalyst–ligand combination for the reaction to proceed in high yield. The oxophilicity associated with phosphine ligand, high cost as well as air sensitivity of palladium catalysts and operational difficulty associated with LiHMDS prompted us to explore the Cu-catalyzed Ullmann reaction conditions (Table 2).
Table 1 Optimization of 2-amino-5-iodopyridine using Pd2(dba)3
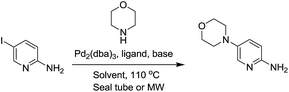
|
Entry |
Ligand (15 mol%) |
Base |
Solvent |
Yielda |
Isolated yield. Microwave (50 W) at 100 °C in toluene for 1 h. K2CO3 and Cs2CO3 was used instead of NatOBu. The reaction was performed on a 0.5 mmol scale, 3.0 equiv. of base, 10 mol% Pd2(dba)3 and 20–30 mol% ligand. |
1 |
BINAP |
NatOBu |
THFb |
No productc |
2 |
XantPhos |
NatOBu |
THF |
No product |
3 |
DavePhos |
NatOBu |
THF |
No product |
4 |
XPhos |
LiHMDS |
THF |
78% |
5 |
XPhos |
NatOBu |
THFb |
Trace |
6 |
XPhos |
NatOBu/LiBr |
THF |
<10% |
7 |
XantPhos |
LiHMDS |
THF |
28% |
8 |
BINAP |
LiHMDS |
THF |
No product |
9 |
DavePhos |
LiHMDS |
THF |
No product |
Table 2 Optimization of reaction conditions used to couple 2-amino-5-iodopyridine with morpholine
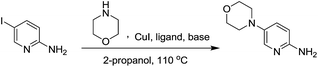
|
Entry |
Ligand (20 mol%) |
Base |
Time |
Yielda |
Isolated yield. t-BuOH as solvent showed same yield. Reaction performed in 0.5 mmol scale, 10 mol% CuI, 3.0 equiv. of base, 10 mol% ligand in seal tube. |
1 |
Ethylene glycol |
K3PO4 |
10 h |
87%b |
2 |
Ethylene glycol |
K2CO3 |
12 h |
80% |
3 |
Ethylene glycol |
Cs2CO3 |
12 h |
85% |
4 |
cis-1,2-Cyclohexanediol |
K3PO4 |
36 h |
74% |
5 |
trans-1,2-Cyclohexanediol |
K3PO4 |
36 h |
72% |
6 |
2-Methoxyethanol |
K3PO4 |
48 h |
63% |
7 |
1,3-Propandiol |
K3PO4 |
48 h |
No product |
8 |
Glucose |
K3PO4 |
24 h |
No product |
9 |
Ethylene diamine |
K3PO4 |
24 h |
No product |
10 |
N,N-Dimethylethane-1,2-diamine |
K3PO4 |
24 h |
No product |
11 |
L-Proline |
K3PO4 |
48 h |
55% |
12 |
4-Hydro-L-proline |
K3PO4 |
48h |
Trace |
13 |
N-Hydroxy succinimide |
K3PO4 |
48 h |
No product |
14 |
No ligand |
K3PO4 |
48 h |
No product |
We initiated our study using inexpensive CuI as the catalyst. We decided to evaluate the effect of N,N-bidentate, O,O-bidentate and N,O-bidentate ligands. The C–N bond formation was favored with 1,2-diol ligands such as ethylene glycol, cis-1,2-cyclohexanediol and trans-1,2-cyclohexanediol. Although cis-1,2-cyclohexanediol and trans-1,2-cyclohexanediol provided good yields, the time taken for the completion of the reactions was 36 h. It is worth mentioning that 2-methoxyethanol, where one of the hydroxyl groups of ethylene glycol is protected with a methyl group, provided the expected product with 63% yield along with the recovery of starting material after 48 h. The reaction proceeded efficiently using CuI as the catalyst and ethylene glycol as the ligand in t-butanol or 2-propanol at 110 °C with an 87% isolated yield after 10 h. The use of inexpensive and readily available ethylene glycol is pivotal as propylene glycol, which is a 1,3-diol, failed to provide any product. Furthermore, no product was observed in the absence of ethylene glycol. Thus, we could assume that the 1,2-diol ligand plays an important role in accelerating the rate of the Cu-salt catalyzed C–N bond formation reaction when compared to 1,3-diol. This could be attributed to ethylene glycol being more effective in stabilizing the copper complex. Interestingly, among all the other ligands used, only L-proline gave 55% yield of the expected product. The reactions with 2-amino-5-bromopyridine and various amines yielded ∼10% less products (Table 4).
Next, we intended to establish a generalized protocol for C–N bond formation using both Cu(I) and Cu(II) salts (Table 3). Accordingly we studied the role of CuI, CuBr2, Cu2O, CuO and Cu(OAc)2. Interestingly, the reaction could be performed readily with both oxidation states of the copper salts with high yields. With respect to the reaction yield and reaction time, Cu(I) was found to be the best choice, while the other copper salts gave lower yields with longer reaction times.
Table 3 The effect of the Cu(I) and Cu(II) catalysts on the coupling of 2-amino-5-iodopyridine with morpholine
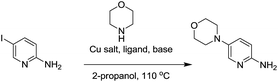
|
Entry |
Cu salt |
Time |
Yielda |
Isolated yield. 10 mol% CuI, 3.0 equiv. of base, 10 mol% ligand in sealed tube. |
1 |
CuI |
10 h |
87% |
2 |
Cu2O |
36 h |
74% |
3 |
CuO |
36 h |
70% |
4 |
CuBr |
14 h |
82% |
5 |
Cu(OAc)2 |
24 h |
72% |
To explore the generality of the method, a wide variety of substrates were subjected to the standardized Ullmann reaction conditions using 2-amino-5-iodopyridine and 2-hydroxy-5-iodopyridine (Table 4). The substrates include primary amines, aliphatic cyclic amines, heterocycles, aliphatic amides, cyclic amides and aromatic amides. The reaction with aliphatic cyclic amines (entries 1–3, 12, 13) provided the expected product in excellent yield. It is noteworthy to mention that the reaction conditions could be tolerated by the susceptible-Boc group (entry 3, 13). The reaction was performed on 5.0 g scale with 85% yield. The reaction with primary amines, containing benzylic amines (entry 4, 14) and cycloalkyl amine (entry 5), readily yielded the product in 72%, 78% and 86% respectively. N-Aryl imidazole,15 N-arylindole16 and N-arylamide17 motifs are prevalent structural elements present in numerous natural products, drugs and energetic materials. The reaction with different heterocycles such as imidazole (entry 6, 15), pyrazole (entry 7) and indole (entry 8, 16) efficiently yielded the expected amination reaction at the C-5 position. The reaction with indole provided exclusively the N-aryl product and no traces of the C-aryl product were obtained.18 The reaction with aliphatic amide (entry 9), cyclic amides (entry 10, 17) and aromatic amide (entry 11) also provided the expected products. The amination reaction between 2-amino-5-iodopyridine and 2-hydroxy-5-iodopyridine provided comparable chemical yields. We inserted differential protecting groups (entry 3, 13) to depict the robustness of the methodology and for further strategic manipulation and derivatization.
Table 4 The reaction of 2-amino/hydroxy-5-iodopyridine with amines, amides and heterocycles
Encouraged by these results, we further explored the selective nature of the protocol in dihalopyridines. It has been reported that 2-5-dibromopyridine exclusively delivers amination at the C-2 position via Ullmann reaction conditions.19 On the contrary, we have found that on changing the reactivity of the carbon–halo bond, the amination product could be reversed. 2-Bromo-5-iodopyridine, when subjected to the standardized reaction conditions (Table 5) with aliphatic amines (entry 1, 2), heterocycles (entry 3, 4) and amides (entry 5), provided amination at the C-5 position with excellent chemoselectivity and high chemical yield. In case of 2-bromo-5-iodopyridine, the amination occurs at the more reactive C–I bond resulting in selective amination at C-5 position. We also extended the methodology to the synthesis of privileged scaffolds, such as imidazopyridine16 and pyridopyrimidine17 as shown in Scheme 1.
Table 5 The substrate scope of the reaction of 2-bromo-5-iodopyridine with amines, amide and heterocycles
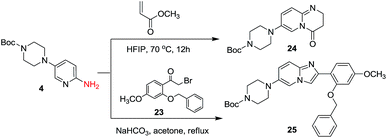 |
| Scheme 1 Application in the synthesis of biologically active cores. | |
Conclusions
In summary, we have achieved a copper-catalyzed ethylene glycol promoted amination of 2-amino/2-hydroxy/2-bromo-5-halopyridine containing unprotected functional groups. The robustness of the protocol was evident from the fact that various primary amines, aliphatic cyclic amines, heterocycles, and amides were coupled with excellent yield and selectivity. We have established that selective amination preferably at the C-5 position could be achieved in 2,5-dihalopyridines. On changing the reactivity of the carbon–halo bond at the C-5 position, the amination product could be reversed. This operationally simple and economically attractive protection free protocol not only reduces the number of steps and undesirable by-products but also eliminates the use of phosphine ligands and expensive Pd catalysts. The protocol was applied to the synthesis of privileged scaffolds such as imidazopyridine and pyridopyrimidine.
Conflicts of interest
There are no conflicts to declare.
Acknowledgements
S. R., B. P., A. M. and B. K. would like to acknowledge the UGC for fellowship. The research was supported by the DST-SERB (Grant No. EMR/2015/000117) to A. T. We thank Dr Ramalingam Natarajan, Senior Scientist CSIR-Indian Institute of Chemical Biology for his suggestions.
Notes and references
-
(a) S. H. Cho, J. Y. Kim, J. Kwak and S. Chang, Chem. Soc. Rev., 2011, 40, 5068 RSC;
(b) M. S. Butler, J. Nat. Prod., 2004, 67, 2141 CrossRef CAS PubMed;
(c) A. F. Pozharskii, A. T. Sodartenko and A. Katritzky, Heterocycles in Life and Society, Wiley, New York, 1997 Search PubMed.
-
(a) J. J. Cui, M. Tran-Dubé, H. Shen, M. Nambu, P. P. Kung, M. Pairish, L. Jia, J. Meng, L. Funk, I. Botrous, M. McTigue, N. Grodsky, K. Ryan, E. Padrique, G. Alton, S. Timofeevski, S. Yamazaki, Q. Li, H. Zou, J. Christensen, B. Mroczkowski, S. Bender, R. S. Kania and M. P. Edwards, J. Med. Chem., 2011, 54, 6342 CrossRef CAS PubMed;
(b) F. I. Carroll, F. Liang, H. A. Navarro, L. E. Brieaddy, P. Abraham, M. I. Damaj and B. R. Martin, J. Med. Chem., 2001, 44, 2229 CrossRef CAS PubMed;
(c) H. Yamagishi, S. Shirakami, Y. Nakajima, A. Tanaka, F. Takahashi, H. Hamaguchi, K. Hatanaka, A. Moritomo, M. Inami, Y. Higashi and T. Inoue, Bioorg. Med. Chem., 2015, 23, 4846 CrossRef CAS PubMed;
(d) J. W. Daly, T. F. Spande and H. M. Garraffo, J. Nat. Prod., 2005, 68, 1556 CrossRef CAS PubMed.
-
(a) J. L. Bolliger, M. Oberholzer and C. M. Frech, Adv. Synth. Catal., 2011, 353, 945 CrossRef CAS;
(b) P. Lechat, S. Tesleff and W. C. Bownan, Aminopyridines and Similarly Acting Drugs, Oxford, Pergamon, 1982 Search PubMed;
(c) J. Zhang, H. I. Pettersson, C. Huitema, C. Niu, J. Yin, M. N. G. James, L. D. Eltis and J. C. Vederas, J. Med. Chem., 2007, 50, 1850 CrossRef CAS PubMed;
(d) S. Bhargava and A. Choudhary, Int. J. Pharm. Pharm. Sci., 2014, 6, 553 Search PubMed;
(e) V. Summa, O. Kinzel and M. Donghi, US 2009/0221571 A1, 2009..
-
(a) F. Ullmann, Ber. Dtsch. Chem. Ges., 1903, 36, 2382 CrossRef;
(b) I. Goldberg, Ber. Dtsch. Chem. Ges., 1906, 39, 1691 CrossRef.
- J. F. Hartwig, Acc. Chem. Res., 2008, 41, 1534 CrossRef CAS PubMed.
- D. S. Surry and S. L. Buchwald, Chem. Sci., 2011, 2, 27 RSC.
- F. Y. Kwong, A. Klapars and S. L. Buchwald, Org. Lett., 2002, 4, 581 CrossRef CAS PubMed.
- J. Bariwal and E. Van der Eycken, Chem. Soc. Rev., 2013, 42, 9283 RSC.
- D. Maiti, B. P. Fors, J. L. Henderson, Y. Nakamura and S. L. Buchwald, Chem. Sci., 2011, 2, 57 RSC.
- K. W. Anderson, R. E. Tundel, T. Ikawa, R. A. Altman and S. L. Buchwald, Angew. Chem., Int. Ed., 2006, 45, 6523 CrossRef CAS PubMed.
-
(a) J. J. Hanthorn, L. Valgimigli and D. A. Pratt, J. Org. Chem., 2012, 77, 6908 CrossRef CAS PubMed;
(b) M. Reich, S. Schunk, J. Stolberg, V. Herentals, K. Aachen, G. Aachen and E. Turnhout, U.S. 20120071461, 2012.
- N. G. Cooke, G. Fernandes, S. P. Dos, N. Graveleau, C. Hebach, K. Hoegenauer, G. Hollingworth, A. B. Smith, N. Soldermann, F. Stowasser, R. Strang, N. Tufilli, A. Von Matt, R. Wolf and F. Zecri, WO 2012004299 A1, 2012.
-
(a) J. N. Heo, Y. S. Song and B. T. Kim, Tetrahedron Lett., 2005, 46, 4621 CrossRef CAS;
(b) N. C. Becknell, J. A. Lyons, L. D. Aimone, J. A. Gruner, J. R. Mathiasen, R. Raddatz and R. L. Hudkins, Bioorg. Med. Chem. Lett., 2011, 21, 7076 CrossRef CAS PubMed.
-
(a) J. Bariwal and E. V. Eycken, Chem. Soc. Rev., 2013, 42, 9283–9303 RSC;
(b) H. Lin and D. Sun, Org. Prep. Proced. Int., 2013, 45, 341 CrossRef CAS PubMed;
(c) E. V. Beletskiy, C. h. Sudheer and C. J. Douglas, J. Org. Chem., 2012, 77, 5884 CrossRef CAS PubMed;
(d) L. Zhu, P. Guo, G. Li, J. Lan, R. Xie and J. You, J. Org. Chem., 2007, 72, 8535 CrossRef CAS PubMed;
(e) K. J. Filipski, J. T. Kohrt, A. Casimiro-Garcia, C. A. Van Huis, D. A. Dudley, W. L. Cody, C. F. Bigge, S. Desiraju, S. Sun, S. N. Maiti, M. R. Jaber and J. J. Edmunds, Tetrahedron Lett., 2006, 47, 7677 CrossRef CAS.
-
(a) Z. Jin, Nat. Prod. Rep., 2005, 22, 196 RSC;
(b) L. D. Luca, Curr. Med. Chem., 2006, 13, 1 Search PubMed;
(c) H. Gao and J. M. Shreeve, Chem. Rev., 2011, 111, 7377 CrossRef CAS PubMed;
(d) Y. Liu, Q. Zhang, X. Ma, P. Liu, J. Xie, B. Dai and Z. Liu, Int. J. Org. Chem., 2013, 3, 185 CrossRef.
-
(a) M. A. Alam, Z. Alsharif, H. Alkhattabi, D. Jones, E. Delancey, A. Gottsponer and T. Yang, Sci. Rep., 2016, 6, 36316, DOI:10.1038/srep36316;
(b) N. K. Kaushik, N. Kaushik, P. Attri, N. Kumar, C. H. Kim, A. K. Verma and E. H. Choi, Molecules, 2013, 18, 6620 CrossRef CAS PubMed;
(c) K. Andersen, T. Liljefors, J. Hyttel and J. Perregaard, J. Med. Chem., 1996, 39, 3723 CrossRef CAS PubMed;
(d) J. C. Antilla, A. Klapars and S. L. Buchwald, J. Am. Chem. Soc., 2002, 124, 11684 CrossRef CAS PubMed;
(e) R. K. Rao, A. B. Naidu, E. A. Jaseer and G. Sekar, Tetrahedron, 2009, 65, 4619 CrossRef CAS.
-
(a) C. Wang, L. Liu, W. Wang, D.-S. Ma and H. Zhang, Molecules, 2010, 15, 1154 CrossRef CAS PubMed;
(b) P. N. Craig, Comprehensive Medicinal Chemistry, ed. C. J. Drayton, Pergamon Press, New York, NY, USA, 1991, vol. 8 Search PubMed.
- D. W. Old, M. C. Harris and S. L. Buchwald, Org. Lett., 2000, 2, 1403 CrossRef CAS PubMed.
-
(a) H. Zhang, Q. Cai and D. Ma, J. Org. Chem., 2005, 70, 5164–5173 CrossRef CAS PubMed;
(b) J. Ji, T. Li and W. H. Bunnelle, Org. Lett., 2003, 5, 4611 CrossRef CAS PubMed.
Footnotes |
† Electronic supplementary information (ESI) available. See DOI: 10.1039/c7ra08482j |
‡ Swarnali Roy and Barnali Paul contributed equally. |
|
This journal is © The Royal Society of Chemistry 2017 |
Click here to see how this site uses Cookies. View our privacy policy here.