DOI:
10.1039/C7RA11362E
(Paper)
RSC Adv., 2017,
7, 54399-54406
Brønsted acidic ionic liquid-promoted direct C3-acylation of N-unsubstituted indoles with acid anhydrides under microwave irradiation†
Received
15th October 2017
, Accepted 23rd November 2017
First published on 27th November 2017
Abstract
A green and efficient pathway for the synthesis of 3-acylindoles using a Brønsted acidic ionic liquid as a catalyst has been developed for the first time. The C3-acylation of N-unsubstituted indoles with acid anhydrides affords the desired products in good to excellent yields with high regioselectivity under microwave irradiation. Moreover, the Brønsted acidic ionic liquid can be recycled up to four times without significant loss of catalytic activity.
Introduction
Acylindoles are commonly found in many biologically active natural products and pharmaceutical compounds.1–3 Among these, 3-acylindole derivatives potentially show biological activities including anti-diabetic,2,3 anti-cancer,4–6 and anti-HIV.7–10 However, their synthesis remains challenging and includes N-protection,11 low yields,12,13 poor regioselectivity, excess reactants,14 high temperature, and long reaction time.11,15,16 Traditional methods involved the use of stoichiometric amounts of Lewis or Brønsted acids for the Friedel–Crafts acylation of indole.11,17,18 Recently, the preparation of 3-acylindoles has received much attention and metal-based catalytic pathways have been studied extensively.13,18 However, some of the existing methods are often harmful to the environment. Thus, developing new catalysts for the synthesis of 3-acylindoles with excellent yields and high selectivity under mild and environmentally friendly conditions is highly desirable.18
Ionic liquids have received much attention in many fields including catalysis,19–25 electrolytes,26–29 extraction desulfurization,30–32 biomass processing,33–35 and biodiesel.36–39 Friedel–Crafts C3-acylation of indoles using ionic liquids has been reported in previous literature.40,41 Recently, Brønsted acidic ionic liquids (ionic liquids with acidic properties) have been widely used for various organic transformations due to their unique properties.19,42–56 Nevertheless, to the best of our knowledge, they have never been used for the direct C3-acylation of N-unsubstituted indoles. Thus, we now report the development of a high-yielding and regioselectivity method for the C3-acylation N-unsubstituted indoles using Brønsted acidic ionic liquid as an efficiently homogeneous catalyst under microwave irradiation.
Results and discussion
Preparation of [(4-SO3H)BMIM]HSO4 ionic liquid
[(4-SO3H)BMIM]HSO4 ionic liquid has been prepared by two steps: formation of the zwitterionic intermediate and subsequent protonation by concentrated sulphuric acid. However, these methods suffered from some drawbacks such as low yields and long reaction times.36,37,57–60 Thus, the ultrasound irradiation was chosen to improve the reaction efficiency for the synthesis of [(4-SO3H)BMIM]HSO4. It should be noted that the two-step procedure provided [(4-SO3H)BMIM]HSO4 in excellent yield within very short reaction time under solvent-free sonication (please see ESI, Tables S1 and S2†). The structure of ionic liquid was characterized by NMR, FT-IR, and HR-MS (ESI). The ionic liquid is hygroscopic but moisture-stable and should be constantly stored in a desiccator.
Effect of reaction time and temperature for the C3-propionylation of indole
The catalytic activity of the prepared [(4-SO3H)BMIM]HSO4 was evaluated for the Friedel–Crafts acylation of indoles with acid anhydrides under solvent-free microwave irradiation. To optimize the reaction conditions, the reaction of indole and propionic anhydride under microwave irradiation was conducted as a model reaction. Initially, the Friedel–Crafts propionylation of indole in the presence of [(4-SO3H)BMIM]HSO4 (25 mol%) under microwave irradiation at 60 °C for 5 min was employed to prepare the desired product. To our surprise, the model reaction afforded the propionylindole in 46% yield with 100% regioselectivity in C3-position, and no impurity was found in TLC and GC (Table 1, entry 1). After screening the reaction conditions, we determined the optimal conditions at 100 °C for 5 min for the formation of the corresponding product in 92% yield (Table 1, entry 3). The control experiments were also carried out under conventional heating and ultrasound irradiation, but only a trace amount of 3-propionylindole was detected.
Table 1 Optimization of the reaction conditionsa
Effect of reaction time and amount of catalyst for the C3-propionylation of indole
Next, the amount of catalyst was varied from 5 to 100 mol% to study its effect on the yield of product. The results were presented in Table 2. The desired product was not detected in the absence of the catalyst (Table 2, entry 1). As only a small catalyst loading of 5 mol% was introduced to the reaction mixture, the desired product straightforwardly formed with a detectable yield of 35% (Table 2, entry 2). The best yield was obtained in 92% yield in the presence of 25 mol% of [(4-SO3H)BMIM]HSO4 ionic liquid under solvent-free microwave irradiation at 100 °C for 5 min (Table 2, entry 4).
Table 2 Effect of the catalytic amounta
Entry |
[(4-SO3H)BMIM]HSO4 (mol%) |
Yieldb (%) |
Selectivityc (1 : 2 : 3) |
Reaction condition: indole (1.0 mmol), propionic anhydride (1.0 mmol) under solvent-free microwave irradiation at 100 °C for 5 min. Isolated yield. Determined by GC-MS. |
1 |
0 |
0 |
0 |
2 |
5 |
35 |
0 : 0 : 100 |
3 |
15 |
60 |
0 : 0 : 100 |
4 |
25 |
92 |
0 : 0 : 100 |
5 |
50 |
92 |
1 : 0 : 99 |
6 |
100 |
93 |
2 : 1 : 97 |
Effect of acylating reagents for the C3-propionylation of indole
With optimal reaction conditions in hand, we extended the current method to other acid anhydrides such as acetic, butyric, isobutyric, pivalic, and benzoic anhydride. The results were presented in Table 3. The desired products were obtained in good to excellent yields (65–92%) with over 95% selectivity in C3-position. The low yields of pivalic and benzoic anhydride were obtained under current method presumably due to steric effect (Table 3, entries 5 and 6). Interestingly, no 1,3-diacylated and polymerized products were obtained under current method. The selectivity to C3-acylation can be rationalized based on the electron-donating resonance from the lone pair on the nitrogen atom to C3 through C2, giving rise to a partially negative charge on C3. The resonance pathway via benzene ring to end up with a partially negative charge on C2 is disfavored because it destroys the aromaticity of the benzene ring. The higher reactivity of C3-center over unsubstituted-NH moiety in this method can be attributed to the assumption that a soft electrophile as acid anhydride preferably attacks to a soft nucleophilic C3-position rather than a hard center as unsubstituted-NH moiety. We also experimentally tested this hypothesis by conducting the same reaction in which a typical hard electrophile, benzoyl chloride, was employed as acylation reagent instead of acid anhydrides. As expected, the N-benzoylated product was selectively formed in the yield of 80%.
Table 3 Effect of acylating reagentsa
Effect of substrate scope for the C3-acylation of indole derivative
To examine the scope of reaction, the reactions of various indoles and a wide range of acid anhydrides were investigated under current method. The results were presented in Table 4. A wide range of indoles bearing the substituent on the position C5 could proceed well and afford the corresponding products in good yields (Table 2, entries 6–20). Non-substituted indole and indoles bearing electron-donating groups such as 5-methylindole and 5-methoxyindole gave the desired products in excellent yields (Table 4, entries 1–10). Indoles bearing electron-poor group such as 5-fluoroindole, 5-chloroindole, 5-bromoindole were also reactive under high temperature and/or extended reaction times (Table 4, entries 11–19). The reaction was highly governed by steric effect as the reaction of 4-bromoindole with propionic and pivalic anhydride afforded the corresponding products in low yields and poor regioselectivity to C3-acylation (Table 4, entries 21–22).
Table 4 [(4-SO3H)BMIM]HSO4-catalyzed acylation of free (NH) indoles with acid anhydrides under solvent-free microwave irradiationa

|
Entry |
Indoles |
Acid anhydrides |
Condition |
Products |
Yieldb (%) |
Selectivityc (1 : 2 : 3) |
Reaction condition: indoles (1.0 mmol), acid anhydrides (1.0 mmol), and [(4-SO3H)BMIM]HSO4 (25 mol%) under solvent-free microwave irradiation. Isolated yield. Determined by GC-MS. |
1 |
 |
(CH3CO)2O |
100 °C, 8 min |
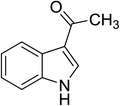 |
90 |
0 : 0 : 100 |
2 |
(C2H5CO)2O |
100 °C, 5 min |
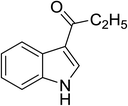 |
92 |
1 : 0 : 99 |
3 |
(n-C3H7CO)2O |
100 °C, 10 min |
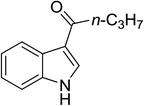 |
92 |
1 : 0 : 99 |
4 |
(t-C4H9CO)2O |
100 °C, 10 min |
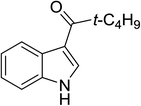 |
82 |
1 : 0 : 99 |
5 |
(C6H5CO)2O |
100 °C, 10 min |
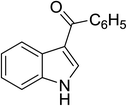 |
78 |
1 : 0 : 99 |
6 |
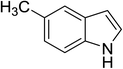 |
(CH3CO)2O |
100 °C, 5 min |
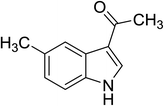 |
92 |
4 : 0 : 96 |
7 |
(C2H5CO)2O |
100 °C, 5 min |
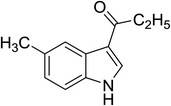 |
90 |
5 : 0 : 95 |
8 |
(i-C3H7CO)2O |
100 °C, 5 min |
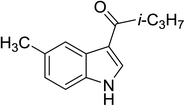 |
89 |
5 : 0 : 95 |
9 |
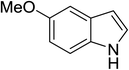 |
(CH3CO)2O |
100 °C, 5 min |
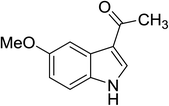 |
92 |
5 : 0 : 95 |
10 |
(i-C3H7CO)2O |
100 °C, 5 min |
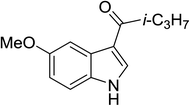 |
90 |
6 : 0 : 94 |
11 |
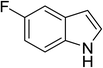 |
(CH3CO)2O |
100 °C, 10 min |
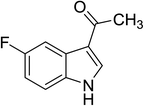 |
88 |
1 : 0 : 99 |
12 |
(C2H5CO)2O |
100 °C, 10 min |
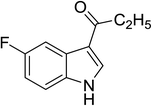 |
84 |
1 : 1 : 98 |
13 |
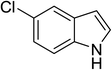 |
(CH3CO)2O |
100 °C, 10 min |
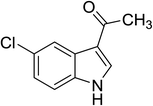 |
84 |
1 : 1 : 98 |
14 |
(C2H5CO)2O |
100 °C, 10 min |
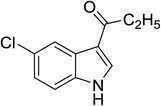 |
80 |
2 : 1 : 97 |
15 |
(n-C3H7CO)2O |
100 °C, 10 min |
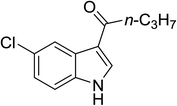 |
76 |
3 : 1 : 96 |
16 |
(i-C3H7CO)2O |
100 °C, 10 min |
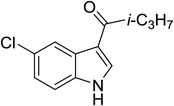 |
82 |
2 : 1 : 97 |
17 |
(t-C4H9CO)2O |
100 °C, 10 min |
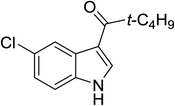 |
72 |
1 : 1 : 98 |
18 |
(C6H5CO)2O |
100 °C, 10 min |
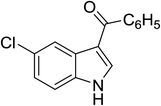 |
70 |
2 : 1 : 97 |
19 |
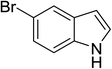 |
(C2H5CO)2O |
120 °C, 10 min |
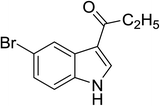 |
80 |
3 : 1 : 96 |
20 |
(t-C4H9CO)2O |
120 °C, 10 min |
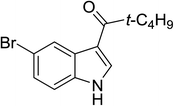 |
65 |
4 : 1 : 95 |
21 |
 |
(C2H5CO)2O |
120 °C, 10 min |
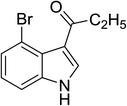 |
56 |
25 : 0 : 75 |
22 |
(t-C4H9CO)2O |
120 °C, 10 min |
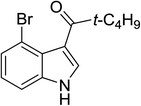 |
42 |
49 : 0 : 51 |
Investigation on recycling of [(4-SO3H)BMIM]HSO4 ionic liquid for the C3-propionylation of indole
The reusability of [(4-SO3H)BMIM]HSO4 ionic liquid was carried out in the model reaction at 100 °C for 5 min under microwave irradiation. The results were reported in Table 5. Four consecutive runs were tested with less reduction in the catalytic activity. FT-IR spectra of fresh and recovered [(4-SO3H)BMIM]HSO4 confirmed that the structure of ionic liquid kept unchanged.
Table 5 Reusability of [(4-SO3H)BMIM]HSO4 ionic liquid for the synthesis of 3-propionylindole
Run |
Isolated yield (%) |
Selectivity (1 : 2 : 3) |
0 |
90 |
0 : 0 : 100 |
1 |
90 |
1 : 0 : 99 |
2 |
89 |
1 : 0 : 99 |
3 |
88 |
1 : 1 : 98 |
4 |
89 |
1 : 0 : 99 |
Mechanism of C3-acylation of indoles by [(4-SO3H)BMIM]HSO4 ionic liquid
Based on the previous literatures, we propose a plausible mechanism depicted in Scheme 1. First, the nucleophilic addition of indole at the C-3 position to acid anhydride in the presence of [(4-SO3H)BMIM]HSO4 provided the intermediate A. Next, the removal of carboxylic acid resulted in the formation of the intermediate B which can be subsequently undergo the elimination of proton to afford the desired product and regenerate [(4-SO3H)BMIM]HSO4 to finish the catalytic cycle.
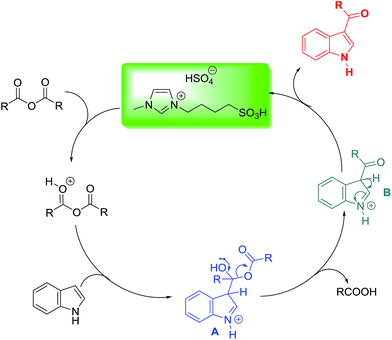 |
| Scheme 1 Proposed mechanism. | |
Comparison of [(4-SO3H)BMIM]HSO4 ionic liquid with other previous catalysts
A comparison of current work with previous literatures is presented in Table 6. The acetylation of indole with acetic anhydride in the presence of [(4-SO3H)BMIM]HSO4 provided the 3-acetylindole in higher yield within shorter reaction time. Moreover, the [(4-SO3H)BMIM]HSO4 ionic liquid was easily recovered and reused several times without decrease in catalytic activity.
Table 6 The comparative study of the current method with previous literaturesa
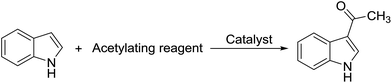
|
Entry |
Catalyst |
Acetylating reagent |
Condition |
Yield (%) |
Selectivity (1 : 2 : 3) |
1,3-Diacetylindole was obtained in 14% yield. |
1 |
ZrCl4 (1.5 equiv.) DCE, N2 |
 |
30 °C, 4 h |
67 (ref. 61) |
nd |
2 |
TfOH (0.15 equiv.) |
 |
80 °C, 24 h |
64 (ref. 17) |
nd |
3 |
Y(OTf)3 (0.01 equiv.)/[BMI]BF4 (1.0 equiv.) |
 |
80 °C, 24 h |
88 (ref. 62) |
0 : 0 : 100 |
4 |
Pd(OAc)2 (0.05 equiv.); 2,2′-bipyridine (6 mol%), CSA (0.6 mmol) |
 |
120 °C, 36 h |
84 (ref. 63) |
nd |
5 |
Hβ zeolite (0.05 g) |
 |
120 °C, 8 min |
70 (ref. 64) |
9 : 0 : 77a |
6 |
Current work |
 |
100 °C, 8 min |
90 |
|
Experimental
Chemicals, supplies, and instruments
All starting materials were purchased from Sigma-Aldrich and immediately used without further purification. Silica gel 230–400 mesh (for flash chromatography) and TLC plates were obtained from Merck. Microwave irradiation was used on a CEM Discover BenchMate. GC-MS spectra were performed on an Agilent GC System 7890 equipped with a mass selective detector Agilent 5973N. FT-IR spectra were analyzed by a Bruker Vertex 70. 1H and 13C NMR spectra were recorded on a Bruker Advance 500. HRMS (ESI) data were performed on Bruker micrOTOF-QII MS at 80 eV (please see in ESI,† Section S1).
Preparation of [(4-SO3H)BMIM]HSO4 catalyst under solvent-free sonication
The two-step procedure for the synthesis of ionic liquids from 1-methylimidazole, 1,4-butane sultone, and sulfuric acid under ultrasound irradiation were reported in the Section S2 (please see ESI†).
General procedure for Friedel–Crafts acylation of indole
Indole (1.0 mmol, 0.117 g), propionic anhydride (1.0 mmol, 0.130 g) and [(4-SO3H)BMIM]HSO4 (25 mol%) was heated under microwave irradiation at 100 °C for 5 min in a CEM Discover apparatus. The completion of the reaction was checked by TLC and GC. The mixture was then extracted with diethyl ether (5 × 5 mL). The organic layer was decanted, washed with aqueous NaHCO3 (2 × 15 mL), water (15 mL) and brine (15 mL), and dried with Na2SO4. The solvent was removed under vacuum. The crude product was purified by silica gel chromatography using ethyl acetate–hexane (1
:
9). The purified product was then characterized by 1H and 13C NMR, GC-MS or HR-MS (ESI).
Recycling of [(4-SO3H)BMIM]HSO4
The recycling of [(4-SO3H)BMIM]HSO4 was also carried out under microwave irradiation in the model reaction between indole and propionic anhydride. After completion of the reaction, diethyl ether was used to extract the reaction mixture until both starting materials and products were entirely separated from the ionic liquid. The recovered [(4-SO3H)BMIM]HSO4 with immiscible in diethyl ether was dried under vacuum at 80 °C for 60 min. The recycled [(4-SO3H)BMIM]HSO4 was used for four consecutive runs. Remarkably, the yield of the product decreased slightly after each run. The simple process of recycling [(4-SO3H)BMIM]HSO4 could be potentially developed on a large scale.
Conclusions
In summary, we have developed a green and efficient method using [(4-SO3H)BMIM]HSO4 as an effective catalyst for the highly regioselective C3-acylation of N-unsubstituted indoles with acid anhydrides. Remarkably, the accelerated microwave-assisted Friedel–Crafts acylation catalyzed by [(4-SO3H)BMIM]HSO4 was reported for the first time. Furthermore, the [(4-SO3H)BMIM]HSO4 possesses several advantages including mild preparation from commercially available materials, easy handling, and recyclability without loss of reactivity. Finally, it provides a simple, facile, and efficient alternative to the existing synthetic methods of 3-acylindoles.
Conflicts of interest
There are no conflicts to declare.
Acknowledgements
The Vietnam National Foundation for Science and Technology Development (NAFOSTED) is acknowledged for financial support through project code 104.01-2016.59. We thank Duy-Khiem Nguyen Chau, Hoang-Tan Le Doan (VNU-HCM), and Ngoc-Mai Hoang Do (IPH-HCM) for their valuable discussions.
References
- S. Bera, C. G. Daniliuc and A. Studer, Angew. Chem., Int. Ed. Engl., 2017, 56, 7402–7406 CrossRef CAS PubMed.
- S.-Y. Chou and H. J. Tsai, Drug Dev. Res., 2011, 72, 247–258 CrossRef CAS.
- M. Somei and F. Yamada, Nat. Prod. Rep., 2003, 20, 216–242 RSC.
- Y. Song, Z. Xin, Y. Wan, J. Li, B. Ye and X. Xue, Eur. J. Med. Chem., 2015, 90, 695–706 CrossRef CAS PubMed.
- E. R. El-Sawy, A. H. Mandour, S. M. El-Hallouty, K. H. Shaker and H. M. Abo-Salem, Arabian J. Chem., 2013, 6, 67–78 CrossRef CAS.
- J. R. Weng, C. H. Tsai, S. K. Kulp and C. S. Chen, Cancer Lett., 2008, 262, 153–163 CrossRef CAS PubMed.
- J. Q. Ran, N. Huang, H. Xu, L. M. Yang, M. Lv and Y. T. Zheng, Bioorg. Med. Chem. Lett., 2010, 20, 3534–3536 CrossRef CAS PubMed.
- M. Sechi, M. Derudas, R. Dallocchio, A. Dessì, A. Bacchi, L. Sannia, F. Carta, M. Palomba, O. Ragab, C. Chan, R. Shoemaker, S. Sei, R. Dayam and N. Neamati, J. Med. Chem., 2004, 47, 5298–5310 CrossRef CAS PubMed.
- L. L. Fan, Z. P. Che, R. Zhang, X. Yu, X. Y. Zhi and H. Xu, Mol. Diversity, 2012, 16, 415–421 CrossRef CAS PubMed.
- S. Ferro, M. L. Barreca, L. De Luca, A. Rao, A. M. Monforte, Z. Debyser, M. Witvrouw and A. Chimirri, Arch. Pharm., 2007, 340, 292–298 CrossRef CAS PubMed.
- S. J. Yao, Z. H. Ren, Y. Y. Wang and Z. H. Guan, J. Org. Chem., 2016, 81, 4226–4234 CrossRef CAS PubMed.
- H. Johansson, A. Urruticoechea, I. Larsen and D. Sejer Pedersen, J. Org. Chem., 2015, 80, 471–481 CrossRef CAS PubMed.
- C. Wang, S. Wang, H. Li, J. Yan, H. Chi, X. Chen and Z. Zhang, Org. Biomol. Chem., 2014, 12, 1721–1724 CAS.
- Q. Y. Lai, R. S. Liao, S. Y. Wu, J. X. Zhang and X. H. Duan, New J. Chem., 2013, 37, 4069–4076 RSC.
- Q. Shi, P. Li, X. Zhu and L. Wang, Green Chem., 2016, 18, 4916–4923 RSC.
- L. Gu, C. Jin, J. Liu, H. Zhang, M. Yuan and G. Li, Green Chem., 2016, 18, 1201–1205 RSC.
- Q. Xing, P. Li, H. Lv, R. Lang, C. Xia and F. Li, Chem. Commun., 2014, 50, 12181–12184 RSC.
- S.-J. Yao, Z.-H. Ren and Z.-H. Guan, Tetrahedron Lett., 2016, 57, 3892–3901 CrossRef CAS.
- A. C. Cole, J. L. Jensen, I. Ntai, K. L. T. Tran, K. J. Weaver, D. C. Forbes and J. H. Davis, J. Am. Chem. Soc., 2002, 124, 5962–5963 CrossRef CAS PubMed.
- J. D. Patil, S. N. Korade, S. A. Patil, D. S. Gaikwad and D. M. Pore, RSC Adv., 2015, 5, 79061–79069 RSC.
- M. A. Martins, C. P. Frizzo, A. Z. Tier, D. N. Moreira, N. Zanatta and H. G. Bonacorso, Chem. Rev., 2014, 114, PR1–PR70 CrossRef PubMed.
- B. Zhen, Q. Jiao, Y. Zhang, Q. Wu and H. Li, Appl. Catal., A, 2012, 445–446, 239–245 CrossRef CAS.
- H. Niedermeyer, J. P. Hallett, I. J. Villar-Garcia, P. A. Hunt and T. Welton, Chem. Soc. Rev., 2012, 41, 7780–7802 RSC.
- J. P. Hallett and T. Welton, Chem. Rev., 2011, 111, 3508–3576 CrossRef CAS PubMed.
- H.-P. Steinrück and P. Wasserscheid, Catal. Lett., 2014, 145, 380–397 CrossRef.
- J. Wu, Z. Lan, J. Lin, M. Huang, Y. Huang, L. Fan and G. Luo, Chem. Rev., 2015, 115, 2136–2173 CrossRef CAS PubMed.
- C. T. Li, C. P. Lee, C. T. Lee, S. R. Li, S. S. Sun and K. C. Ho, ChemSusChem, 2015, 8, 1244–1253 CrossRef CAS PubMed.
- A. N. Tran, T.-N. Van Do, L.-P. M. Le and T. N. Le, J. Fluorine Chem., 2014, 164, 38–43 CrossRef CAS.
- M. Ghavre, O. Byrne, L. Altes, P. K. Surolia, M. Spulak, B. Quilty, K. R. Thampi and N. Gathergood, Green Chem., 2014, 16, 2252–2265 RSC.
- T. Yao, S. Yao, C. Pan, X. Dai and H. Song, Energy Fuels, 2016, 30, 4740–4749 CrossRef CAS.
- J. Yin, J. Wang, Z. Li, D. Li, G. Yang, Y. Cui, A. Wang and C. Li, Green Chem., 2015, 17, 4552–4559 RSC.
- W. Zhu, C. Wang, H. Li, P. Wu, S. Xun, W. Jiang, Z. Chen, Z. Zhao and H. Li, Green Chem., 2015, 17, 2464–2472 RSC.
- Q. Bao, K. Qiao, D. Tomida and C. Yokoyama, Catal. Commun., 2008, 9, 1383–1388 CrossRef CAS.
- M. Chidambaram and A. T. Bell, Green Chem., 2010, 12, 1253–1262 RSC.
- X. Sun, Z. Liu, Z. Xue, Y. Zhang and T. Mu, Green Chem., 2015, 17, 2719–2722 RSC.
- E. Rafiee and F. Mirnezami, J. Mol. Struct., 2017, 1130, 296–302 CrossRef CAS.
- P. Fan, S. Xing, J. Wang, J. Fu, L. Yang, G. Yang, C. Miao and P. Lv, Fuel, 2017, 188, 483–488 CrossRef CAS.
- A. H. M. Fauzi and N. A. S. Amin, Renew. Sustain. Energy Rev., 2012, 16, 5770–5786 CrossRef.
- D. Z. Troter, Z. B. Todorović, D. R. Đokić-Stojanović, O. S. Stamenković and V. B. Veljković, Renew. Sustain. Energy Rev., 2016, 61, 473–500 CrossRef CAS.
- K.-S. Yeung, M. E. Farkas, Z. Qiu and Z. Yang, Tetrahedron Lett., 2002, 43, 5793–5795 CrossRef CAS.
- P. H. Tran, H. T. Nguyen, P. E. Hansen and T. N. Le, RSC Adv., 2016, 6, 37031–37038 RSC.
- A. S. Amarasekara, Chem. Rev., 2016, 116, 6133–6183 CrossRef CAS PubMed.
- X. Tong and Y. Li, ChemSusChem, 2010, 3, 350–355 CrossRef CAS PubMed.
- T. L. Greaves and C. J. Drummond, Chem. Rev., 2008, 108, 206–237 CrossRef CAS PubMed.
- M. Safa, B. Mokhtarani, H. R. Mortaheb, K. Tabar Heidar, A. Sharifi and M. Mirzaei, Energy Fuels, 2017, 31, 10196–10205 CrossRef CAS.
- S. Das, S. Santra, S. Jana, G. V. Zyryanov, A. Majee and A. Hajra, Eur. J. Org. Chem., 2017, 2017, 4955–4962 CrossRef CAS.
- J. Banothu, R. Gali, R. Velpula and R. Bavantula, Arabian J. Chem., 2017, 10, S2754–S2761 CrossRef CAS.
- S. Mahato, S. Santra, R. Chatterjee, G. V. Zyryanov, A. Hajra and A. Majee, Green Chem., 2017, 19, 3282–3295 RSC.
- H. B. Wang, N. Yao, L. Wang and Y. L. Hu, New J. Chem., 2017, 41, 10528–10531 RSC.
- M. Safa, B. Mokhtarani, H. R. Mortaheb and K. Tabar Heidar, Energy Fuels, 2016, 30, 10909–10916 CrossRef CAS.
- M. Zolfigol, A. Moosavi-Zare and E. Noroozizadeh, Synlett, 2016, 27, 1682–1684 CrossRef.
- L.-L. Lou, J. Zhang, H. Du, B. Zhao, S. Li, W. Yu, K. Yu and S. Liu, Catal. Today, 2016, 264, 109–114 CrossRef CAS.
- K. V. Wagh and B. M. Bhanage, Green Chem., 2015, 17, 4446–4451 RSC.
- J. Boulanger, A. Seingeot, B. Léger, R. Pruvost, M. Ibert, A. Mortreux, T. Chenal, M. Sauthier, A. Ponchel and E. Monflier, Catal. Commun., 2015, 69, 143–146 CrossRef CAS.
- B. M. Matsagar and P. L. Dhepe, Catal. Sci. Technol., 2015, 5, 531–539 CAS.
- M. Vafaeezadeh and H. Alinezhad, J. Mol. Liq., 2016, 218, 95–105 CrossRef CAS.
- J. Gui, X. Cong, D. Liu, X. Zhang, Z. Hu and Z. Sun, Catal. Commun., 2004, 5, 473–477 CrossRef CAS.
- J. Deng, B.-H. Xu, Y.-F. Wang, X.-E. Mo, R. Zhang, Y. Li and S.-J. Zhang, Catal. Sci. Technol., 2017, 7, 2065–2073 CAS.
- J. Lunagariya, A. Dhar and R. L. Vekariya, RSC Adv., 2017, 7, 5412–5420 RSC.
- M. Olkiewicz, N. V. Plechkova, M. J. Earle, A. Fabregat, F. Stüber, A. Fortuny, J. Font and C. Bengoa, Appl. Catal., B, 2016, 181, 738–746 CrossRef CAS.
- S. K. Guchhait, M. Kashyap and H. Kamble, J. Org. Chem., 2011, 76, 4753–4758 CrossRef CAS PubMed.
- P. H. Tran, H. N. Tran, P. E. Hansen, M. H. N. Do and T. N. Le, Molecules, 2015, 20, 19605–19619 CrossRef CAS PubMed.
- T.-S. Jiang and G.-W. Wang, Org. Lett., 2013, 15, 788–791 CrossRef CAS PubMed.
- G. Bai, T. Li, Y. Yang, H. Zhang, X. Lan, F. Li, J. Han, Z. Ma, Q. Chen and G. Chen, Catal. Commun., 2012, 29, 114–117 CrossRef CAS.
Footnote |
† Electronic supplementary information (ESI) available. See DOI: 10.1039/c7ra11362e |
|
This journal is © The Royal Society of Chemistry 2017 |
Click here to see how this site uses Cookies. View our privacy policy here.