DOI:
10.1039/C7RA08593A
(Paper)
RSC Adv., 2017,
7, 47091-47098
Preparation, characterization and anticancer activities of resveratrol loaded redox-sensitive F127-SS-TOC micelles†
Received
3rd August 2017
, Accepted 1st October 2017
First published on 6th October 2017
Abstract
Resveratrol (RES), a natural polyphenolic compound existing in plants, exhibits anticancer potential in inhibiting growth of various types of human cancers especially breast cancer. However, the poor aqueous solubility and low bioavailability limit its clinical application. Pluronic F127 (F127)-SS-Tocopherol (TOC) polymer is a redox-sensitive conjugate which has been synthesized by our previous study. Due to the amphiphilic properties of block copolymer F127-SS-TOC, RES was encapsulated by the F127-SS-TOC polymer and formed RES-loaded micelles (F127-SS-TOC/RES) in aqueous solution by self-assembly via a solvent evaporation method. The drug loading of F127-SS-TOC/RES micelles was 13.25 ± 2.29%, which remained stable for 7 days at 25 °C. The particle size and zeta potential of the above-mentioned micelles measured by dynamic light scattering (DLS) were 39.50 ± 1.39 nm and −4.55 ± 0.39 mV, respectively. Besides, F127-SS-TOC/RES micelles have significant cytotoxicity in human breast cancer cells MCF-7 and MDA-MB-231 compared to free RES and non-redox-sensitive F127-TOC/RES micelles. The results also showed that redox-sensitive F127-SS-TOC/RES micelles could induce apoptosis and elevate the intracellular reactive oxygen species (ROS) level against MDA-MB-231 cells. Hence, our results indicated that RES loaded F127-SS-TOC micelles hold potential in improving the treatment of breast cancer.
1 Introduction
Breast cancer is one of the common malignant diseases with a high morbidity rate worldwide in women.1,2 Surgery, chemotherapy and radiotherapy are the major and common treatments for breast cancer, occasionally, some side effects may occur without being expected, such as postoperative trauma, gastro-intestinal reactions, alopecia, etc. An increasing number of drug delivery systems (DDS) have attracted more attention for improving therapeutics via designing multifunctional drug vehicles including polymeric micelles,3 nanoparticles,4 liposomes,5 nanogels,6 et al. Polymeric micelles can encapsulate poorly water-soluble drugs with a narrow size distribution, high drug loading capacity and the ability of extending systemic circulation.7,8 Moreover, the polymeric micelles are amphiphilic block copolymer and formed the micelles structure with hydrophobic inner core and hydrophilic outer shell self-assembling in water.9–12 Due to enhanced permeability and retention (EPR) effect, drug loaded micelles can passively target to the tumor site and release drug.13,14 When the drug loaded micelles enter into cells, the fast drug release is expected to play the therapeutic effect. Nowadays, redox-sensitive micelles, one of stimuli-responsive drug release systems, has been designed to achieve rapid drug release in cancer cells, which is triggered by the higher intracellular concentration of glutathione (GSH) (about 2–10 mM).15,16 In our previous study, we synthesized a redox-sensitive Pluronic F127-SS-α-tocopherol (TOC) polymer using a disulfide bond to link F127 and TOC, which might cleave in response to reductive environment of intracellular GSH in cancer cells.17
Resveratrol (RES), a natural antioxidant polyphenolic compound, is found in a variety of plants, including grapes, berriers, peanuts, the roots of Polygonum, et al.18–20 Besides, it was reported that due to the structure similarity between RES and estrogenic agent diethylstilbestrol, RES could be a phytoestrogen.1 RES, the main ingredient of red wine, had potential in inhibiting the growth of different types of cancer without obvious side effects. The anticancer mechanism of this natural compound might associate with modulating different signaling pathways involved in cell inflammation, proliferation, apoptosis, invasion, metastasis and angiogenesis.20,21 It was reported that RES induced cell apoptosis via the activation of mitogen-activated protein kinases (MAPKs)22 and extracellular regulated protein kinases (ERKs).21 Nevertheless, the challenges of RES needed to be faced before the clinical use, especially the poor aqueous solubility (3 mg/100 mL), low bioavailability, photo-labile property, etc.8,23 In this study, F127-SS-TOC micelles system was investigated to deliver RES against breast cancer cells (MCF-7 and MDA-MB-231) for the first time. Firstly, the RES loaded micelles (F127-SS-TOC/RES) were formed by solvent evaporation method. Secondly, the characterizations of F127-SS-TOC/RES micelles were evaluated through the tests of particle sizes, zeta potential, drug loading, transmission electron microscopy (TEM), drug release profile and stability. Thirdly, the biocompatibility and cytotoxicity of F127-SS-TOC/RES micelles were evaluated by 3-(4,5-dimethyl-2-thiazolyl)-2,5-diphenyl tetrazolium bromide (MTT) assay. Furthermore, the cell apoptosis and intracellular reactive oxygen species (ROS) generation were also measured in this study. We found F127-SS-TOC polymer is a promising carrier to deliver RES against breast cancer.
2 Materials and methods
2.1 Materials
F127-SS-TOC polymers were synthesized and the synthetic method was described previously.17 Dithiothreitol (DTT) was purchased from Sigma-Aldrich (St. Louis, MO, USA). Resveratrol (RES) was obtained from Aladdin. Millipore water was prepared with a Milli-Q Plus System (Merck Millipore Co., Billerica, MA, USA). All chemicals were in analytical grade.
Dulbecco's Modified Eagle's Medium (DMEM), FBS, phosphate-buffered saline (PBS), penicillin–streptomycin, and 0.25% (w/v) trypsin/1 mM EDTA were obtained from Thermo Fisher Scientific (Waltham, MA, USA). 3-[4,5-Dimethyl-2-thiazolyl]-2,5-diphenyl tetrazolium bromide (MTT) was purchased from Sigma-Aldrich (St Louis, MO, USA). 2′,7′-Dichlorodihydrofluorescein diacetate (DCFH-DA), Annexin V-FITC and propidium iodide (PI) were purchased from Nanjing Jiancheng Bioengineering Institute.
2.2 Cell culture
Human breast cancer cell lines MCF-7 and MDA-MB-231 were acquired from the American Type Culture Collection (ATCC). Both MCF-7 and MDA-MB-231 cells were cultured in DMEM medium with antibiotics (100 U mL−1 penicillin, 100 μg mL−1) streptomycin and 10% heat-inactivated fetal bovine serum (FBS, v/v) under 5% CO2 at 37 °C.
2.3 Preparation of F127-SS-TOC/RES micelles and F127-TOC/RES micelles
RES loaded F127-SS-TOC (F127-SS-TOC/RES) micelles were prepared using the solvent evaporation method as reported previously.17 Briefly, 10 mg F127-SS-TOC polymer and 2 mg RES were completely dissolved in 1 mL methanol. Then obtained organic solution was added drop-wise into 10 mL distilled water under vigorous stirring for 6 h at room temperature in the dark. The solution was evaporated in vacuum to remove residual organic solvent. The final F127-SS-TOC/RES micelles were acquired by filtering with 0.45 μm membrane. In addition, the synthesis and characterization of F127-TOC polymer was supplied in ESI (Fig. S1 and S2†). F127-TOC/RES, blank F127-SS-TOC and blank F127-TOC micelles were also prepared by the above-mentioned method.
2.4 Characterization of F127-SS-TOC/RES micelles
RES loaded F127-SS-TOC micelles were analyzed based on their particle size, zeta potential and morphology. The particle size and zeta potential of F127-SS-TOC/RES were detected by dynamic light scattering (DLS) of Zetasizer Nano ZSP system (Malvern Instruments, Malvern, UK) at 25 °C. F127-SS-TOC/RES micelle morphology was observed by transmission electron microscopy (TEM, Tecnai G20, FEI Company, Oregon, USA) under the accelerating voltage of 200 kV. The characterization of F127-TOC micelles was supplied in ESI (Fig. S3 and S4†).
The quantification of RES in F127-SS-TOC/RES micelles and F127-TOC/RES micelles were measured by Shimadzu LC-20AT HPLC with a C18 reverse-phase liquid chromatography column (250 × 4.6 mm) under the flow rate of 1 mL min−1 and maximum absorption wavelength of 306 nm. The mobile phase was acetonitrile/0.25% acetic acid (35/65, v/v). F127-SS-TOC/RES micelles and F127-TOC/RES micelles were dissolved in methanol to disrupt micellar shell and the concentration of entrapped RES was determined by above-mentioned HPLC method. The drug loading (DL) was calculated by the following equations:
DL (%) = amount of the drug in micelles/amount of materials and drugs × 100% |
2.5 In vitro release of resveratrol from micelles
The drug release kinetics of RES from F127-SS-TOC micelles and F127-TOC micelles were carried out under the condition of reducing or non-reducing medium at 37 °C by dialysis method. Dithiothreitol (DTT) was used as a substitute to instead the reducing agents in intracellular environment, such as glutathione (GSH). In order to demonstrate the reducibility of F127-SS-TOC/RES micelles or F127-TOC/RES micelles, the release medium was consist of phosphate-buffered saline solution (PBS, pH 7.4) containing 0.1% (w/v) Tween 80 with or without 10 mM DTT. 2 mL F127-SS-TOC/RES micelle was placed in a dialysis bag (MWCO: 3500 Da), respectively. The dialysis bag was immersed completely in 30 mL release media in presence or absence of 10 mM DTT and gently shaken at 37 °C in shaking incubator at 100 rpm. At certain time interval, 1 mL release medium was got out and replaced the equal volume fresh medium into this system. The amount of RES in the collected release medium was determined by HPLC as above-mentioned method.
2.6 The stability of F127-SS-TOC/RES micelles
The stability is one of the important parameters to evaluate the characteristics in micelles. The average particle size and zeta potential of F127-SS-TOC/RES micelles were measured by DLS for 7 days to evaluate the stability of micelles. The micelles were prepared by the method mentioned above, and the temperature was kept at room temperature.
Besides, the stability of F127-SS-TOC/RES micelles was also investigated by examining the average particle size change in the presence of different FBS concentrations, which could mimic the stability of samples in blood circulation by in vitro experiments. Briefly, F127-SS-TOC/RES micelles were dispersed in water contained 10%, 20%, 30%, 40% or 50% FBS, and incubated at 37 °C for different time (4 h, 8 h, 12 h, 24 h). At scheduled time points, the particle size of micelles was measured by DLS. The particle size change ratios between the hydrodynamic diameter and its initial particle size appearance were calculated at different time points.
2.7 Cytotoxicity assay
In vitro cytotoxicity of F127-SS-TOC/RES, F127-TOC/RES, blank F127-SS-TOC and F127-TOC micelles against MDA-MB-231 and MCF-7 cells were determined by MTT assay. MDA-MB-231 and MCF-7 cells were seeded in 96-well plates at a density of 5000 cells per well in 100 μL DMEM medium. After incubation overnight, the cells were treated with free RES, F127-SS-TOC/RES and F127-TOC/RES micelles at different concentrations ranging from 5 μM to 160 μM, and the blank F127-SS-TOC and F127-TOC micelles were added to the cells at the concentrations ranging from 12.5 to 200 μg mL−1, respectively. After incubation 48 h at 37 °C, the medium containing MTT solution (1 mg mL−1) was added to per well and replaced the culture media. Cells were cultured for another 4 h and then discarded the medium containing MTT solution before adding 100 μL DMSO for the purpose of dissolving formazan crystals. The optical absorbance value recorded by a microplate reader (SpectaMax M5, Molecular Devices) at 570 nm, which associated with the cell viability. The experiments were repeated in triplicate.
2.8 Apoptosis assay or Annexin V-FITC/PI assay
Apoptotic cells caused by free drug or drug loaded micelles were determined by Annexin V-FITC/propidium iodide (PI) double staining assay in this experiment. MDA-MB-231 cells were seeded on 6-well plates at a density of 2 × 105 cell per well. MDA-MB-231 cells were treated with free RES solution, F127-TOC/RES and F127-SS-TOC/RES micelles in an equal concentration of 80 μM RES for 48 h. Untreated cells as control group. The cells were collected after incubation for 48 h, and washed twice with cold FBS. Then the cells were re-suspended in 200 μL binding buffer-dilute Annexin-FITC solution followed by incubation in the dark for 15 min at room temperature. PI solution was added to cells before flow cytometry analysis was analyzed.
2.9 Intracellular reactive oxygen species (ROS) generation
The determination of intracellular ROS formation might associate with pro-apoptotic effects and mechanisms of samples on cancer cells. Intracellular ROS could be detected by the probe 2′,7′-dichlorodihydrofluorescein diacetate DCFH-DA16 on MDA-MB-231 cells. Briefly, MDA-MB-231 cells were treated with free RES, F127-SS-TOC/RES and F127-TOC/RES micelles at an equal drug concentration of 80 μM for 4 h. After incubation, the cells were washed twice by PBS and incubator with 10 μM DCFH-DA for 30 min at 37 °C, 5% CO2 in the dark. Dichlorofluorescein (DCF) showed green fluorescence which converted by the interaction of DCFH-DA and ROS. The intensity of green fluorescence generated by DCF reflected the intracellular ROS was measured by a microplate reader (SpectaMax M5, Molecular Devices) with an excitation wavelength of 485 nm and the emission wavelength of 538 nm.
2.10 Statistical analysis
Data were from three experiments as the means ± SD and statistical analysis was done by GraphPad Prism 5 software. Statistical comparison was analyzed by t-test. A p value < 0.05 was considered as statistical significance, and very significant for **p < 0.01 and ***p < 0.001.
3 Results
3.1 Preparation and characterization of F127-SS-TOC/RES micelles
The amphiphilic polymer F127-SS-TOC could encapsulate RES into micelles (F127-SS-TOC/RES) by the solvent evaporation method. Scheme 1 showed the illustration of the preparation of RES-loaded F127-SS-TOC (F127-SS-TOC/RES) micelles. The particle size of nanoparticle ranged from 10 to 200 nm could facilitate drug to accumulate in the tumor site by enhanced permeability and retention (EPR) effect. In this study, DLS was applied to measure the average particle size, particle dispersion index (PDI) and zeta potential of F127-SS-TOC/RES micelles. As shown in Fig. 1A, the particle size and PDI of F127-SS-TOC/RES micelles were 39.50 ± 1.39 nm and 0.16 ± 0.01, respectively, which indicated RES-loaded micelles were small in particle size and might suitable for tumor site accumulation. In addition, the zeta potential of F127-SS-TOC/RES micelles was −4.55 ± 0.39 mV measured by DLS.
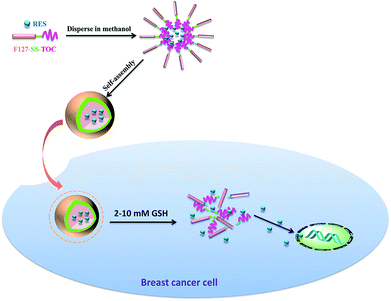 |
| Scheme 1 Illustration of the preparation of RES-loaded F127-SS-TOC (F127-SS-TOC/RES) micelles. | |
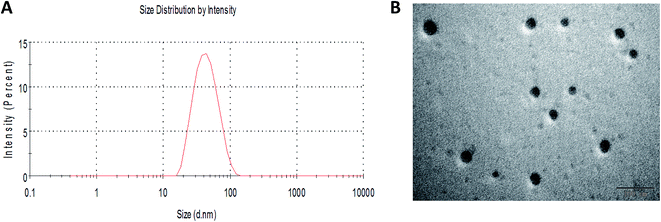 |
| Fig. 1 Characterization of F127-SS-TOC/RES micelles. (A) Particle size of F127-SS-TOC/RES micelles. (B) TEM image of F127-SS-TOC/RES micelles. The scale bars correspond to 100 nm in the image. | |
The TEM images of F127-SS-TOC/RES micelles were benefit for observing the shape of micelles, it was also an important parameter to evaluate nanoparticle. The morphology of F127-SS-TOC/RES micelles was observed in Fig. 1B, which illustrated that the RES loaded micelles had homogeneous and integrated spherical shapes with smooth surface and without aggregation. The particle sizes of F127-SS-TOC/RES micelles in TEM image were similar to the results measured by DLS. The drug loading of RES in F127-SS-TOC micelles was determined to be 13.25 ± 2.29%, respectively. Hence, RES was effectively encapsulated in F127-SS-TOC micelles with favorable integrity of structure.
3.2 The stability of F127-SS-TOC/RES micelles
The storage stability of F127-SS-TOC/RES micelles over a period of 7 days was evaluated via the change of particle size and zeta potential. As shown in Fig. 2A, there was no significant change in particle size or zeta potential value at 25 °C.
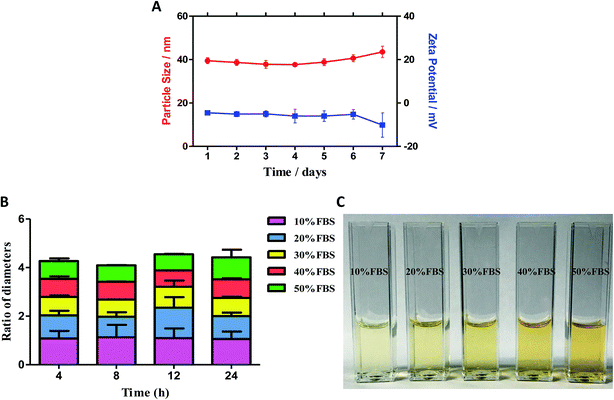 |
| Fig. 2 (A) The particle size and zeta potential of F127-SS-TOC/RES micelles at 25 °C after storage for 7 days. (B) The stability of F127-SS-TOC/RES micelles in water containing 10%, 20%, 30%, 40% and 50% FBS during 24 h at 37 °C. The particle size change ratio was the ratio between the hydrodynamic diameter and the initial diameter in water at different time point. (C) Photographs of F127-SS-TOC/RES micelles after treated with 10%, 20%, 30%, 40% and 50% FBS at 37 °C for 24 h. | |
The stability of F127-SS-TOC micelles in the presence of Fetal Bovine Serum (FBS) was estimated in this test. As shown in Fig. 2B, F127-SS-TOC/RES micelles were stable in water containing 10%, 20%, 30%, 40% and 50% FBS at 37 °C by the reason of no significant particle size change at different time point. The particle size change ratio of F127-SS-TOC micelles dispersed in water with 50% FBS was about 0.9-fold increase of the initial diameter after 24 h at 37 °C, indicating that F127-SS-TOC micelles was stable even in high concentration of FBS. Moreover, the diameter ratio of F127-SS-TOC micelles dispersed in water with 10% FBS was approximately 1.06-fold augment of the initial diameter after 24 h at the same condition, which might show that the RES loaded micelles had great potential in maintaining the structure during the blood circulation. Furthermore, the drug loaded micelles F127-SS-TOC/RES still exhibited good dispersion in water containing different concentrations of FBS without visible particle precipitation at 24 h (Fig. 2C).
3.3 In vitro release of resveratrol from micelles
The in vitro drug release of free RES, F127-SS-TOC/RES micelles and F127-TOC/RES micelles were investigated under sink conditions. As shown in Fig. 3, the release rate of RES released from micelles in medium with or without 10 mM DTT was slower than free RES solution in medium without DTT. Moreover, the drug cumulative release of free RES solution was much higher than another four groups until 48 h. In the presence of DTT, the cumulative release of F127-SS-TOC/RES micelles was higher than the same RES loaded micelles in PBS without DTT, but less than free RES solution without DTT at 48 h. The cumulative release profiles of F127-TOC/RES micelles were similar no matter in the presence of DTT or not. The cumulative release of free RES solution, F127-SS-TOC/RES micelles or F127-TOC/RES micelles with DTT and F127-SS-TOC/RES micelles or F127-TOC/RES micelles without DTT at 48 h was about 85%, 58%, 41% 45% and 37%, respectively. Compared to F127-SS-TOC/RES micelles without DTT group, the higher release percentage of F127-SS-TOC/RES micelles with DTT showed that the high concentration of reducing agents (10 mM DTT) could promote the cleavage of disulfide bonds in F127-SS-TOC polymer, which was benefit for the cracking of RES loaded micelles and releasing the drug from micelles. This phenomenon also reflected the intracellular high GSH concentration in tumor cells, which might trigger the disruption of F127-SS-TOC/RES micelles. Therefore, this test also demonstrated that F127-SS-TOC/RES micelles had redox-sensitivity that triggered by DTT, which was matched with our previous study.17 However, the presence of DTT showed no significant difference with the release profiles of F127-TOC/RES micelles, which demonstrated F127-TOC/RES micelles had non-redox-sensitivity. In addition, the drug release profile reflected that RES could incorporate into the hydrophobic core of F127-SS-TOC micelles with a slow release behavior compared to free drug. In general, the results showed that RES-loaded F127-SS-TOC micelles had sustained release behavior and redox-sensitive ability, which was conducive to maintain an effective concentration during the therapy.
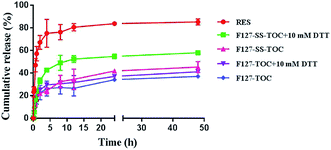 |
| Fig. 3 In vitro release profile of RES from F127-SS-TOC/RES micelles and F127-TOC/RES micelles in PBS (pH 7.4) containing 0.1% Tween 80 (v/v) with or without 10 mM DTT. | |
3.4 Cytotoxicity assay
The cytotoxicity of free RES, F127-TOC/RES (FT/RES), F127-SS-TOC/RES (FST/RES) micelles against MCF-7 and MDA-MB-231 cells was evaluated using MTT assay. The blank polymers of F127-TOC or F127-SS-TOC at the concentration of 200 μg mL−1, which was higher than the concentration of blank polymers used for forming RES loaded micelles F127-TOC/RES or F127-SS-TOC/RES, had rare cytotoxicity in MCF-7 and MDA-MB-231 cells (Fig. 4A and B). The results demonstrated that the cytotoxicity of F127-TOC/RES and F127-SS-TOC/RES micelles had little effects caused by polymers. In addition, both F127-TOC/RES and F127-SS-TOC/RES micelles had higher cytotoxicity than free RES, especially more significant in F127-SS-TOC/RES micelles, accompany with the time and concentration-dependent inhibited efficiency on MCF-7 and MDA-MB-231 cells. This phenomenon might associate with the redox-sensitivity of F127-SS-TOC/RES micelles which triggered by the high concentration of GSH in cancer cells. In MCF-7 cancer cells, The IC50 values were 75.8 μM for free RES, 74.43 μM for F127-TOC/RES micelles and 45.38 μM for F127-SS-TOC/RES micelles after 48 h incubation. Furthermore, the cytotoxicity of F127-SS-TOC/RES micelles showed very significant difference compared to free RES in MCF-7 cells. Markedly, in MDA-MB-231 cells, the IC50 values of F127-SS-TOC/RES micelles were much lower than that of free RES and F127-TOC/RES micelles at the equivalent drug concentration and certain treatment period, which were 79.61 μM for free RES comparing to 46.10 μM for F127-TOC/RES micelles and 26.10 μM for F127-SS-TOC/RES micelles after 48 h treatment (Fig. 4C and D). Besides, F127-SS-TOC/RES micelles had very significant difference in MDA-MB-231 cells compared to free RES and F127-TOC/RES micelles. These results showed that F127-SS-TOC/RES micelles possessed significantly enhanced cytotoxicity than free RES and F127-TOC/RES micelles in MCF-7 cells and MDA-MB-231 cells.
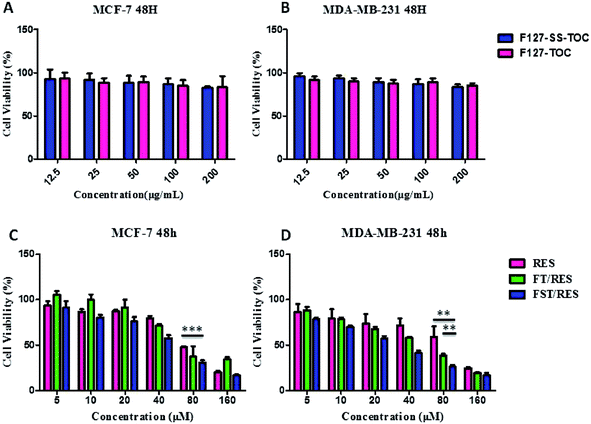 |
| Fig. 4 Cytotoxicity of F127-SS-TOC, F127-TOC against MCF-7 (A) and MDA-MB-231 (B) cells for 48 h. Cell viability of MCF-7 (C) and MDA-MB-231 (D) cells treated with different concentrations of free RES, F127-TOC/RES, F127-SS-TOC/RES micelles for 48 h. Very significant difference between F127-SS-TOC/RES micelles and free RES treated with 80 μM in MCF-7 cells represented as ***p < 0.001. Significant difference between F127-SS-TOC/RES micelles and free RES or F127-SS-TOC/RES micelles and F127-TOC/RES micelles treated with 80 μM in MDA-MB-231 cells represented as **p < 0.01. | |
3.5 Apoptosis assay or Annexin V-FITC/PI assay
Apoptotic induction is a vital tactic in cancer chemotherapy, and it is reported that RES have the ability to induce pro-apoptotic effect in cancer cells. In this study, cell apoptosis assay was performed by flow cytometry with Annexin V-FITC/PI double staining method to detect cell apoptosis rate. As shown in Fig. 5, MDA-MB-231 cells treated with F127-SS-TOC/RES micelles for 48 h had a significant pro-apoptotic effects compared to F127-TOC/RES micelles and free RES at the equal concentration of RES in 80 μM. The order of inducing apoptosis treated by different samples on MDA-MB-231 cells for 48 h were as follows: F127-SS-TOC/RES micelles (62.7%) > F127-TOC/RES micelles (49.82%) > free RES (43.11%) > control (2.66%). The results indicated that F127-SS-TOC/RES micelles could trigger by reductive environment on cancer cells and quickly release RES to have an effect on inducing cancer cells apoptosis, which was better than the pro-apoptotic effects of non-reductive F127-TOC/RES micelles and free RES.
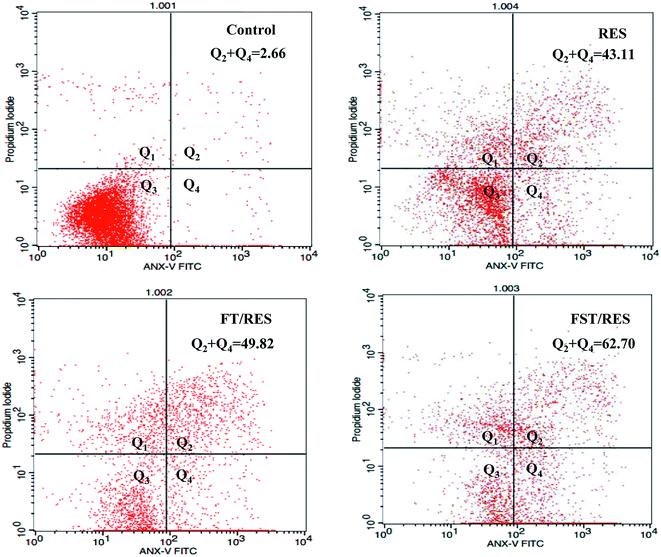 |
| Fig. 5 Cell apoptosis of MDA-MB-231 cells treated by free RES, F127-SS-TOC/RES (FST/RES) and F127-TOC/RES (FT/RES) micelles for 48 h at RES concentration of 80 μM. Q1, Q2, Q3, and Q4 phases represent necrotic cells, late apoptotic cells, live cells, and early apoptotic cells, respectively. | |
3.6 Intracellular reactive oxygen species (ROS) generation
Numerous studies indicated that ROS was closely related to cancer.24 In order to determine whether the cytotoxicity of cancer cells induced by RES was related to ROS, the intracellular ROS level was measured by ROS assay kit, where the fluorescent dye DCFH-DA converted into DCF accompanying by ROS detecting. As shown in Fig. 6, compared to free RES, F127-SS-TOC/RES micelles could significant induce a higher ROS production in MDA-MB-231 cells at RES concentration of 80 μM for 4 h, however, there was no significant difference between F127-SS-TOC/RES micelles and F127-TOC/RES micelles. In same condition, the ability generated intracellular ROS of different groups in MDA-MB-231 cells followed the order: F127-SS-TOC/RES micelles > F127-TOC/RES micelles > free RES > control. It was reported that the augment of ROS might lead to cell apoptosis or cell growth arrest. Hence, the results showed that redox-sensitive F127-SS-TOC/RES micelles have the greater potential in generating ROS in MDA-MB-231 cells, which might play an important role in triggering cancer cells death.
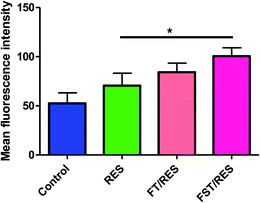 |
| Fig. 6 Intracellular ROS level in MDA-MB-231 cells treated by free RES, F127-SS-TOC/RES (FST/RES) and F127-TOC/RES (FT/RES) micelles at an equivalent RES concentration of 80 μM for 4 h by microplate reader determination. The results were expressed as mean ± SD. Significant difference between FST/RES micelles and free RES represented as *p < 0.05. | |
4 Conclusion
In this study, the prepared RES loaded F127-SS-TOC micelles via solvent evaporation method possessed several attractive features including satisfactory particle size, relatively high drug loading, redox-sensitive drug release, favourable stability at 25 °C for 7 days or in water containing FBS at 37 °C. Due to F127-SS-TOC polymer well encapsulate RES to form micelles and maintain good status, which might deliver the drug into tumor site by EPR effect. The disulfide bond existed in F127-SS-TOC polymer would disassociate in cancer cells with high concentration of GSH, following the rapid release of RES from micelles. Additionally, increased cytotoxicity, higher cell apoptosis and more ROS generation against breast cancer cells were discovered compared to free RES and non-redox-responsive F127-TOC/RES micelles. In summary, the F127-SS-TOC polymer could be a potential carrier for drug delivery and enhanced drug treatment in cancer cells.
Conflicts of interest
There are no conflicts to declare.
Acknowledgements
This study was supported by the Ministry of Science and Technology of the People's Republic of China (ZYBZH-C-GZ-10, ZYBZH-C-JL-25), the Beijing Municipal Science & Technology Commission (Z161100001816044) and the Fundamental Research Funds for the Central public welfare research institutes (ZXKT17006).
References
- L. Le Corre, N. Chalabi, L. Delort, Y. J. Bignon and D. J. Bernard-Gallon, Mol. Nutr. Food Res., 2005, 49, 462–471 CAS.
- S. Gangopadhyay, A. Nandy, P. Hor and A. Mukhopadhyay, Clin. Breast Cancer, 2013, 13, 7–15 CrossRef CAS PubMed.
- C. Gong, Y. Xie, Q. Wu, Y. Wang, S. Deng, D. Xiong, L. Liu, M. Xiang, Z. Qian and Y. Wei, Nanoscale, 2012, 4, 6004–6017 RSC.
- A. M. Friedhuber, V. Chandolu, S. Manchun, O. Donkor, P. Sriamornsak and C. R. Dass, J. Pharm. Pharmacol., 2015, 67, 68–77 CrossRef CAS PubMed.
- S. Biswas, N. S. Dodwadkar, P. P. Deshpande and V. P. Torchilin, J. Controlled Release, 2012, 159, 393–402 CrossRef CAS PubMed.
- H. Fujii, M. Shin-Ya, S. Takeda, Y. Hashimoto, S. A. Mukai, S. Sawada, T. Adachi, K. Akiyoshi, T. Miki and O. Mazda, Cancer Sci., 2014, 105, 1616–1625 CrossRef CAS PubMed.
- Y. Lu and K. Park, Int. J. Pharm., 2013, 453, 198–214 CrossRef CAS PubMed.
- Q. S. Zhao, L. L. Hu, Z. D. Wang, Z. P. Li, A. W. Wang and J. Liu, Mater. Sci. Eng., C, 2017, 72, 185–191 CrossRef CAS PubMed.
- T. Woraphatphadung, W. Sajomsang, P. Gonil, A. Treetong, P. Akkaramongkolporn, T. Ngawhirunpat and P. Opanasopit, Int. J. Pharm., 2016, 497, 150–160 CrossRef CAS PubMed.
- H. Lu, X. Zhao, W. Tian, Q. Wang and J. Shi, RSC Adv., 2014, 4, 18460–18466 RSC.
- X.-Z. Yang, X.-J. Du, Y. Liu, Y.-H. Zhu, Y.-Z. Liu, Y.-P. Li and J. Wang, Adv. Mater., 2014, 26, 931–936 CrossRef CAS PubMed.
- Y.-C. Ma, J.-X. Wang, W. Tao, H.-S. Qian and X.-Z. Yang, ACS Appl. Mater. Interfaces, 2014, 6, 16174–16181 CAS.
- K. Greish, Methods Mol. Biol., 2010, 624, 25–37 CAS.
- G. H. Gao, Y. Li and D. S. Lee, J. Controlled Release, 2013, 169, 180–184 CrossRef CAS.
- Y. Bao, Y. Guo, X. Zhuang, D. Li, B. Cheng, S. Tan and Z. Zhang, Mol. Pharmaceutics, 2014, 11, 3196–3209 CrossRef CAS PubMed.
- F. Chen, J. Zhang, Y. He, X. Fang, Y. Wang and M. Chen, Biomater. Sci., 2016, 4, 167–182 RSC.
- Y. Liu, S. Fu, L. Lin, Y. Cao, X. Xie, H. Yu, M. Chen and H. Li, Int. J. Nanomed., 2017, 12, 2635–2644 CrossRef PubMed.
- H. T. Yin, Q. Z. Tian, L. Guan, Y. Zhou, X. E. Huang and H. Zhang, Asian Pac. J. Cancer Prev., 2013, 14, 1703–1706 CrossRef PubMed.
- Y. Hu, S. Wang, X. Wu, J. Zhang, R. Chen, M. Chen and Y. Wang, J. Ethnopharmacol., 2013, 149, 601–612 CrossRef CAS PubMed.
- S. Wang, R. Chen, J. Morott, M. A. Repka, Y. Wang and M. Chen, Expert Opin. Drug Delivery, 2015, 12, 361–373 CrossRef CAS PubMed.
- L. Le Corre, N. Chalabi, L. Delort, Y. J. Bignon and D. J. Bernard-Gallon, Mol. Nutr. Food Res., 2005, 49, 462–471 CAS.
- P. Signorelli and R. Ghidoni, J. Nutr. Biochem., 2005, 16, 449–466 CrossRef CAS PubMed.
- I. A. Siddiqui, V. Sanna, N. Ahmad, M. Sechi and H. Mukhtar, in Resveratrol and Health, ed. O. Vang and J. M. Pezzuto, Blackwell Science Publ, Oxford, 2015, vol. 1348, pp. 20–31 Search PubMed.
- S. Prasad, S. C. Gupta and A. K. Tyagi, Cancer Lett., 2017, 387, 95–105 CrossRef CAS PubMed.
Footnote |
† Electronic supplementary information (ESI) available. See DOI: 10.1039/c7ra08593a |
|
This journal is © The Royal Society of Chemistry 2017 |
Click here to see how this site uses Cookies. View our privacy policy here.