DOI:
10.1039/C7RA08811F
(Paper)
RSC Adv., 2017,
7, 47753-47757
Highly diastereoselective crystallization-induced asymmetric transformation of 1,3-disubstituted-tetrahydro-β-carbolines in water†
Received
9th August 2017
, Accepted 27th September 2017
First published on 11th October 2017
Abstract
A green and highly stereoselective method for the synthesis of cis or trans-1,3-disubstituted-tetrahydro-β-carbolines has been developed using water as the solvent. A mixture of cis and trans-1,3-disubstituted-tetrahydro-β-carboline hydrochlorides can be converted to a single cis or trans isomer via a crystallization-induced asymmetric transformation process. It is possible to get both isomers using this method by incorporating different additives in water. This method has advantages such as environmental friendliness, high stereoselectivity, suitability for industrialization, and non-toxicity.
Introduction
Water is one of the most common resources on earth, and has played an important role in humanity’s development and engenders life. As we know, enzymatic processes in nature can only occur in an aqueous environment. Water is not only a medium for biochemical reactions, but is also a reagent in some oxidation and reduction reactions in the body. As people pay more and more attention to environmental protection, there is a tendency to implement green chemistry in organic synthesis.1 Chemists have devoted a lot of research effort to replacing harmful and toxic solvents with more environmentally friendly alternatives in the past two decades. Water is considered a green solvent for organic reactions, having advantages like non-toxicity, non-flammability, non-volatility, cheapness, and ready availability. One of the earliest examples was reported by Diels and Alder in 1931, for the Diels–Alder reaction of furan and maleic anhydride.2 More and more researchers have carried out various organic reactions using water as a solvent in recent years.3 Nevertheless, it is still a highly challenging task to find practical reactions which use water as solvent because of the drawbacks such as the insolubility of organic reactants and high surface tension.
Crystallization-induced asymmetric transformations (CIATs), which are also referred to as crystallization-induced stereoisomer transformations (CISTs), are a highly practical means for converting enantiomeric or diastereomeric mixtures into a single stereoisomeric product with a theoretical yield of 100% via simple crystallization under appropriate conditions.4 It can in principle be divided into two types: crystallization-induced enantiomer transformations (CIETs) and crystallization-induced diastereomer transformations (CIDTs). As CIAT processes are consistent with the requirements of “atom-economy” and green chemistry, and as the synthesis of chiral compounds becomes more and more important, there has been an explosion of research activities utilizing CIAT techniques to prepare chiral compounds in the last few decades.5,4c–e However, new, efficient and highly stereoselective CIAT processes remain highly desirable.
The tetrahydro-β-carboline scaffold exists widely in natural products and medicinal compounds, so the asymmetric syntheses of chiral tetrahydro-β-carbolines have attracted much attention.6 1,3-Disubstituted-tetrahydro-β-carbolines (1,3-disubstituted-THβCs) are an important kind of compound due to their various biological activities7 including antitumor,7a antioxidant,7b antiparasitic,7c anti-inflammatory,7d antithrombotic,7e fungicidal,7f antiviral7g properties and their use in the treatment of type 2 diabetes mellitus.7h Several methods have been reported for the preparation of optically pure 1,3-disubstituted-THβCs.8 However, most of them have drawbacks such as poor stereoselectivity,8a the introduction and removal of sterically hindered groups,8b–e low yield8f,8g and using harmful solvents.8h Herein, we describe the first highly stereoselective method for the synthesis of cis or trans 1,3-disubstituted-THβCs via a CIAT process in water.
Results and discussion
Our investigation commenced with the preparation of mixtures of cis and trans 1,3-disubstituted-THβCs via the classical Pictet–Spengler reaction. We obtained the mixtures of cis and trans 1,3-disubstituted-THβCs hydrochlorides in 85–98% yields via the condensation of L-tryptophan ester hydrochlorides with different aldehydes under reflux for 2–12 hours in isopropanol. The diastereomeric ratio of the cis and trans-isomers ranged from 11
:
89 to 92
:
8 (see Scheme 1). We then tried to convert the mixtures of 1,3-disubstituted-THβCs to a single isomer via a CIAT process in water. We began our study with mix-1a·HCl [mixtures of (1S,3S) and (1R,3S)-diastereomers] as the model substrate. The starting diastereomeric ratio of the cis and trans-isomers was 46
:
54 after the Pictet–Spengler reaction. We initially put mix-1a·HCl in pure water and heated it to 95 °C. We found that part of the solid dissolved with a quick conversion of the cis-isomer to the trans-isomer at the beginning. However, the rate of conversion slowed down rapidly and equilibrium between the cis and trans-isomers was reached after two hours. The diastereomeric ratio of the cis and trans-isomers changed to 16
:
84.
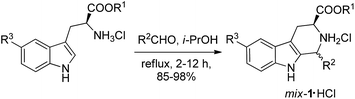 |
| Scheme 1 Synthesis of mix-1·HCl from L-tryptophan ester hydrochloride. | |
We thought that the high solubility of the materials probably resulted in the poor stereoselectivity of the CIAT process. Inspired by the salting-out effect and ionic equilibrium theory, we used 5% sodium chloride aqueous solution as the solvent. To our surprise, the diastereomeric ratio of the cis and trans-isomers changed to 6
:
94 when equilibrium was reached after 6 hours. Experiments were then performed to investigate the influence of the concentration of sodium chloride on the diastereomeric ratio and reaction rate (see Fig. 1). As can be seen from Fig. 1, when the concentration of sodium chloride was increased from 0% to 15%, the purity of the trans-isomer was improved from 84% to >99% after the equilibrium was finally reached. On the other hand, increasing the concentration of sodium chloride significantly decreased reaction rate. When a 10% sodium chloride aqueous solution was used as the solvent, a great diastereoselectivity (99
:
1) was obtained after 10 hours.
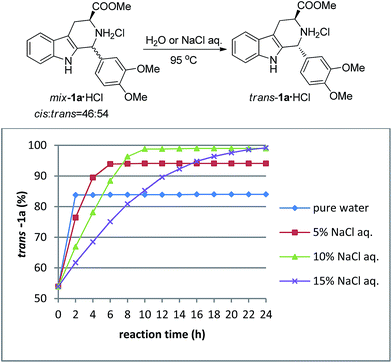 |
| Fig. 1 Influence of the concentration of NaCl on the diastereoselectivity and reaction rate of CIAT processes for mix-1a·HCl. | |
Next, we attempted to extend the scope of the above CIAT process (see Table 1). We found that the above CIAT process could be applied to almost all substrates; the CIAT of some substrates produced trans-isomers with high diastereoselectivities, while the CIAT of other substrates produced cis-isomers with high diastereoselectivities. We also found that the optimized concentrations of sodium chloride were different for various substrates, ranging from 0% to 20%.
Table 1 Highly stereoselective synthesis of cis or trans 1,3-disubstituted-THβCs via a CIAT process in watera

|
Reaction conditions: mix-1a–u·HCl (1 g), NaCl aqueous solution (8 mL), 95 °C. Isolated yields. Cis/trans ratios were determined by HPLC, the relative configurations of products were assigned by 1H–1H NOESY.8h |
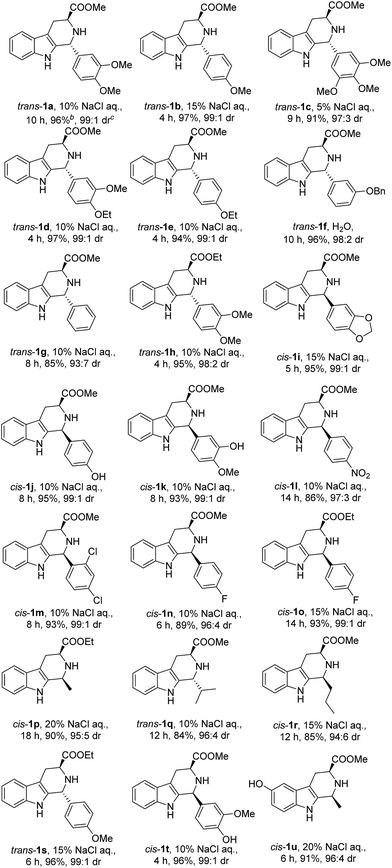 |
We have also investigated the influence of various salts on the above CIAT process using mix-1a·HCl; a total of twenty salts were examined and the results are summarized in Table 2. To our surprise, the addition of some salts produced trans-1a·HX as the major product (entries 1–8, 11–13 and 18–20), while the addition of other salts produced cis-1a·HX as the major product (entries 9, 10 and 14–17). We found that the different anions of the additive salts could change the configuration of the major product (comparing entries 1, 9, 11, 14, 15 and 17–20), whereas the different cations of the additive salts could not change the configuration of the major product (comparing entries 1–8 or 11–13). This is probably due to the fact that the different anions (X−) of the additive salts might exchange with the Cl− of mix-1a·HCl to crystallize out less soluble trans (or cis)-1a·HX.
Table 2 The effect of additives on the CIAT stereoselectivity of mix-1a·HCl
We then investigated the CIAT processes of other substrates by adding different salts. We examined the CIAT processes of all twenty-one substrates shown in Table 1. It was found that the configuration of the major products from the CIAT processes of six substrates could be changed by adding different salts (see Table 3). Therefore we can obtain both the cis and trans-isomers of these six substrates by incorporating different additive salts in water.
Table 3 The effect of different additive salts on the diastereoselectivities of CIATsa
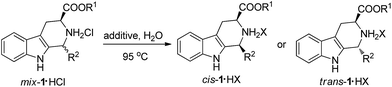
|
Reaction conditions: mix-1·HCl (1 g), 10% additive salt aqueous solution (8 mL), 95 °C. Isolated yields. Cis/trans ratios were determined by HPLC, the relative configurations of the products were assigned by 1H–1H NOESY.8h |
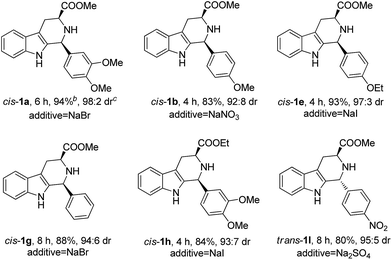 |
It is worth noting that cis or trans 1,3-disubstituted-THβCs, which can be readily obtained by the above CIAT process, are very useful for drug synthesis. For example, (+)-cis-1i was synthesized from D-tryptophan methyl ester hydrochloride via the same CIAT process, which is an important intermediate for the synthesis of Tadalafil (see Scheme 2),9 a selective PDE5 inhibitor. Additionally, trans-1f was successfully used herein for the synthesis of HR22C16 (see Scheme 2), which is a potent mitotic kinesin Eg5 inhibitor.10
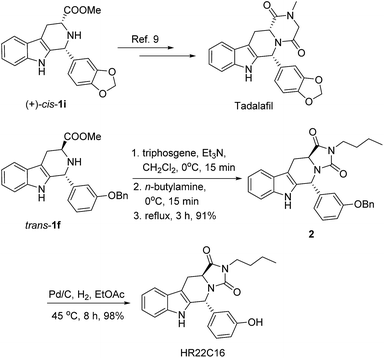 |
| Scheme 2 Syntheses of Tadalafil and HR22C16 from the 1,3-disubstituted-THβCs cis-1i and trans-1f, respectively. | |
Some people have proposed that the least soluble diastereomer will crystallize preferentially during a CIAT process.5b,11 Based on this assumption, a plausible mechanism for the above CIAT process of mix-1·HCl in aqueous NaCl solution is proposed in Scheme 3. Both the solid cis and trans-1·HCl would partially dissolve into the aqueous solution phase by dissociating into cis and trans-1 cations and chloride anions, the cis and trans-1 cations would interchange via cleavage and formation of the C–N bond.12 Crystallization of the less soluble isomer would shift the equilibrium to afford the less soluble isomer as the major product of the CIAT process.
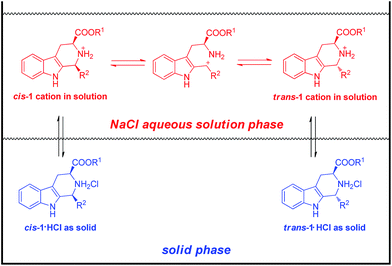 |
| Scheme 3 Proposed mechanism for the CIAT process of mix-1a·HCl in aqueous NaCl solution. | |
Conclusions
In conclusion, we have developed the first protocol for a highly diastereoselective CIAT process of 1,3-disubstituted-THβCs in water. This protocol provides efficient access to a single isomer of either cis or trans-1,3-disubstituted-THβCs, which are very useful for drug synthesis. It was also observed that addition of different salts in water exhibits an obvious influence on the diastereoselectivities and rate of the CIAT process. The above CIAT protocol has the following advantages: (1) it was successfully carried out in pure water, so it is environmentally benign; (2) all the products have good to excellent diastereoselectivities; (3) the scope of the CIAT process is very wide, it was applicable to all the tested substrates; (4) the experiments were carried out on a gram scale; (5) it is practical because the starting materials are easy to obtain and operation is very simple.
Conflicts of interest
There are no conflicts to declare.
Acknowledgements
We are grateful to the National Natural Science Foundation of China (No. 20972048) and the Shanghai Educational Development Foundation (The Dawn Program: No. 03SG27) for the financial support of this work.
Notes and references
-
(a) R. A. Sheldon, Green Chem., 2017, 19, 18 RSC
;
(b) F. Roschangar, R. A. Sheldon and C. H. Senanayake, Green Chem., 2015, 17, 752 RSC
;
(c) R. A. Sheldon, Chem. Soc. Rev., 2012, 41, 1437 RSC
;
(d) K. Sanderson, Nature, 2011, 469, 18 CrossRef CAS PubMed
;
(e) P. Anastas and N. Eghbali, Chem. Soc. Rev., 2010, 39, 301 RSC
;
(f) E. S. Beach, Z. Cui and P. T. Anastas, Energy Environ. Sci., 2009, 2, 1038 RSC
;
(g) M. Poliakoff and P. Licence, Nature, 2007, 450, 810 CrossRef CAS PubMed
;
(h) I. T. Horvath and P. T. Anastas, Chem. Rev., 2007, 107, 2169 CrossRef CAS PubMed
;
(i) J. H. Clark, Green Chem., 2006, 8, 17 RSC
;
(j) B. Horton, Nature, 1999, 400, 797 CrossRef CAS
;
(k) P. T. Anastas and J. C. Warner, Green Chemistry: Theory and Practice, Oxford University Press, UK, 1998 Search PubMed
. - O. Diels and K. Alder, Liebigs Ann. Org. Bioorg. Chem., 1931, 490, 243 CrossRef
. -
(a) M. B. Gawande, V. D. B. Bonifacio, R. Luque, P. S. Branco and R. S. Varma, Chem. Soc. Rev., 2013, 42, 5522 RSC
;
(b) M. O. Simon and C. J. Li, Chem. Soc. Rev., 2012, 41, 1415 RSC
;
(c) R. N. Butler and A. G. Coyne, Chem. Rev., 2010, 110, 6302 CrossRef CAS PubMed
;
(d) D. Dallinger and C. O. Kappe, Chem. Rev., 2007, 107, 2563 CrossRef CAS PubMed
;
(e) C. I. Herrerias, X. Yao, Z. Li and C. J. Li, Chem. Rev., 2007, 107, 2546 CrossRef CAS PubMed
;
(f) C. J. Li and L. Chen, Chem. Soc. Rev., 2006, 35, 68 RSC
;
(g) M. H. Shinde and U. A. Kshirsagar, Green Chem., 2016, 18, 1455 RSC
;
(h) L. Tang, Y. Yang, L. Wen, X. Yang and Z. Wang, Green Chem., 2016, 18, 1224 RSC
;
(i) W. Zhao, C. Yang, Z. Cheng and Z. Zhang, Green Chem., 2016, 18, 995 RSC
;
(j) T. Mizugaki, Y. Nagatsu, K. Togo, Z. Maeno, T. Mitsudome, K. Jitsukawa and K. Kaneda, Green Chem., 2015, 17, 5136 RSC
;
(k) V. S. Shende, S. H. Deshpande, S. K. Shingote, A. Joseph and A. A. Kelkar, Org. Lett., 2015, 17, 2878 CrossRef CAS PubMed
;
(l) M. C. Pirrung and K. D. Sarma, J. Am. Chem. Soc., 2004, 126, 444 CrossRef CAS PubMed
;
(m) W. J. Ang, Y. S. Chng and Y. Lam, RSC Adv., 2015, 5, 81415 RSC
;
(n) S. Paul, M. M. Islam and S. M. Islam, RSC Adv., 2015, 5, 42193 RSC
;
(o) C. Yang, W.-Q. Su and D.-Z. Xu, RSC Adv., 2016, 6, 99656 RSC
. -
(a) K. Sakai, N. Hirayama and R. Tamura, in Novel Optical Resolution Technologies, ed. R. Yoshioka, Springer-Verlag Berlin Heidelberg, New York, 2007, pp. 83–132 Search PubMed
;
(b) E. M. Carreia and H. Yamamoto, in Comprehensive Chirality, ed. R. M. Kellogg and M. Leeman, Elsevier Ltd., Groningen, 2012, vol. 9, pp. 367–399 Search PubMed
;
(c) N. G. Anderson, Org. Process Res. Dev., 2005, 9, 800 CrossRef CAS
;
(d) K. M. J. Brands and A.J. Davies, Chem. Rev., 2006, 106, 2711 CrossRef CAS PubMed
;
(e) S. Caddick and K. Jenkins, Chem. Soc. Rev., 1996, 25, 447 RSC
. -
(a) F. Belanfger, C. E. Chase, A. Endo, F. G. Fang, J. Li, S. R. Mathieu, A. Z. Wilcoxen and H. Zhang, Angew. Chem., Int. Ed., 2015, 54, 5108 CrossRef PubMed
;
(b) F. A. Kortmann, M.-C. Chang, E. Otten, E. P. A. Couzijn, M. Lutz and A. J. Minnaard, Chem. Sci., 2014, 5, 1322 RSC
;
(c) D. L. Jameson, T. Field, M. R. Schmidt, A. K. DeStefano, C. J. Stiteler, V. J. Venditto, B. Krovic, C. M. Hoffman, M. T. Ondisco and M. E. Belowich, J. Org. Chem., 2013, 78, 11590 CrossRef CAS PubMed
;
(d) A. Duris, T. Wiesenganger, D. Moravcikova, P. Baran, J. Kozisek, A. Daich and D. Berkes, Org. Lett., 2011, 13, 1642 CrossRef CAS PubMed
;
(e) T. Ueda, N. Kanomata and H. Machida, Org. Lett., 2005, 7, 2365 CrossRef CAS PubMed
;
(f) K. M. J. Brands, J. F. Payack, J. D. Rosen, T. D. Nelson, A. Candelario, M. A. Huffman, M. M. Zhao, J. Li, B. Craig, Z. J. Song, D. M. Tschaen, K. Hansen, P. N. Devine, P. J. Pye, K. Rossen, P. G. Dormer, R. A. Reamer, C. J. Welch, D. J. Mathre, N. N. Tsou, J. M. Mcnamara and P. J. Reider, J. Am. Chem. Soc., 2003, 125, 2129 CrossRef CAS PubMed
;
(g) E. Vedejs, R. W. Chapman, S. Lin, M. Muller and D. R. Powell, J. Am. Chem. Soc., 2000, 122, 3047 CrossRef CAS
;
(h) M. Petit, A. J. B. Lapierre and D. P. Curran, J. Am. Chem. Soc., 2005, 127, 14994 CrossRef CAS PubMed
;
(i) O. Hamelin, J. Pecaut and M. Fontecave, Chem.–Eur. J., 2004, 10, 2548 CrossRef CAS PubMed
. -
(a) J. Stöckigt, A. P. Antonchick, F. Wu and H. Waldmann, Angew. Chem., Int. Ed., 2011, 50, 8538 CrossRef PubMed
;
(b) S.-G. Wang, Z.-L. Xia, R.-Q. Xu, X.-J. Liu, C. Zheng and S.-L. You, Angew. Chem., 2017, 129, 7548 CrossRef
;
(c) S.-L. You, Q. Cai and M. Zeng, Chem. Soc. Rev., 2009, 38, 2190 RSC
;
(d) R. N. Rao, B. Maiti and K. Chanda, ACS Comb. Sci., 2017, 19, 199 CrossRef CAS PubMed
;
(e) Q. Yin, S.-G. Wang and S.-L. You, Org. Lett., 2013, 15, 2688 CrossRef CAS PubMed
;
(f) T. Arai, M. Wasai and N. Yokoyama, J. Org. Chem., 2011, 76, 2909 CrossRef CAS PubMed
;
(g) M. Jida, D. Tourwe and S. Ballet, RSC Adv., 2014, 4, 38159 RSC
;
(h) H.-L. Zhu, J.-B. Ling and P.-F. Xu, J. Org. Chem., 2012, 77, 7737 CrossRef CAS PubMed
;
(i) Q. Yin, S.-G. Wang and S.-L. You, Org. Lett., 2013, 15, 2688 CrossRef CAS PubMed
;
(j) W. Dai, H. Lu, X. Li, F. Shi and S.-J. Tu, Chem.–Eur. J., 2014, 20, 11382 CrossRef CAS PubMed
. -
(a) R. Yamanokuchi, K. Imada, M. Miyazaki, H. Kato, T. Watanabe, M. Fujimuro, Y. Sadki, S. Yoshinaga, H. Terasawa, N. Iwasaki, H. Rotinsulu, F. Losung, R. E. P. Mangindaan, M. Namikoshi, N. J. Voogd, H. Yokosawa and S. Tsukamoto, Bioorg. Med. Chem., 2012, 20, 4437 CrossRef CAS PubMed
;
(b) S. M. Lin, J. Y. Wu, C. Su, S. Ferng, C. Y. Lo and R. Y. Y. Chiou, J. Agric. Food Chem., 2012, 60, 9856 CrossRef CAS PubMed
;
(c) R. Brokamp, B. Bergmann, I. B. Muller and S. Bienz, Bioorg. Med. Chem., 2014, 22, 1832 CrossRef CAS PubMed
;
(d) X. Hu, M. Zhao, Y. Wang, Y. Wang, S. Zhao, J. Wu, X. Li and S. Peng, J. Mater. Chem. B, 2016, 4, 1384 RSC
;
(e) C. Li, X. Zhang, M. Zhao, Y. Wang, J. Wu, J. Liu, M. Zheng and S. Peng, Eur. J. Med. Chem., 2011, 46, 5598 CrossRef CAS PubMed
;
(f) H. J. Song, Y. X. Liu, Y. X. Liu, Y. Q. Huang, Y. Q. Li and Q. M. Wang, Bioorg. Med. Chem. Lett., 2014, 24, 5228 CrossRef CAS PubMed
;
(g) H. Song, Y. Liu, Y. Liu, L. Wang and Q. Wang, J. Agric. Food Chem., 2014, 62, 1010 CrossRef CAS PubMed
;
(h) A. Pasternak, Z. Feng, R. Jesus, Z. Ye, S. He, P. Dobbelaar, S. A. Bradley, G. G. Chicchi, K. L. Tsao, D. Trusca, G. J. Eiermann, C. Li, Y. Feng, M. Wu, Q. Shao, B. B. Zhang, R. Nargund, S. G. Mills, A. D. Howard, L. Yang and Y. P. Zhou, ACS Med. Chem. Lett., 2012, 3, 289 CrossRef CAS PubMed
. -
(a) P. D. Bailey, M. A. Beard and T. R. Phillips, Tetrahedron Lett., 2009, 50, 3645 CrossRef CAS
;
(b) J. Dong, T. Z. Meng, X. X. Shi, W. H. Zou and X. Lu, Tetrahedron: Asymmetry, 2013, 24, 883 CrossRef CAS
;
(c) S. Yu, O. M. Berner and J. M. Cook, J. Am. Chem. Soc., 2000, 122, 7827 CrossRef CAS
;
(d) J. Sandrin, S. P. Hollinshead and J. M. Cook, J. Org. Chem., 1989, 54, 5636 CrossRef CAS
;
(e) F. Ungemach, M. Dipierro, R. Weber and J. M. Cook, J. Org. Chem., 1981, 46, 164 CrossRef CAS
;
(f) V. F. Vavsari, V. Dianati, S. Ramezanpour and S. Balalaie, Synlett, 2015, 26, 1955 CrossRef CAS
;
(g) L. Alberch, P. D. Bailey, P. D. Clingan, T. J. Mills, R. A. Price and R. G. Pritchard, Eur. J. Org. Chem., 2004, 9, 1887 CrossRef
;
(h) S. Xiao, X. Lu, X. X. Shi, Y. Sun, L. L. Liang, X. H. Yu and J. Dong, Tetrahedron: Asymmetry, 2009, 20, 430 CrossRef CAS
. - A. Daugan, P. Grondin, C. Ruaut, A. C. L. M. Gouville, H. Coste, J. M. Linget, J. Kirilovsky, F. Hyafil and R. Labaudiniere, J. Med. Chem., 2003, 46, 4533 CrossRef CAS PubMed
. - S. Hotha, J. C. Yarrow, J. G. Yang, S. Garrett, K. V. Renduchintala, T. U. Mayer and T. M. Kapoor, Angew. Chem., Int. Ed., 2003, 42, 2379 CrossRef CAS PubMed
. -
(a) D. Berkes, P. Jakubec, D. Winklerova, F. Povazanec and A. Daich, Org. Biomol. Chem., 2007, 5, 121 RSC
;
(b) D. Berkes, A. Kolarovic, R. Manduch, P. Baran and F. Povazanec, Tetrahedron: Asymmetry, 2005, 16, 1927 CrossRef CAS
;
(c) T. Shiraiwa, S. Sakata, K. Fujishima and H. Kurokawa, Bull. Chem. Soc. Jpn., 1991, 64, 191 CrossRef CAS
. -
(a) M. L. V. Linn and J. M. Cook, J. Org. Chem., 2010, 75, 3587 CrossRef PubMed
;
(b) H. J. Kumpaty, M. L. V. Linn, M. S. Kabir, F. H. Forsterling, J. R. Deschamps and J. M. Cook, J. Org. Chem., 2009, 74, 2771 CrossRef CAS PubMed
;
(c) X. X. Shi, S. L. Liu, W. Xu and Y. L. Xu, Tetrahedron: Asymmetry, 2008, 19, 435 CrossRef CAS
;
(d) M. J. Earle, M. Noe, A. Perosa and K. R. Seddon, RSC Adv., 2014, 4, 1204 RSC
.
Footnote |
† Electronic supplementary information (ESI) available. See DOI: 10.1039/c7ra08811f |
|
This journal is © The Royal Society of Chemistry 2017 |
Click here to see how this site uses Cookies. View our privacy policy here.