DOI:
10.1039/C7FO01170A
(Paper)
Food Funct., 2018,
9, 320-330
Physicochemical stability, antioxidant properties and bioaccessibility of β-carotene in orange oil-in-water beverage emulsions: influence of carrier oil types
Received
31st July 2017
, Accepted 11th November 2017
First published on 13th November 2017
Abstract
This study investigated the effect of carrier oils (short-chain triglycerides (SCT) vs. long-chain triglycerides (LCT)) on the physical stability, chemical degradation, antioxidant properties and bioaccessibility of β-carotene-loaded orange oil-in-water beverage emulsions. Beverages formulated with 50% LCT (corn oil) were physically more stable compared to the ones with 50% SCT (tributyrin) as indicated by the Turbiscan 32 Stability Indices during chilled storage for 28 days. No chemical degradation of β-carotene was observed during storage regardless of the carrier oil composition. The formation of primary (conjugated dienes) or secondary (thiobarbituric acid reactive substances) oxidation products at the end of the storage period was insignificant. The bioaccessibility of β-carotene was significantly affected (P < 0.05) by the type of carrier oil and decreased in the following order: 50% LCT + 50% orange oil > 50% SCT + 50% orange oil > 100% orange oil. The high bioaccessibility of β-carotene (30.06%) in the beverages formulated with LCTs was attributed to the enhanced solubilisation capacity of the lipophilic carotenoid in mixed micelles after the in vitro digestion protocol.
1. Introduction
Carotenoids are a group of more than 600 lipophilic compounds that contribute to the yellow, orange and red colours of fruits and vegetables, and their inclusion in the diet is considered to contribute to human health and well-being.1,2 It has been demonstrated that the antioxidant properties of dietary carotenoids are associated with a reduced risk for the development of chronic diseases, such as coronary heart diseases, some types of cancers, macular degenerations and cataracts.3–5
β-Carotene is one of the major dietary carotenoids and a member of the carotenes.6 Carotenes are terpenoids with a long hydrocarbon backbone, which is synthesized biochemically in plants from isoprene units and they may or may not have oxygen atoms attached. Among the 50 carotenoids which can be metabolized into vitamin A once ingested by humans, β-carotene has the highest pro-vitamin A activity; thus, acting as a precursor of vitamin A, its consumption is important for various biological functions such as vision, gene expression, embryonic development and immune system growth.7,8 For these reasons, the food and drink industry is interested to include β-carotene in formulations in order to meet consumer demand for new food products with increased nutritional value and health-promoting properties.
The addition of β-carotene to food formulations is often problematic and comes with various challenges, mainly because of its high susceptibility to light, oxygen, temperature and pH; this practically means that depending on the processing conditions, the dispersion of β-carotene into foods can result in its rapid degradation and subsequent loss of functionality. Furthermore, β-carotene is a polyene hydrocarbon chain lacking polar groups, which renders the molecule highly insoluble in water; the lipophilic nature of β-carotene reduces its uptake at the human intestine level, which in turn limits its abundance in the systemic circulation.2,9,10 On the other hand, scientific evidence suggests that carotenoid bioavailability can increase when they are co-ingested with other lipids.11
In the past few years, researchers aimed to identify methods suitable for increasing carotenoid bioavailability, and the results based primarily on bioaccessibility studies suggested that emulsification can be an effective method.12 Emulsion-based systems are prepared by homogenizing an aqueous-phase containing an effective emulsifier, with an oil-phase in which the lipophilic bioactive compound is solubilized.13,14 In these emulsified foods, carotenoids are present within triglyceride droplets dispersed in an aqueous medium. During digestion in the gastrointestinal tract, lipids are broken down by lipases and free fatty acids are formed which are then incorporated along with bile acids and phospholipids into mixed micelles; these particular structures are capable of solubilizing and transporting carotenoids across the epithelial intestinal cells, where they are absorbed and then packed into chylomicrons that are secreted into the lymphatic system.15,16
Several studies have shown that there are many factors affecting the bioaccessibility of carotenoids; one of them is surely the total amount of fat present during digestion. It is now recognized that a higher lipid content is positively correlated with an increased carotenoid uptake.11 Furthermore, the structure of food and processing methods need to be considered for interpreting the bioaccessibility of carotenoids, since these factors determine their release from the matrix and chemical degradation.17 There is also scope to study more complex or even mixtures of food matrices in order to enhance our understanding of the digestion process of real meals. This will help identify the synergistic effects of combined foods on carotenoid bioaccessibility and will enable the design of optimum diets to improve bioavailability. The bioaccessibility of carotenoids is also dependent on the type of carrier oil. Indeed, there is substantial evidence demonstrating that the amount of carotenoids available for absorption is higher when long chain triglycerides (LCTs) are predominantly present in the oil phase. This is due to the fact that long chain fatty acids are more efficient than the short chain ones to form mixed micelles with a large hydrophobic core in which carotenoid molecules are accommodated and solubilized.18–20
The main objective of this study was to develop an edible orange oil-in-water beverage emulsion containing β-carotene, in which the dispersed phase is partially replaced by LCTs or SCTs. More particularly, we used beverage emulsions containing more than one type of oil as a model system to investigate the influence of the carrier lipid on several parameters affecting the bioaccessibility of the lipophilic bioactive compound. In particular, the specific objectives were: (1) to verify the physicochemical stability of the emulsions during four weeks of chilled storage; (2) to evaluate their antioxidant properties; and (3) to assess the bioaccessibility of β-carotene by using an in vitro gastro-intestinal digestion model. The results of this study have important implications for developing effective delivery systems of β-carotene for applications within food and beverage products which contain orange oil.
2. Materials and methods
2.1. Materials
β-Carotene was purchased from DSM Nutritional Products Ltd (Heanor, UK). Tocopherol-stripped corn oil was used as an example of a long chain triglyceride (LCT) and was purchased from Sigma-Aldrich, Co Ltd (Dorset, UK). Tributyrin and orange oil were used as the examples of short chain triglycerides (SCT) and non-digestible flavor oil, respectively, and were purchased from Sigma Aldrich (St Louis, MO, USA). Citric acid, amylase (type VI-B), pepsin, pancreatin, and bile extract were purchased from Sigma Aldrich (St Louis, MO, USA). Pure Whey Isolate TM 97 powder was used as an emulsifier and was purchased from Bulk Powders (Colchester, UK). All reagents used were of analytical grade.
2.2. Methods
2.2.1. Preparation and storage of oil-in-water (o/w) beverage emulsions.
Emulsion beverages were prepared using a standard weight-to-weight (w/w) recipe: 92% water, 3% WPI, 4% oil, 0.7% citric acid, and 0.5% β-carotene. To evaluate the effect of different carrier oil types, the oil-phases were adjusted as follows: (A) 4% orange oil, (B) 2% orange oil + 2% tributyrin (SCF) and (C) 2% orange oil + 2% corn oil (LCT). In brief, WPI was initially reconstituted in water to allow hydration for 20 min with mild agitation and then the pH was adjusted to 3.2 with citric acid. β-Carotene was added to the aqueous phase and then the mixture was agitated until dissolved. A coarse emulsion was initially formed by adding oil at a steady rate and mixing the rest of the ingredients using a high speed blender (Morphy Richards, Argos, UK) for 2 min at room temperature. Samples were then processed using a single stage valve homogenizer (APV-1000, SPX Flow Technology, West Sussex, UK). Each sample was passed two times through the homogenizer to ensure complete emulsification. The homogenization pressure was set at 40 MPa for both passages through the homogenizing valve. Three different batches for each oil type emulsion beverage (500 g) were prepared, which then were stored at 4 °C for a period of 4 weeks until further analysis (sampling was performed on a weekly basis).
2.2.2. Emulsion physical stability.
The physical stability of beverage emulsions was monitored using Turbiscan MA2000 (Formulaction, Ramonville St Agne, France) apparatus, which comprises a detection head equipped with a near-infrared light source (880 nm) which scans the length of the sample, acquiring transmission and backscattering data every 40 μm. The light source scanned the sample at 5 min intervals from the top to bottom and measured the percentage of light backscattered or transmitted during a 1 h period at 37 °C. The measured backscattering intensity is directly proportional to the photon mean free path l* which directly depends on the particle size (d), the particle concentration (Phi), the refractive index of the particles and the continuous phase (np and nf). According to Mie theory, our backscattering signal (BS) can be used to compute the particle size: BS = f (d, Phi, np, nf). The refractive indices used for the particle size calculation were 1.47 for the dispersed phase and 1.33 for the continuous phase. The Turbiscan stability index (TSI) was calculated according to the backscattering changes that indicate the particle aggregation and dynamic migration using Turbisoft 2.0.
2.2.3. Emulsion microstructure.
Light micrographs of the emulsion samples were obtained using a Leica DM IL LED inverted laboratory microscope equipped with a Leica DFC295 digital colour camera (Leica microsystems Ltd, Milton Keynes, UK). The samples were observed through a 40× dry objective lens. Pictures were taken using the in-built 3 MP digital camera and picture analysis was performed using Leica application suite software (V.3.6.0).
2.2.4. Color properties.
The color parameters L* (lightness), +a* (redness), and +b* (yellowness) were measured by using a Konica Minolta CR1 10 colorimeter (Konica Minolta Solutions Ltd, Basildon, UK). A white tile was used for standardization. Three replications were conducted.
2.2.5. Quantification of β-carotene.
A reverse phase HPLC method was used to quantify β-carotene in beverage emulsions using fluorescence and visible detection methods according to Hess et al.21 In brief, β-carotene was extracted from the oil phase as follows: 20 mg oil was mixed with 280 μL H2O and 400 μL ethanol. Each tube was vortexed for 10 seconds and 700 μL hexane (containing BHT) and 100 μL echinone were added and the samples were agitated for 10 min using a Vortex Genie mixer and then centrifuged for 5 min. The supernatant hexane layer (600 μL) was removed and dried under speed vacuum for 10 min. Each sample was then dissolved in 200 μL DEA (20% (v/v) 1.4 dioxan, 20% (v/v) ethanol, 60% (v/v) acetonitrile) and was shaken for 5–10 min before being injected for HPLC analysis. HPLC analysis was performed using a Waters 717 plus Autosampler Module (Waters Corporation, Milford, USA) equipped with a Waters 2475 scanning fluorescence detector, a 2487 UV/VIS absorbance detector and a C-18 silica (Beckman Ultrasphere ODS) analytical column (250 × 4.6 mm ID 5 μm particle size). The elution flow rate was 1.1 ml min−1, the sample run time was 30 min and the injection volume was 150 μL. Measurements were determined with mixed standards containing carotenoids and tocopherols at appropriate concentrations and the results were expressed in μg g−1 of oil. Echinone was used as an internal standard.
2.2.6. Antioxidant properties.
2.2.6.1. Ferric reducing power.
The reducing power of β-carotene beverage emulsions was measured as described by Duan et al. with slight modifications.22 One milliliter of samples was dissolved in 2.5 ml PBS buffer (0.2 M, pH 7.0), mixed with 2.5 ml potassium ferricyanide (1% w/v) and incubated at 50 °C for 20 min. At the end of the incubation period, 2.5 ml trichloroacetic acid (10% w/v) was added, and the mixtures were centrifuged at 1800g for 10 min. Two milliliters of distilled water and 0.5 ml ferric chloride (0.1% w/v) were added to the supernatant. The absorbance of the reaction mixture was determined at 700 nm after 10 min, using a UV-1600 (UV-VIS) spectrophotometer (Spectronic Camspec Ltd, Leeds, UK). Blank and control samples were prepared using water instead of potassium ferricyanide and emulsion without β-carotene, respectively.
2.2.6.2. TBARS (thiobarbituric acid reactive substances).
The lipid peroxidation inhibition capacity was determined by using TBARS. Thiobarbituric acid (1 ml of 0.34% w/v in 50% acetic acid) was added to the reaction mixture (150 μl emulsion and 4 mL dH2O) before heating the samples for 30 min in a boiling water bath (VWR International Ltd, Leicestershire, UK). The samples were allowed to cool and were centrifuged at 2465g for 15 min (Eppendorf 5810R). The serum phase was carefully sampled from the middle of the tube (100 μl) and was transferred to a black 96 well plate (Thermo Scientific) and fluorescence was measured with excitation at 515 nm and emission at 546 nm on a plate fluorometer (SpectraMax GEMINI XS, Molecular Devices Ltd, Wokingham, UK), while the concentrations of TBARs were calculated from a standard curve prepared using malonaldehyde in the range of 0 to 8 nmoles.
2.2.6.3. Conjugated dienes (CD).
The oxidative stability of the emulsions was determined by monitoring the formation of conjugated dienes.23 Emulsion samples (0.1 ml) were diluted to a final 10 ml volume in ethanol; this solution was further diluted as necessary (1
:
1) to achieve spectrophotometric readings in the target absorbance range of 0.2–0.8 at 233 nm. Ethanol was used as the blank. The absorbance at 233 nm was determined using a UV–Vis Spectrophotometer (Spectronic Camspec Ltd, Leeds, UK) as an oxidative indicator. CD levels are expressed in units of raw absorbance.
2.2.7.
In vitro gastro-intestinal digestion method.
Each emulsion was passed through a three-step in vitro digestion model that simulates oral, gastric and intestinal digestion. This method was based on the standardized static in vitro digestion model suitable for food applications with several modifications to assess carotenoid bioaccessibility.24 Simulated digestion fluids were used as follows: for the oral phase, a simulated salivary fluid (SSF) (pH 7); for the gastric phase, a simulated gastric fluid (SGF) (pH 3); and for the duodenal phase, a simulated intestinal fluid (SIF) (pH 7). Enzyme solutions were prepared using these fluids as solvents. Briefly, the protocol was as follows: to initiate the oral phase digestion process, 5 mL SSF with α-amylase (final effective concentration: 75 U mL−1) and CaCl2 solution (150 mM) was added to 5 mL emulsion sample and then the mixture was agitated in an incubator (37 °C, 100 rpm) for 2 min (Shaking Incubator, Stuart, UK). Upon the completion of the oral phase, 10 mL SGF, including porcine pepsin (final effective concentration: 2000 U mL−1) and CaCl2 (0.15 mM), was added to the sample (10 mL) from the oral phase. The pH was adjusted to 3.5 using HCl, and the mixture was incubated again for 2 h (37 °C, 100 rpm) under agitation. After 2 h, the intestinal phase digestion process was initiated by adding 20 mL SIF with an appropriate amount of enzyme stock: pancreatin (0.25 ml per ml of digesta) and bile (0.125 ml per ml of digesta). The amount of pancreatin added was based on the trypsin activity (100 U mL−1). After 30 min, the pH was monitored until it reached 7. The samples were incubated at 37 °C for 3 h, under agitation. At the end of the 3 h digestion protocol, the mixtures obtained were used to determine the bioaccessibility of β-carotene. The method adopted is suitable for the determination of free carotenoids, but additional steps are required to confirm and identify the presence of carotenoid esters after in vitro digestion.25
2.2.8. Bioaccessibility determination.
After in vitro digestion, raw digesta were collected and centrifuged (2647g; Centrifuge Eppendorf 5810R) at 25 °C for 40 min. The middle phase was assumed to consist of mixed micelles that solubilized β-carotene.26 The micelle phases were collected and prepared for HPLC analysis. Carotenoid bioaccessibility was calculated using the following equation: | Bioaccessibility (%) = (Cmicella/Craw digesta) × 100 | (1) |
where Cmicelle and Craw digesta are the β-carotene concentrations in the micelle phase and in the total digesta after in vitro digestion, respectively.
2.2.9. Statistical analysis.
Results are expressed as mean ± standard deviation (SD) of three replicates (each replicate corresponds to a different batch). Statistical analysis of the data was performed using the statistical software SPSS Statistics 22 (IBM). Data were analyzed using analysis of variance (ANOVA) and significant differences (p < 0.05) were detected using Scheffé's post hoc test.
3. Results and discussion
3.1. Impact of carrier oil on the physicochemical stability of β-carotene emulsions during storage
The influence of carrier oil types on the physicochemical stability of β-carotene enriched emulsions during 28 days of storage at 4 °C was investigated. Three different carrier oils were used to prepare the emulsions, and in particular the oil-phases were adjusted as follows: (A) 100% flavour oil (orange oil), (B) 50% flavour oil + 50% short chain triglycerides (SCT = tributyrin) and (C) 50% flavour oil + 50% long chain triglycerides (LCT = corn oil). Both SCT and LCT are digestible, whereas orange oil is indigestible.19 This is a novel approach in the fabrication of emulsions compared to the majority of the published studies, as emulsions are typically prepared using a single type of oil, rather than a mixture of two types. The Turbiscan analysis was carried out every week for a total period of 28 days of storage at 4 °C to investigate any effect due to the carrier oil on the physical stability of the beverages. Fig. 1A presents the changes in the Turbiscan stability index (TSI), which is a parameter negatively correlated to stability and takes into account all destabilization phenomena, such as gravitational separation, flocculation, coalescence and Ostwald ripening. Results indicate that the type of oil used for emulsion preparation had a significant effect on physical stability. The TSI values of emulsion C were lower than those of A and B throughout the storage period, which indicates a better stability. Particle size measurements (Fig. 1B) also suggest that emulsion C had the lowest value of particle diameter during the storage period (day 1 = 1.25 μm ± 0.14; day 28 = 1.34 μm ± 0.13). On the other hand, emulsions with SCT (B) had significantly higher particle sizes which were detected from day 1 and significantly increased during storage. These data suggest that the addition of LCT in the oil phase significantly improved the stability of orange oil beverage emulsions. The enhanced stability of emulsion C compared to the others could be attributed to the ability of corn oil to retard Ostwald ripening (OR). OR, which is commonly encountered in flavor emulsions, is the process whereby the mean size of droplets in an emulsion increases over time due to the diffusion of oil molecules from small to large droplets in the continuous phase because of the difference in the Laplace pressure.27 An increase in the mean droplet size (r) with time (t) due to OR is described by28 | 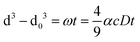 | (2) |
where ω is the OR rate, α = 2γVm/RT (where γ is the interfacial tension, Vm is the molar volume of the lipid, R is the gas constant, and T is the absolute temperature), c is the solubility of the lipid in the continuous phase, and D is the translational diffusion coefficient of the solute through the continuous phase. The aqueous phase solubility of an oil decreases linearly with the oil molar volume, Vm.29 These destabilization phenomena occur frequently in the emulsions prepared using oils with an appreciable water-solubility, such as short-chain triglycerides and flavor or essential oils and are manifested by the formation of a clear layer of oil on the top of the emulsion (emulsion B). Tributyrin is a relatively small nonpolar molecule (low molar volume), with high water solubility and is therefore highly prone to OR.30 On the other hand, the addition of oils containing LCTs with a large molar volume (∼900 cm3 mol−1) and a relatively low water solubility offers a protective effect against OR. Thus, a popular approach to improve the stability of emulsions containing SCT or flavor oils is to add a ripening inhibitor, which is typically a high molecular weight, nonpolar molecule insoluble in the aqueous phase, such as corn oil. Recently, it has been demonstrated that corn oil can act as a ripening inhibitor and retard OR when it is incorporated into the oil phase prior to homogenization.31,32 Furthermore, it was demonstrated that it is sufficient to incorporate ≥10% corn oil to inhibit OR in emulsion-systems. This effect is attributed to an entropy of mixing effect that opposes OR due to differences in curvature.33 Therefore, it is possible that the partial (50%) substitution of orange oil with corn oil (LCT) inhibited or prevented OR. Conversely, both A and B contain lipid phases with high water solubility and therefore are more susceptible to OR. Partial coalescence induced by the crystallization of fat droplets during storage at 4 °C may also account for the increased particle size of emulsion B.34 The conditional presence of a potential crystal seeding material (i.e. carotene) may further enhance such effects. Interestingly, the results of this study indicate similar particle size measurements for emulsions C and A. Thus, other destabilization phenomena (i.e. creaming) may also account for the TSI values obtained for emulsion A (100% orange oil). A previous study reported similar findings and the instability of the flavor emulsion was attributed to gravitational separation due to the low density of orange oil (844 kg m−3) compared to water (998 kg m−3) leading to rapid upward migration of oil droplets.31 Thus, the addition of corn oil may also retard creaming since the lipid phase density (900 kg m−3) is closer to the aqueous phase density. There was no evidence for flocculation in any of the beverages, as indicated by the microscopic analysis of the samples (Fig. 1).
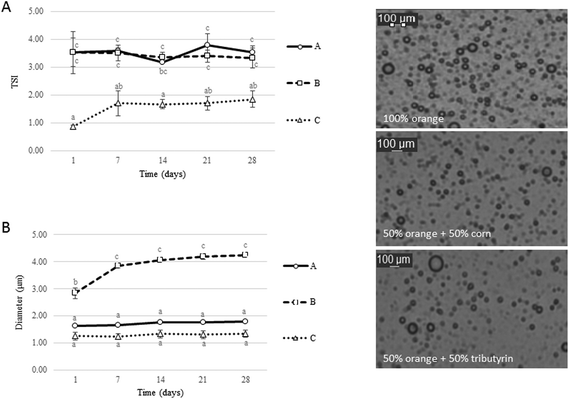 |
| Fig. 1 Changes in TSI (A) and particle diameter (B) values during 28 days of chilled storage and bright field optical microscopy images of emulsion beverages formulated with: (A) 100% orange oil; (B) 50% orange oil + 50% SCT; and (C) 50% orange oil + 100% LCT. Different small letters denote significant differences (P < 0.05) between samples. Bars represent the standard deviation of the mean. | |
Considering the effect of storage at 4 °C for 28 days, the increase of TSI values was insignificant (P < 0.05). This is an important finding regarding the shelf-life of the beverages, because all of the emulsions remained fairly stable during the storage period. The exception was emulsion B, which demonstrated a significant increase in particle size diameter between day 1 and day 28. However, this effect was not reflected on the corresponding TSI values. Cornacchia and Roos investigated the stability of β-carotene emulsions during 28 days of storage at 15 °C and reported similar findings.35 In the latter case, emulsions were also prepared using a similar amount of carotenoids and type of emulsifier (WPI) and were physically stable over the whole period of observation. To prepare a stable emulsion for a defined period of time the most important factors to consider are: the type of emulsifier, the homogenization conditions, the droplets’ size, the droplet's charge and the storage conditions. Hence, the emulsifier type and concentration, the homogenization process and the chilled storage conditions adopted here were suitable for fabricating stable emulsions for a reasonable period of time for commercial applications using three different oil phases (singly or in combination).
3.2. Influence of carrier oil on colour properties
The nature of the carrier oil also had an appreciable but not significant impact on the color of the beverages. This is an important parameter which needs to be taken into consideration when developing a new product or reformulating an existing one, since it is a major determinant of shelf-life and consumer acceptance. Emulsion beverages prepared with 100% orange oil appear to have a light orange colour, whereas the addition of SCT or LCT has a darkening effect (Table 1). Visual observations are also reflected on the color parameters evaluated by the Hunter Lab system. The partial replacement (50%) of orange oil with tributyrin or corn oil decreased the lightness (L*) and slightly lowered the redness and yellowness values as well. Emulsion beverages containing SCT (B) and LCT (C) were very similar and differences were not noticeable between them. These results showed that different carrier oils affect the colours of the beverages and as a result have an impact on the sensory properties of the end product; thus, it is a critical factor to take into account when designing emulsion delivery systems for lipophilic compounds.
Table 1 Photographs and L*, b*, and a* colour values of β-carotene enriched emulsions with different carrier oils: (A) orange oil; (B) orange oil + SCT; and (C) orange oil + LCT. Results are presented as means of replicates ± SD
|
Colour values |
Emulsion |
L* |
a* |
b* |
Differences at a significance level (p < 0.05) were not detected between samples. |
A |
|
5.0 ± 0.9 |
5.2 ± 0.9 |
5.7 ± 0.3 |
B |
|
2.8 ± 0.9 |
4.6 ± 0.1 |
4.0 ± 0.2 |
C |
|
2.5 ± 0.4 |
4.6 ± 1.1 |
4.2 ± 1.0 |
3.3. β-Carotene stability during storage
β-Carotene features a characteristic conjugated polyene chain with two identical rings at each end. Polyenes are poly-unsaturated organic compounds which renders β-carotene susceptible to oxidation. Consequently, the organic molecule may degrade during storage, leading to the loss of its bioactive properties and affecting the quality of the food-system.9 In order to verify the stability of β-carotene in the emulsions, we quantified the carotenoid content of the beverages on a weekly basis for a total period of 28 days of chilled storage using an HPLC method. Data are reported in Table 2. Results indicate that for all beverage emulsions the β-carotene content remained unchanged (P > 0.05) during the overall period of storage. Moreover, no significant differences between the three types of emulsions were detected; this suggests that the stability of β-carotene was independent from the type of carrier oil used. Thus, β-carotene was highly stable when incorporated into all beverage-emulsion systems and was not degraded; in fact, the β-carotene concentration remained essentially the same during 28 days of storage. A possible explanation of this result is that the carotenoid molecules were probably located far from the interface; hence, they were protected from oxidation. Cornacchia and Roos investigated β-carotene stability during storage by comparing oil-in-water systems with solid or liquid dispersed lipid particles and reported similar findings.36 They concluded that the physical structure of the dispersed carrier affected the stability of β-carotene, which was higher for the emulsion with a liquid carrier. It was shown that β-carotene, when incorporated into emulsions with solid lipid particles, was concentrated into physical domains located at the oil/water interface, where it was more susceptible to degradation by the reactive species present in the aqueous phase. Storage conditions can also have an impact on the degradation rate of β-carotene in nanoemulsions. Luo et al. demonstrated that chemical degradation was prevented under storage conditions (4 °C, 14 days) similar to the ones of the present study.37 However, the degradation rate increased considerably with increasing storage temperature, particularly at 55 °C. Numerous other factors are known to confer protection against oxidation in a emulsion-based food system including: the charge of emulsion droplets, the capacity of proteins to chelate metal ions and to react with primary and secondary products of oxidation, the structural characteristics of the oil/water interface and the physical state of lipids.35,38 Previous studies have demonstrated the ability of β-lactoglobulin to prevent the degradation of β-carotene in nanoemulsions.39 A number of reasons may account for the stabilising effect exerted by proteinaceous emulsifiers including their ability to scavenge free radicals at the interface and the formation of complexes with carotenoids through hydrophobic interactions.40,41 In addition, the emulsifier type and concentration used (3% WPI) may also create a physical barrier that protects β-carotene against oxidative deterioration. Furthermore, it should be considered that, as previously discussed (3.1), all of the emulsions were physically stable during the overall time of storage, which could be another preventative factor against oxidation and consequently further limiting the degradation of carotenoids. The results demonstrate that the recipe and the conditions used in this study for the fabrication of the beverage emulsions were suitable to optimize the stability of β-carotene during 28 days of storage.
Table 2 Determination of β-carotene levels (μg ml−1) in beverage emulsions during storage at 4 °C for a period of 28 days. Results are presented as means of replicates ± SD
|
Days of storage at 4 °C |
|
1 |
7 |
14 |
21 |
28 |
Differences at a significance level (p < 0.05) were not detected between samples. |
A |
497.5 ± 28.5 |
476.4 ± 14.0 |
537.1 ± 16.0 |
524.6 ± 19.4 |
472.3 ± 29.8 |
B |
502.4 ± 20.9 |
546.2 ± 19.7 |
467.4 ± 46.1 |
499.7 ± 45.6 |
485.0 ± 35.8 |
C |
508.2 ± 9.2 |
491.8 ± 1.8 |
489.0 ± 12.2 |
481.6 ± 14.8 |
487.0 ± 6.3 |
3.4. Antioxidant properties of β-carotene beverage emulsions
3.4.1. Reducing power.
In addition to formulating stable emulsions and verifying their physicochemical parameters, the second aim of this study was to evaluate the antioxidant properties of the emulsions. Since β-carotene is used in the fabrication of beverages to improve their nutritional value, it is important to assess the antioxidant properties of the emulsion-systems. Firstly, the reducing power of the emulsions was determined (Fig. 2). This spectrophotometric method has been used in the past for measuring the antioxidant properties of emulsions formulated with carotenoids.42 It offers the advantage that measurements are taken at 700 nm, which is fairly distant from the absorption intensity of β-carotene (360–540 nm) and thus reduces the risk of spectral interference. The method was validated by determining the ferric reducing power of a control emulsion (D). The control emulsion was prepared using the same recipe without adding β-carotene. The principle of this method lies in the increase of the absorbance of the reaction mixtures after a certain incubation period: an increase in the absorbance indicates an increment of the antioxidant activity. All beverage emulsions showed a significantly higher antioxidant capacity than the control, demonstrating an effective antioxidant activity of the encapsulated β-carotene. The emulsions had a high potency to convert free radicals into stable products, which suggests that the solubility and dispersibility of β-carotene is enhanced by emulsion encapsulation. Furthermore, there was a significant difference between the emulsions formulated with different carrier oils. Since all beverages contained the same β-carotene levels and oil phases were stripped from any natural antioxidant present, any significant effect on the antioxidant properties of the beverages is attributed to the oil composition. Emulsion B, which was the one that contained SCT (tributyrin), demonstrated the highest reducing power value (P < 0.05). Possibly, this is due to the different fatty acid compositions of the oil phases of the emulsions. Long-chain polyunsaturated fatty acids in corn oil are more susceptible to oxidation phenomena, since they contain more bis-allylic C–H bonds in their hydrocarbon chain.43 In contrast, short chain triglycerides with a higher degree of saturation are more stable, indicating that β-carotene can be more effective when dispersed in this type of oil phase.
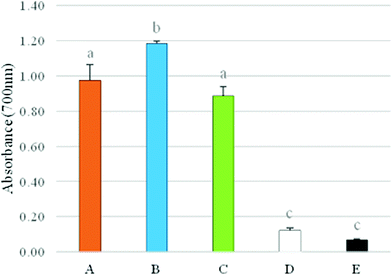 |
| Fig. 2 Reducing power as an indicator of the antioxidant capacity of β-carotene beverage emulsions formulated with: (A) 100% orange oil; (B) 50% orange oil + 50% SCT; (C) 50% orange oil + 50% LCT; (D) emulsion without β-carotene; and (E) water (blank). Different small letters denote significant differences (P < 0.05) between samples. Bars represent the standard deviation of the mean. | |
3.4.2. Lipid peroxidation.
Lipid oxidation is one of the most critical causes of quality deterioration in food and beverages. In this study, we evaluated the lipid peroxidation inhibition capacity of β-carotene enriched emulsions using two different spectrophotometric methods, which included the determination of TBARS and CD in beverage emulsions. The formation of TBARS and CD was determined at day 1 and after 28 days of storage at 4 °C. The aim was to investigate whether the emulsions may inhibit the formation of lipid peroxidation products during storage, which is a critical factor affecting the quality of the beverage and consumer acceptability.
Non-conjugated double bonds are converted into conjugated dienes (CD) almost immediately after peroxides are formed. The measurement of CD at 233 nm is one of the main methods of monitoring lipid oxidation in emulsion systems. The method's preparation time is relatively short, and the method is not dependent on chemical reactions or colour development and requires a small amount of sample.23 As shown in Fig. 3A, no significant differences were detected between samples for CD. CD values were not higher after 28 days of storage for all beverages, which indicates that they were stable under the specified storage conditions. This means that β-carotene exerted its antioxidant activity in the emulsion-systems and conferred protection against lipid peroxidation. CD values were determined in emulsions containing carotenoids by Kiokias and Oreopoulou.23 In the latter study, emulsion samples were held in a shaking water bath and were allowed to oxidize at 60 °C for 4 hours; furthermore, a radical initiator (2,2′-azobis(2-amidinopropane) dihydrochloride) was added to the reaction mixture to enhance the process of lipid peroxidation. It was shown that the control emulsions showed a faster increase in absorbance with storage time; therefore the tested carotenoids were able to inhibit the AAPH-initiated oxidation. The results of the present study are expected considering the processing and storage conditions of the samples. The beverages were stored at cold temperatures for a short period of time. Furthermore, it is likely that the relatively low amount of carrier oil (4%) in the absence of a radical initiator prevented the formation of peroxyl radicals.
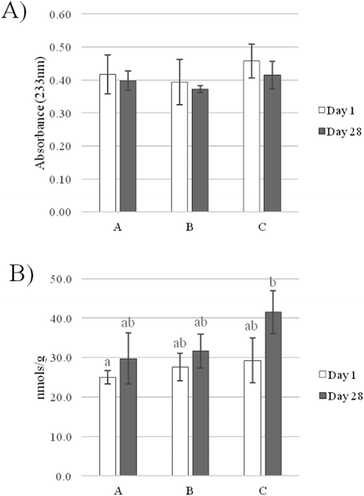 |
| Fig. 3 Influence of carrier oil types on the lipid peroxidation inhibition capacity of β-carotene beverage emulsions. Changes in CD (A) and TBARS values (B) for a total period of 28 days of chilled storage. Different small letters denote significant differences (P < 0.05) between samples. Bars represent the standard deviation of the mean. | |
Thiobarbituric acid reactive substances (TBARS) are the secondary products of lipid peroxidation formed by the degradation of lipid hydroperoxides. Emulsion A at day 1 had the lowest TBARS concentration, whereas emulsion C had the highest values after 28 days of storage (Fig. 3B). TBARS levels were similar (P > 0.05) for all beverages at day 1. Furthermore, the levels increased (P > 0.05) for all emulsions during storage and this effect was slightly more noticeable for emulsion C. However, no significant differences with respect to TBARS formation were detected between samples after 28 days of storage. In agreement with the results on CD, there was no indication of lipid peroxidation occurring in all beverage formulations. As previously discussed (3.2), β-carotene was not degraded during storage, which might be an additional factor preventing the process of lipid peroxidation. Ensuring the chemical stability of bioactive compounds (i.e. antioxidants) during processing and storage in foods and beverages is a prerequisite for designing delivery systems of functional ingredients.9 Furthermore, WPI may also have contributed to the oxidative stability of the beverages by forming an interfacial physical and electrostatic barrier to iron and other pro-oxidants that are common to the aqueous phase. Previous research has indicated that the protective layer formed by protein emulsifiers may be hindering the radical scavenging activity of β-carotene in sunflower oil-in-water emulsions.44 The results obtained clearly suggest that the formulation used for emulsion fabrication and the processing and storage conditions of the beverages are adequate to inhibit lipid peroxidation and ensure oxidative stability during shelf-life.
3.5. Influence of the oil composition on β-carotene bioaccessibility
The influence of the carrier oil composition on β-carotene bioaccessibility was determined using an in vitro gastro-intestinal digestion model. Emulsion samples were consequentially digested in the simulated oral, gastric and intestinal phases. After the intestinal stage, the digesta obtained were centrifuged to separate the micelle phase (middle layer), which contained the solubilized fraction of β-carotene. The bioaccessibility of β-carotene was determined by measuring the β-carotene concentration in the micelle phase and the total digesta.26 Data are presented in Fig. 4 and indicate that the bioaccessibility of β-carotene was significantly affected by the type of carrier oil present in the beverages. The bioaccessibility value of β-carotene was 6% for beverages formulated exclusively with orange oil and increased to 10% and 30% when the latter phase was partially substituted (50%) with SCT and LCT, respectively. The results of this study are in agreement with previously published data. Qian et al. showed that the bioaccessibility of β-carotene clearly depends on the type of carrier lipid present in a nanoemulsion delivery system.19 Orange oil, MCT (medium chain triglycerides) and corn oil were used to form nanoemulsions and according to their findings the highest bioaccessibility was demonstrated by the samples formulated with corn oil (66%). The bioaccessibility of β-carotene for the other carrier oil types was considerably low (<15%), which was further reduced upon the filtration of the samples.19 Another study investigated the effect of the carrier oil composition on the bioaccessibility of β-carotene by comparing emulsions with different MCT
:
LCT ratios. A significant increase in β-carotene bioaccessibility was observed as the fraction of LCT within the oil phases increased.20 Specifically, the bioaccessibility of β-carotene increased from 14% to 86% as the LCT fraction in the oil phase increased from 0% to 100%. Similar findings were reported in a study which investigated the impact of lipid phase types (medium or long chain triacylglycerides) on the β-carotene delivery efficacy of excipient nanoemulsion using dietary supplements (tablet or soft gel formulations). In agreement with the previous findings, the study concluded that using LCT as a lipid phase significantly increased β-carotene bioaccessibility in both types of dietary supplements.45
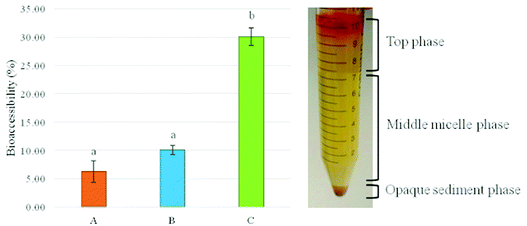 |
| Fig. 4 Bioaccessibility (%) of β-carotene beverage emulsions formulated with different carrier oils: (A) 100% orange oil; (B) 50% orange oil + 50% SCT; and (C) 50% orange oil + 50% LCT. Different small letters denote significant differences (P < 0.05) between samples. Bars represent the standard deviation of the mean. | |
In view of the findings described above, it is possible to explain our results as follows. Firstly, bioaccessibility was very low in emulsion A, which contains 100% of orange oil. Orange oil is an indigestible oil, which lacks triacylglycerol components and therefore cannot be digested into free fatty acids by lipases. However, this step is essential for the formation of mixed micelles by bile salts and lipolysis products which contain the lipophilic carotenoid molecules.16 As a result, β-carotene is not adequately solubilized in mixed micelles when orange oil is exclusively used for fabricating the beverage emulsions, which results in low bioaccessibility values. The bioaccessibility of β-carotene increased when orange oil was partially substituted with digestible oils. Emulsion C, which contains 50% LCT, showed the highest bioaccessibility. Our results are in agreement with those of previous studies. Rao et al. reported that β-carotene bioaccessibility depended on the type of oil present and increased in the presence of triglyceride oils.26 Interestingly, the results are comparable with the ones of the current study. The bioaccessibility values were ∼35% for nanoemulsions containing equal amounts of lemon and corn oil. This effect was attributed to the ability of digestible oils to increase the number of mixed micelles capable of solubilising β-carotene. On the other hand, the bioaccessibility values for emulsion B with 50% SCT remained low. A proposed mechanism which may justify the fact that the bioaccessibility of β-carotene was significantly higher for the emulsions containing LCT rather than SCT is extensively documented. The solubilisation capacity of a lipophilic compound into mixed micelles tends to increase with increasing fatty acid chain length.46 It has been hypothesized that long chain triglycerides (i.e. present in corn oil) are capable of forming mixed micelles that have larger hydrophobic cores than those formed by SCT. Accordingly, the micelles formed by LCT contain hydrophobic domains large enough to accommodate the linear, rod-like, hydrophobic β-carotene molecules. A number of studies suggest that the colloidal structures formed by the digestion of long chain triglycerides are more efficient to accommodate large lipophilic compounds than those formed by the medium or short chain ones, which in turn determined the solubilisation capacity.19,20,26,45 The degree of fatty acid saturation can also determine the gastrointestinal fate of β-carotene and thus affects its bioaccessibility.47 Corn oil contains significant amounts of polyunsaturated and monounsaturated long chain fatty acids (i.e. linoleic acid, oleic acid), which is deemed favorable for β-carotene bioaccessibility.48,49 Furthermore, other factors may also determine the solubilisation capacity of β-carotene in mixed micelles such as the initial particle size of the emulsion. A strong correlation between the initial droplet size and β-carotene bioaccessibility has been reported.50 This may be explained in terms of the high digestion rate of small droplets within the gastrointestinal tract due to their large surface areas accessible to digestive enzymes. In the present study, beverage emulsions with 50% corn oil also demonstrated the smallest particle size, which may have contributed to the enhanced carotenoid bioaccessibility. Thus, the type of carrier oil has a strong influence on the bioaccessibility of β-carotene. Therefore, depending on the application and the desired properties of the final product, the selection of the oil phase is a critical step for the development of an efficacious emulsion delivery system capable of encapsulating carotenoids that provide improved health benefits to consumers.
4. Conclusions
This study shows that the type of carrier oil has a significant impact on the physicochemical properties of β-carotene-loaded beverage emulsions formulated with orange oil. The inclusion of LCTs enhanced the physical stability of the beverages by retarding Ostwald ripening and creaming phenomena. β-Carotene was stable during refrigerated storage for 28 days regardless of the composition of the carrier oil, suggesting that the processing and storage conditions are suitable for the fabrication of an effective delivery system of the bioactive carotenoid. There was no noticeable effect on the oxidative stability of the beverages caused by the carrier oil or the length of storage. The mild storage conditions and the inclusion of a proteinaceous emulsifier may also have contributed to the increased chemical and oxidative stability of the beverage emulsions. This further suggests that the potential sensory performance and shelf-life of the beverage remains unaffected. The bioaccessibility of β-carotene was significantly increased with the inclusion of LCTs in the formulation. The findings of this study highlight the importance of the formulation strategy when designing emulsion-based delivery systems and have useful applications for the food and beverage industries. Further studies to elucidate the effect of carrier oils on the bioavailability of β-carotene using in vivo models (cells, animals or humans) are required.
Conflicts of interest
The authors declare that there are no conflicts of interest.
Acknowledgements
This work was a part of the Strategic Research Programme 2016–2021 and was funded by the Scottish Government's Rural and Environment Science and Analytical Services Division (RESAS).
References
- G. Britton, Structure and properties of carotenoids in relation to function, FASEB J., 1995, 9, 1551 CAS.
- J. J. M. Castenmiller and C. E. West, Bioavailability and bioconversion of carotenoids, Annu. Rev. Nutr., 1998, 18, 19 CrossRef CAS PubMed.
- S. Kiokias and M. H. Gordon, Antioxidant properties of carotenoids in vitro and in vivo, Food Rev. Int., 2007, 20, 99 CrossRef.
- N. I. Krinsky and E. J. Johnson, Carotenoid actions and their relation to health and disease, Mol. Aspects Med., 2005, 26, 459 CrossRef CAS PubMed.
- S. A. Paiva and R. M. Russel, β-Carotene and other carotenoids as antioxidants, J. Am. Coll. Nutr., 1999, 18, 426 CrossRef CAS PubMed.
- G. Maiani, M. J. Castón, G. Catasta, E. Toti, I. G. Cambrodón, A. Bysted, F. Granado-Lorencio, B. Olmedilla-Alonso, P. Knuthsen, M. Valoti, V. Böhm, E. Mayer-Miebach, D. Behsnilian and U. Schlemmer, Carotenoids: actual knowledge on food sources, intakes, stability and bioavailability and their protective role in humans, Mol. Nutr. Food Res., 2009, 53, 194 Search PubMed.
-
National Academy of Sciences, Institute of Medicine, Food and Nutrition Board, Vitamin A. Dietary Reference Intakes for Vitamin A, Vitamin K, Arsenic, Boron, Chromium, Copper, Iodine, Iron, Manganese, Molybdenum, Nickel, Silicon, Vanadium, and Zinc, National Academy Press, Washington, DC, 2001, pp. 82–161 Search PubMed.
- H. Sies, W. Stahl and A. R. Sundquist, Antioxidant functions of vitamins. Vitamins E and C, beta-carotene, and other carotenoids, Ann. N. Y. Acad. Sci., 1992, 30, 7 CrossRef.
- C. S. Boon, D. J. McClements, J. Weiss and E. A. Decker, Factors influencing the chemical stability of carotenoids in foods, Crit. Rev. Food Sci. Nutr., 2010, 50, 515 CrossRef CAS PubMed.
- G. Zakynthinos and T. Varzakas, Carotenoids: From Plants to Food Industry, Curr. Res. Nutr. Food Sci., 2016, 4, 38 CrossRef.
- M. J. Brown, M. G. Ferruzzi, M. L. Nguyen, D. A. Cooper, A. L. Eldridge, S. J. Schwartz and W. S. White, Carotenoid bioavailability is higher from salads ingested with full-fat than with fat-reduced salad dressings as measured with electrochemical detection, Am. J. Clin. Nutr., 2004, 80, 396 CAS.
- D. J. McClements, E. A. Decker, Y. Park and J. Weiss, Structural design principles for delivery of bioactive components in nutraceuticals and functional foods, Crit. Rev. Food Sci. Nutr., 2009, 49, 577 CrossRef CAS PubMed.
- M. J. McClements, Emulsion design to improve the delivery of functional lipophilic components, Annu. Rev. Food Sci. Technol., 2010, 1, 241 CrossRef PubMed.
- V. Raikos and V. Ranawana, Designing emulsion droplets of foods and beverages to enhance delivery of lipophilic bioactive components – a review of recent advances, Int. J. Food Sci. Technol., 2017, 52, 68 CrossRef CAS.
- V. Tyssandier, B. Lyan and P. Borel, Main factors governing the transfer of carotenoids from emulsion lipid droplets to micelles, Biochim. Biophys. Acta, Mol. Cell Biol. Lipids, 2001, 1533, 285 CrossRef CAS.
- L. Yonekura and A. Nagao, Intestinal absorption of dietary carotenoids, Mol. Nutr. Food Res., 2007, 51, 107 CAS.
- T. Bohn, G. J. McDougall, A. Alegría, M. Alminger, E. Arrigoni, A.-M. Aura, C. Brito, A. Cilla, S. N. El, S. Karakaya, M. C. Martínez-Cuesta and C. N. Santos, Mind the gap – deficits in our knowledge of aspects impacting the bioavailability of phytochemicals and their metabolites – a position paper focusing on carotenoids and polyphenols, Mol. Nutr. Food Res., 2015, 59, 1307–1323 CAS.
- T. Huo, M. G. Ferruzzi, S. J. Schwartz and M. L. Failla, Impact of fatty acyl composition and quantity of triglycerides on bioaccessibility of dietary carotenoids, J. Agric. Food Chem., 2007, 55, 8950 CrossRef CAS PubMed.
- C. Qian, E. A. Decker, H. Xiao and D. J. McClements, Nanoemulsion delivery systems: Influence of carrier oil on b-carotene bioaccessibility, Food Chem., 2012, 135, 1440 CrossRef CAS PubMed.
- L. Salvia-Trujillo, C. Qian, O. Martín-Belloso and D. J. McClements, Modulating β-carotene bioaccessibility by controlling oil composition and concentration in edible nanoemulsions, Food Chem., 2013, 15, 878 CrossRef PubMed.
- D. Hess, H. E. Keller, B. Oberlin, R. Bonfantim and W. Schüep, Simultaneous determination of retinol, tocopherols, carotenes and lycopene in plasma by means of high-performance liquid chromatography on reverse phase, Int. J. Vitam. Nutr. Res., 1991, 61, 232 CAS.
- X. Duan, M. Li, H. Ma, X. Xu, Z. Jin and X. Liu, Physicochemical properties and antioxidant potential of phosvitin-resveratrol complexes in emulsion system, Food Chem., 2016, 1, 102 CrossRef PubMed.
- S. Kiokias and V. Oreopoulou, Antioxidant properties of natural carotenoid extracts against the AAPH-initiated oxidation of food emulsions, Innovative Food Sci. Emerging Technol., 2006, 7, 132 CrossRef CAS.
- M. Minekus, M. Alminger, P. Alvito, S. Balance, T. Bohn, C. Bourlieu, F. Carrière, R. Boutrou, M. Corredig, D. Dupont, C. Dufour, L. Egger, M. Golding, S. Karakaya, B. Kirkhus, S. Le Feunteun, U. Lesmes, A. Macierzanka, A. Mackie, S. Marze, D. J. McClements, O. Ménard, I. Recio, C. N. Santos, R. P. Singh, G. E. Vegarud, M. S. Wickham, W. Weitschies and A. Brodkorb, A standardized static in vitro digestion method suitable for food - an international consensus, Food Funct., 2014, 5, 1113 CAS.
- D. B. Rodrigues, L. R. B. Mariutti and A. Z. Mercadante, An in vitro digestion method adapted for carotenoids and carotenoid esters: moving forward towards standardization, Food Funct., 2016, 7, 4992 CAS.
- J. Rao, E. A. Decker, H. Xiao and D. J. McClements, Nutraceutical nanoemulsions: influence of carrier oil composition (digestible versus indigestible oil) on β-carotene bioavailability, J. Sci. Food Agric., 2013, 93, 3175 CrossRef CAS PubMed.
- A. S. Kabalnov and E. D. Shchukin, Ostwald ripening theory-applications to fluorocarbon emulsion stability, Adv. Colloid Interface Sci., 1992, 38, 69 CrossRef CAS.
- P. Taylor, Ostwald ripening in emulsions, Adv. Colloid Interface Sci., 1998, 75, 107 CrossRef CAS.
- P. Taylor, Ostwald ripening in emulsions: estimation of solution thermodynamics of the disperse phase, Adv. Colloid Interface Sci., 2003, 106, 261 CrossRef CAS PubMed.
- T. J. Wooster, M. Golding and P. Sanguansri, Impact of oil type on nanoemulsion formation and Ostwald ripening stability, Langmuir, 2008, 24, 12758 CrossRef CAS PubMed.
- D. J. McClements, L. Henson, L. M. Popplewell, E. A. Decker and S. J. Choi, Inhibition of Ostwald ripening in model beverage emulsions by addition of poorly water soluble triglyceride oils, J. Food Sci., 2012, 71, C33 CrossRef PubMed.
- Y. Li, S. Le Maux, H. Xiao and D. J. McClementsm, Emulsion-based delivery systems for tributyrin, a potential colon cancer preventive agent, J. Agric. Food Chem., 2009, 57, 9243 CrossRef CAS PubMed.
- A. S. Kabalnov, A. V. Pertzov and A. D. Shchukin, Ostwald ripening in 2-component disperse phase systems-application to emulsion stability, Colloids Surf., 1987, 24, 19 CrossRef CAS.
- B. M. Degner, C. Chung, V. Schlegel, R. Hutkins and D. J. McClements, Factors influencing the freeze-thaw stability of emulsion-based foods, Compr. Rev. Food Sci. Food Saf., 2014, 13, 98 CrossRef CAS.
- L. Cornacchia and Y. H. Roos, Stability of β-carotene in protein-stabilized oil-in-water delivery systems, J. Agric. Food Chem., 2011, 59, 7013 CrossRef CAS PubMed.
- L. Cornacchia and Y. H. Roos, State of dispersed lipid carrier and interface composition as determinants of beta-carotene stability in oil-in-water emulsions, J. Food Sci., 2011, 78, C1211 CrossRef PubMed.
- X. Luo, Y. Zhou, L. Bai, F. Liu, Y. Deng and D. J. McClements, Fabrication of β-carotene nanoemulsion-based delivery systems using dual-channel microfluidization: Physical and chemical stability, J. Colloid Interface Sci., 2017, 490, 328 CrossRef CAS PubMed.
- M. Hu, D. J. McClements and E. A. Decker, Lipid oxidation in corn oil-in-water emulsions stabilized by casein, whey protein isolate, and soy protein isolate, J. Agric. Food Chem., 2003, 51, 1696 CrossRef CAS PubMed.
- C. Qian, E. A. Decker, H. Xiao and D. J. McClements, Inhibition of β-Carotene degradation in oil-in-water nanoemulsions: Influence of oil-soluble and water-soluble antioxidants, Food Chem., 2012, 135, 1036 CrossRef CAS PubMed.
- L. M. Tong, S. Sasaki, D. J. McClements and E. A. Decker, Mechanisms of the antioxidant activity of a high molecular weight fraction of whey, J. Agric. Food Chem., 2000, 48, 1473 CrossRef CAS PubMed.
- H. Wackerbath, T. Stoll, S. Gebken, C. Pelters and U. Bindrich, Carotenoid-protein interaction as an approach for the formulation of functional food emulsions, Food Res. Int., 2009, 42, 1254 CrossRef.
- C. Tan, J. Xue, S. Abbas, B. Feng, X. Zhang and S. Xia, Liposome as a delivery system or carotenoids: comparative antioxidant activity of carotenoids as measured by ferric reducing antioxidant power, DPPH assay and lipid peroxidation, J. Agric. Food Chem., 2014, 62, 6726 CrossRef CAS PubMed.
- C. C. Berton-Carabin, M. H. Ropers and C. Genot, Lipid oxidation in oil-water-emulsions: Involvement of the interfacial layer, Compr. Rev. Food Sci. Food Saf., 2014, 13, 945 CrossRef CAS.
- C. Dimakou and V. Oreopoulou, Antioxidant activity of carotenoids against the oxidative destabilization of sunflower oil-in-water emulsions, LWT–Food Sci. Technol., 2012, 46, 393 CrossRef CAS.
- L. Salvia-Trujillo and D. J. McClements, Improvement of β-carotene bioaccessibility from dietary supplements using excipient nanoemulsions, J. Agric. Food Chem., 2016, 64, 4639 CrossRef CAS PubMed.
- J. Ø. Christensen, K. Schultz, B. Mollgaard, H. G. Kristensen and A. Mullertz, Solubilisation of poorly-water soluble drugs during in vitro lipolysis of medium- and long-chain triacylglycerols, Eur. J. Pharm. Sci., 2004, 23, 287 CrossRef CAS PubMed.
- J. Corte-Real, E. Richling, L. Hoffmann and T. Bohn, Selective factors governing in vitro β-carotene bioaccessibility: negative influence of low filtration cutoffs and alterations by emulsifiers and food matrices, Nutr. Res., 2014, 34, 1101 CrossRef CAS PubMed.
- A. Alvarez-Sala, G. Garcia-Llatas, A. Cilla, R. Barberá, L. M. Sánches-Siles and M. J. Lagarda, Impact of lipid components and emulsifiers on plant sterols bioaccessibility from milk-based fruit beverages, J. Agric. Food Chem., 2016, 64, 5686 CrossRef CAS PubMed.
- S. R. Goltz, W. W. Campbell, C. Chitchumroonchokchai, M. L. Failla and M. G. Feruzzi, Meal triacylglycerol profile modulates post-prandial absorption of carotenoids in humans, Mol. Nutr. Food Res., 2012, 56, 866 CAS.
- L. Salvia-Trujillo, S. H. E. Verkenmpinck, L. Sun, A. M. Van Loeym, T. Grauwet and M. E. Hendrickx, Lipid digestion, micelle formation, and carotenoid bioaccessibility kinetics: Influence of emulsion droplet size, Food Chem., 2017, 229, 653 CrossRef CAS PubMed.
|
This journal is © The Royal Society of Chemistry 2018 |
Click here to see how this site uses Cookies. View our privacy policy here.