DOI:
10.1039/C7FO01253E
(Paper)
Food Funct., 2018,
9, 389-396
Quercetin inhibits renal cyst growth in vitro and via parenteral injection in a polycystic kidney disease mouse model
Received
15th August 2017
, Accepted 23rd November 2017
First published on 28th November 2017
Abstract
Autosomal dominant polycystic kidney disease (ADPKD) is a common monogenic disease characterized by massive enlargement of fluid-filled cysts in the kidney. There is an urgent need to develop effective ADPKD therapies. We used an in vitro Madin–Darby canine kidney (MDCK) cyst model and a murine embryonic kidney cyst model to evaluate whether quercetin inhibits cyst development. We then used a polycystic kidney disease (PKD) mouse model to further determine the in vivo effects of quercetin (100 mg per kg body weight twice per day) on PKD mice via subcutaneous injections. The results show that quercetin significantly and dose-dependently inhibited cyst formation and enlargement in the MDCK cyst and embryonic kidney cyst models. Quercetin also noticeably reduced the cystic index in PKD mice. Furthermore, the effective dose of quercetin did not cause cytotoxicity in MDCK cells. Quercetin treatment decreased the levels of intracellular signalling proteins in PKD mouse kidneys, including phosphorylated protein kinase B (also known as AKT) and phosphorylated extracellular signal-regulated kinase (ERK), which are upregulated and promote cyst development in ADPKD. Quercetin also reversed E-cadherin expression, which is localized in normal proximal tubules in PKD mouse kidneys. Taken together, these results demonstrate that quercetin hinders renal cyst development in vivo and in vitro and represents a novel candidate strategy for the treatment of ADPKD.
Introduction
Autosomal dominant polycystic kidney disease (ADPKD) is one of the most common monogenic diseases in humans carrying mutations in the polycystic kidney disease 1 (PKD1) or PKD2 genes,1 which encode the polycystin-1 (PC1) and PC2 proteins in renal tubular epithelia.2,3 The disease is characterized by massive bilateral development of fluid-filled kidney cysts, which progressively compromise the normal renal architecture and ultimately lead to renal failure.4,5 Patients with ADPKD depend upon lifelong haemodialysis or kidney transplantation at the end stage.6 There is currently no effective clinical therapy to delay the development of renal cysts. Thus, it is necessary to develop novel drugs for the treatment of ADPKD.
The mechanisms underlying cystic development and growth in ADPKD are complicated and not completely understood. Many studies have reported that the abnormal proliferation and fluid secretion associated with ADPKD development are regulated via multiple intracellular signalling pathways during ADPKD development, including the mitogen-activated protein kinase (MAPK)/extracellular signal-regulated kinase (ERK) pathway,7,8 phosphatidylinositol 3-kinase (PI3K)/protein kinase B (also known as AKT) pathway,9 mammalian target of rapamycin (mTOR) pathway,10 and Wnt/β-catenin pathway.11 Recently, several studies have found that numerous chemical and natural compounds inhibit renal cysts and alleviate the progression of ADPKD via these pathways.12–14
Quercetin (3,3′,4′,5,7-pentahydroxyflavone), a kind of plant polyphenol, is derived from a variety of fruits and vegetables and possesses multiple pharmacological properties including anticancer,15,16 anti-inflammatory,17 and antioxidant effects.18 Studies have shown that quercetin markedly inhibits malignant cell growth and proliferation through multiple pathways such as the MAPK/ERK and AKT/mTOR pathways,19,20 which are also activated during the development of ADPKD. Thus, we hypothesized that quercetin may inhibit renal cyst development by modulating these related signalling pathways.
In the current study, we investigated the effects of quercetin on renal cysts using an in vitro Madin–Darby canine kidney (MDCK) cyst model and an in vivo PKD mouse model. Surprisingly, our experimental results show that quercetin significantly inhibits cyst formation and development in both the in vitro and in vivo cyst models, indicating that the compound could be developed as a candidate therapeutic drug for preventing ADPKD.
Materials and methods
Reagents
Quercetin (Sigma, Q4951), epidermal growth factor (EGF, Sigma, E4127), forskolin (Sigma, F6886), 8-bromoadenosine 3′,5′-cyclic monophosphate (Sigma, B5386), and insulin-transferrin-selenium (ITS, Gibco, 51500056) were used in these experiments. Primary antibodies were used as follows: anti-actin (Bioworld, AP0060), anti-ERK (CST, 9102), anti-phospho-ERK (Thr202/Tyr204) (CST, 4370), anti-Akt (CST, 4691), anti-phospho-Akt (Ser473) (CST, 4060), anti-E-cadherin (CST, 3195), and fluorescein-labelled lotus tetragonolobus lectin (LTL, Vector Laboratories, FL-1321). In addition, donkey anti-rabbit IgG H&L (Alexa Fluor 555) (Abcam, ab150074) and goat anti-rabbit (LI-COR, 926-3221) secondary antibodies were used. A cAMP enzyme immunoassay kit (Sigma, CA200) and cell counting kit-8 (CCK-8) (Dojindo, CK04) were used for related research.
MDCK cyst model
NBL-2 MDCK cells (purchased from Shanghai Institutes for Biological Sciences, Chinese Academy of Sciences, GNO23) were incubated at 37 °C and 5% CO2. A mixture of Dulbecco's modified Eagle's medium (DMEM) and F-12 medium (1
:
1) was used with 5% foetal bovine serum, 100 U ml−1 penicillin, and 100 μg ml−1 streptomycin. For cyst formation, 1 × 103 MDCK cells were suspended in a solution containing 2.655 × minimum essential medium (MEM, 400 μl), 3.2 mg ml−1 collagen (PureCol, Advanced BioMatrix), 1 M hydroxyethyl piperazineethanesulfonic acid (HEPES), 5% NaHCO3, 1 N NaOH, 100 U ml−1 penicillin, and 100 μg ml−1 streptomycin. The cells were then seeded onto 24-well plates (400 μl per well) that were pre-warmed at 37 °C for 30 minutes and then incubated at 37 °C for 45 minutes. Quercetin standards were prepared by first diluting the quercetin stock solution (200 mM) into 10 mM, 5 mM, and 2.5 mM solutions using dimethyl sulphoxide (DMSO), followed by preparation of 10 μM, 5 μM, and 2.5 μM solutions using the medium. The medium mixture with or without forskolin (10 μM), EGF (10 ng ml−1), and quercetin was then added to each well and replenished after 4 h; the media was changed every 12 h for 10 days. Cysts were monitored on days 4, 6, 8, and 10 after the cells were seeded via an in situ tracking method. To evaluate growth, cysts with diameters greater than 50 μm were measured on day 6 using Image J software.
Murine embryonic kidney cyst model
Embryonic kidneys were obtained on embryonic day 13.5 (E13.5) and placed on transwells (Corning, 3460).21 The embryonic kidneys were maintained in DMEM/F-12 medium (1
:
1) with 5% foetal bovine serum, 5 mg ml−1 insulin, 0.55 mg ml−1 transferrin, 0.67 ng ml−1 sodium selenite, 0.2 mg ml−1 ethanolamine, 100 U ml−1 penicillin, and 100 μg ml−1 streptomycin at 37 °C and 5% CO2 for 4 days. The medium mixture with or without 8-Br-cAMP (100 μM) and quercetin was then added to each well and replaced every 24 h. The kidneys were photographed daily using an Olympus microscope. Kidneys were measured using Image J software on day 4 to evaluate cyst area and total kidney area.
Animals
Pkd1fl/fl:Ksp-Cre mice were generated by intercrossing Pkd1fl/+:Ksp-Cre mice (State Key Laboratory of Natural and Biomimetic Drugs, School of Basic Medical Sciences, Peking University) with a C57BL/6 genetic background. Neonatal mice (postnatal days 2 or 4) were genotyped using genomic PCR. The Pkd1fl/fl:Ksp-Cre mice can only survive up to 15 days; thus, experiments were performed only up to postnatal day 10 (p10). Mice were injected with quercetin (100 mg kg−1) or vehicle (100% DMSO, 2 μl × body weight) twice per day for 4 or 7 consecutive days. Chemicals were administered via subcutaneous injection in the back of the mice21,22 every 12 h using a microinjector (25 μl, Shanghai Gaoge Industrial and Trading Company, Ltd). The body weight of the animals was from 2.5 to 5.5 g, and the actual injected dose of quercetin was from 0.25 to 0.55 mg per experiment. The quercetin solution (50 mg ml−1) was prepared in DMSO. We then analysed the mice using western blotting, cAMP measurements, and immunofluorescence. Kidneys were collected, weighed, and fixed for histologic examination 5 or 10 days after birth. All procedures in this study were carried out in strict accordance with the recommendations of the Guide for the Care and Use of Laboratory Animals of China Association for Laboratory Animal Science and approved by the Committee on the Ethics of Laboratory Animals of Xuzhou Medical University.
Cytotoxicity assay
A CCK-8 assay was used to assess quercetin cytotoxicity. MDCK cells were seeded in 96-well plates (8000 cells per ml) and then starved in DMEM/F12 (1
:
1) containing 0.4% bovine serum albumin (BSA) for 12 h followed by exposure to quercetin (0, 2.5, 5, 10, or 20 μM) for 24 h at 37 °C and 5% CO2. CCK-8 solution (10 μl) was then added to each well. Cytotoxicity is expressed as an OD450 value.
cAMP measurements
Pkd1fl/fl:Ksp-Cre mice were treated with quercetin from postnatal day 2 (P2) to P5, and kidneys were collected on P5. Tissue samples frozen in liquid nitrogen were then ground to a fine powder under liquid nitrogen in a stainless-steel mortar. After the liquid nitrogen evaporated, we weighed and homogenized the frozen tissue in 10 volumes of 0.1 M HCl. The homogenate was then centrifuged at 600g at room temperature. The diluted samples were used for the cAMP enzyme immunoassay according to cAMP enzyme immunoassay kit (Sigma, CA200).
Immunofluorescence staining
Pkd1fl/fl:Ksp-Cre mice were treated with quercetin (100 mg kg−1) twice per day from P4 to P10. The kidneys were collected after 10 days and fixed in 10% formaldehyde for 48 h at room temperature. Sections (3 μm) were embedded in paraffin, blocked with 5% (w/v) bovine serum albumin for 20 min at 37 °C, and then incubated with E-cadherin (1
:
100) and fluorescein-labelled LTL (1
:
200) primary antibodies overnight at 4 °C before incubating with donkey anti-rabbit IgG H&L (1
:
200) secondary antibodies.
Haematoxylin and eosin (H&E) staining
To assess the cystic area, kidney samples were fixed in 10% formaldehyde and embedded in paraffin. Sections were stained with H&E, and the cystic areas quantified using ImageJ software.
Western blotting
Kidney tissues were homogenized using protein lysis buffer containing 10 mM triethanolamine, 250 mM sucrose (pH 7.6), and protease inhibitor containing 1 mM phenylmethylsulphonyl fluoride (PMSF), 20 mM NaF, and 1 mM Na3VO4. Protein concentrations were determined by the bicinchoninic acid (BCA) protein assay. After adding sodium dodecyl sulphate polyacrylamide gel electrophoresis (SDS-PAGE) buffer, the kidney lysates were denatured in boiling water for 10 minutes, separated by SDS-polyacrylamide gel electrophoresis, and transferred onto nitrocellulose membranes. The membranes were blocked for 1 h with 3% BSA in phosphate-buffered saline (PBS). Primary antibodies were then incubated overnight at 4 °C with rabbit anti-Erk 1
:
1000, p-Erk 1
:
1000, Akt 1
:
1000, p-Akt 1
:
1000, β-Actin 1
:
1000, and E-cadherin 1
:
1000 (Cell Signaling Technology). The membranes were then incubated with goat anti-rabbit secondary antibodies (LI-COR, 926-3221) and detected using an Odyssey infrared imaging system.
Statistical analysis
All results are presented as means ± SE. Statistical analysis was performed using one-way analysis of variance (ANOVA) followed by an unpaired Student's t-test with SPSS 16.0 statistical software. Differences were considered significant when P < 0.05.
Results
Quercetin inhibits cyst formation and growth in an in vitro MDCK model
An MDCK cyst model was used to evaluate the effects of quercetin on cyst formation and growth. MDCK cells were cultured in three-dimensional collagen gels with 10 μM forskolin. The cysts formed by MDCK cells were observed every 2 days from day 4 after cell seeding and progressively expanded over the next 6 days (Fig. 1A, top panel). When the cells were treated with the indicated concentrations of quercetin (2.5, 5, and 10 μM), cyst formation and growth were dramatically inhibited in a dose-dependent manner (Fig. 1B and C).
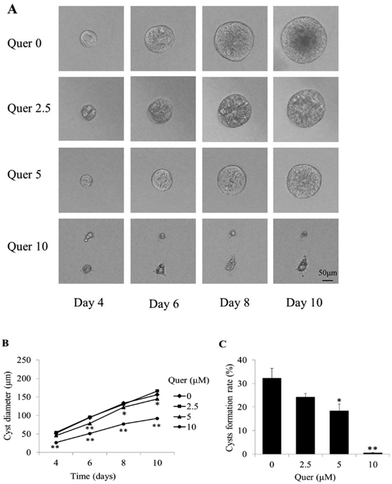 |
| Fig. 1 Quercetin inhibits cyst growth in matrix-grown Madin–Darby canine kidney (MDCK) cells. (A) Light micrographs of MDCK cysts in collagen gels from day 4 to day 10 are shown. Cells were exposed to 10 μM forskolin without quercetin (top row), 2.5 μM quercetin (second row), 5 μM quercetin (third row), and 10 μM quercetin (bottom row). The representative light photographs obtained by an in situ tracking method show the same cyst from day 4 to day 10 after cell seeding. Scale bar = 50 μm. (B) MDCK cell cyst diameters determined from day 4 to day 10 at the indicated concentration of quercetin are shown (mean ± SE, >50 cysts analysed per time point; *P < 0.05 and **P < 0.01 vs. control). (C) Rate of MDCK cyst formation on day 6 of MDCK cell culture with 10 μM forskolin in the absence or presence of quercetin at the indicated concentrations. The cysts are calculated with diameter > 50 μm (mean ± SE, n = 3, *P < 0.05 and **P < 0.01 vs. control). Quer, quercetin. | |
Quercetin impedes cyst development in an embryonic kidney organ culture model
To further examine the effect of quercetin on renal cyst development, we used embryonic kidneys isolated from wild-type mice on E13.5 and cultured for 4 days with or without 100 μM 8-Br-cAMP. Numerous cysts were detected on day 4 in the presence of 8-Br-cAMP. The results showed that quercetin (5 μM and 10 μM) significantly inhibits cyst formation and enlargement (Fig. 2A and B).
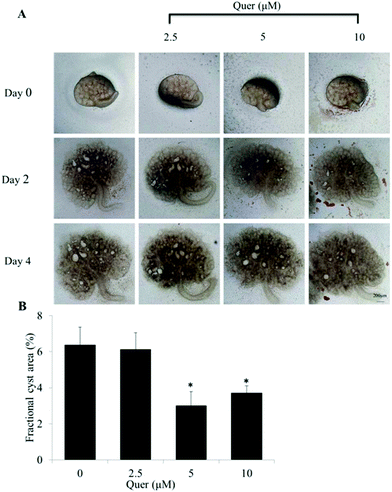 |
| Fig. 2 Quercetin impedes cyst development in an embryonic kidney organ culture model. (A) Embryonic kidneys were cultured with 100 μM 8-Br-cAMP for 4 days and photos were taken with a transmitted light microscope. (B) Quercetin-mediated inhibition of the fraction cyst area at the indicated concentrations is shown (mean ± SE, n = 5–6, *P < 0.05 vs. control). | |
Quercetin inhibits the development of renal cysts in an in vivo PKD mouse model
To test whether quercetin inhibits renal cyst enlargement and development in PKD mice, we generated kidney-specific Pkd1 knockout mice (Pkd1fl/fl:Ksp-Cre) by crossbreeding Pkd1fl/+:Ksp-Cre mice. Quercetin (50 mg ml−1) was prepared in DMSO. Quercetin or vehicle was injected subcutaneously into the back of mice at 100 mg kg−1 twice per day from P4 to P10. Throughout the treatment period, no differences were detected in body weight or activity among PKD mice without any treatment and PKD mouse groups treated with DMSO or quercetin (Fig. 3E), indicating this dose of DMSO or quercetin did not show any effect on the body weight of PKD mice. The ratio of kidney weight to body weight was significantly lower in quercetin-treated PKD mice than that in vehicle-treated PKD mice (Fig. 3A and B). Image analysis of H&E-stained kidney sections showed a noticeably lower cyst index (percentage of cyst area to kidney area) in quercetin-treated PKD mice than that in untreated PKD mice (Fig. 3C and D).
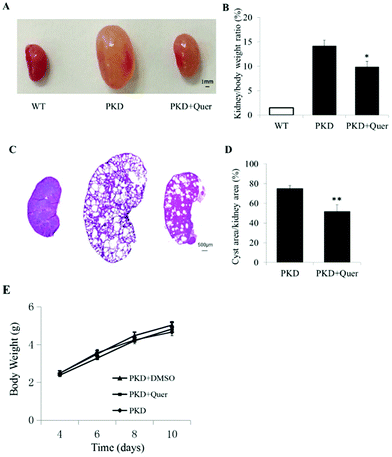 |
| Fig. 3 Quercetin inhibits cyst enlargement in Pkd1fl/fl:Ksp-Cre mice. (A) Representative photographs of the kidney (on postnatal day 10 [P10]) from wild-type mice (left) and Pkd1fl/fl:Ksp-Cre mice treated with dimethyl sulphoxide (DMSO) (middle) or quercetin (right) for 7 days are shown. (B) Kidney weight indexes of wild-type mice and Pkd1fl/fl:Ksp-Cre mice untreated or treated with quercetin 100 mg kg−1 twice per day are shown (mean ± SE, n = 7, *P < 0.05 vs. control). (C) Haematoxylin and eosin (H&E)-stained sections are shown. (D) Cyst indexes of the kidney (%) from DMSO- and quercetin-treated Pkd1fl/fl:Ksp-Cre mice are shown (mean ± SE, n = 7, **P < 0.01 vs. control). (E) Body weights of polycystic kidney disease (PKD) mice untreated or treated with quercetin are shown (mean ± SE, n = 7). WT, wild type; Quer, quercetin. | |
Quercetin does not show significant cytotoxicity in MDCK cells
Quercetin showed inhibitory effects on cyst formation and growth in the in vitro MDCK model. To determine whether cyst inhibition was attributable to quercetin-induced cytotoxicity in MDCK cells, a CCK-8 assay was used to measure MDCK cell proliferation in the presence of quercetin. Interestingly, no cytotoxicity was observed in MDCK cells after treatment with quercetin at concentrations below 20 μM (Fig. 4A).
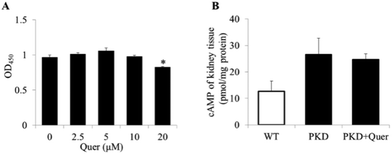 |
| Fig. 4 The effects of quercetin on Madin–Darby canine kidney (MDCK) cells are shown. (A) MDCK cells were incubated in the absence or presence of quercetin at the indicated concentrations (2.5, 5, 10, and 20 μM) for 24 h. Cytotoxicity was assayed using a cell counting kit 8 (CCK-8), and the data are shown as the absorbance at 450 nm (mean ± SE, n = 6, *P < 0.05 vs. control). (B) Cyclic AMP (cAMP) levels were measured in the kidneys of polycystic kidney disease (PKD) mice (mean ± SE, n = 6). Quer, quercetin. | |
Quercetin does not affect cAMP levels in PKD mouse kidneys
It has been reported that cAMP levels are significantly upregulated in ADPKD renal cells, which induces changes in downstream signalling pathways.23 To explore the inhibitory mechanism of quercetin, we measured intracellular cAMP levels (Fig. 4B) in kidneys of PKD mice treated or untreated with quercetin from P2 to P5. We measured intracellular cAMP levels and molecular signalling at the early stage in PKD mice (up to P5), because there are very few normal renal cells at the late stage in PKD mice (on P10). The results showed no obvious differences in the renal cAMP levels between quercetin-treated and untreated PKD mice.
Quercetin regulates the ERK and AKT signalling pathways in the PKD mouse model
To further explore the mechanisms underlying quercetin-mediated inhibition of cyst formation and development, we analysed multiple signalling pathways by means of western blot in kidneys of PKD mice treated or untreated with quercetin (Fig. 5A and B). The levels of p-ERK and p-AKT increased in the kidneys of PKD mice; however, the levels of the activated signalling proteins were significantly reduced by quercetin. These results suggest that quercetin delays the onset and development of renal cysts by regulating the ERK and AKT signalling pathways.
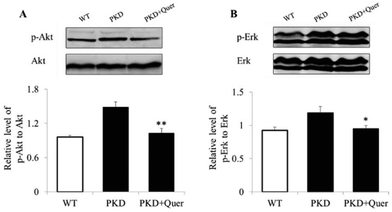 |
| Fig. 5 Quercetin inhibits ERK and AKT signalling pathways in a polycystic kidney disease (PKD) mouse model. Western blots of tissue homogenates (from postnatal day 5 [P5]) of wild-type, untreated, and quercetin-treated (from P2 to P5) PKD kidneys are shown. (A) ERK and AKT expression in wild-type and PKD mice treated with dimethyl sulphoxide (DMSO) and quercetin are shown. (B) The ratios of p-ERK/ERK and p-AKT/AKT are shown (mean ± SE, n = 5, *P < 0.05 and **P < 0.01 vs. control). | |
Quercetin increases E-cadherin expression in PKD mouse kidneys
E-cadherin forms adherens junctions as a stable cell–cell contact via homophilic interaction of its ectodomain and plays a critical role in maintaining the integrity of the planar polarity and cell–cell adhesions.24 The expression of E-cadherin was significantly reduced at the late stage in the PKD mouse kidneys (Fig. 6A). However, quercetin restored the expression of E-cadherin on P10. Fluorescent staining showed that E-cadherin is specifically localized on the proximal tubular cell (Fig. 6B). Following treatment with quercetin, the number of proximal tubules in the PKD mouse kidneys increased, and inhibition of E-cadherin expression was reversed.
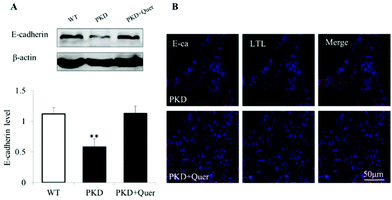 |
| Fig. 6 Quercetin increases E-cadherin expression in the kidneys of polycystic kidney disease (PKD) mice. (A) Western blots of tissue homogenates (P10) of wild-type, untreated, and quercetin-treated (from P4 to P10) PKD kidneys are shown (mean ± SE, n = 5, **P < 0.01 vs. control). (B) Fluorescent staining of E-cadherin and lotus tetragonolobus lectin (LTL), a proximal tubular cell marker, is shown. | |
Discussion
The goal of the present study was to determine whether quercetin exerts any effects on renal cyst formation and development in vivo and in vitro. Intriguingly, quercetin dramatically inhibited cyst formation and enlargement in a MDCK cyst model and a murine embryonic kidney cyst model.
Further, the kidney weight to body weight ratio and cyst indexes were lower in PKD mice treated with quercetin than those in untreated PKD mice.
Quercetin, one of the most prevalent flavonoids in the diet, is widely distributed in various fruits and vegetables, including onions, apples, berries, and broccoli.25 Recent studies have found that quercetin has anticancer effects against diverse tumour types via multiple signalling pathways.26,27 Considering these effects of quercetin, we used a MDCK cyst model to determine whether quercetin inhibits cyst formation and enlargement in vitro. The MDCK cyst model is a classic model imitating renal cysts in vitro, which are established by culturing MDCK type I cells in three-dimensional collagen gels and exposing them to forskolin. MDCK cells grow into large cysts because of abnormal proliferation and fluid secretion, which are dependent upon increased cAMP levels similar to those observed in tubular epithelial cells from ADPKD kidneys.28,29 Intriguingly, our results showed that quercetin significantly inhibited the MDCK cyst growth in a dose-dependent manner. The results from embryonic kidney culture also showed that quercetin impedes cyst formation and enlargement in embryonic kidneys in the presence of 8-Br-cAMP. To further confirm the effects of quercetin on ADPKD development, we treated Pkd1fl/fl:Ksp-Cre mice with quercetin.
Pkd1fl/fl:Ksp-Cre mice are a representative ADPKD mouse model and are used to evaluate therapeutic drugs for PKD. Homozygous mice with a Pkd1 deletion in the kidney develop cysts after birth and deteriorate very quickly, generally die within 20 days after birth. We found that quercetin clearly decreased the kidney weight to body weight ratio in PKD mice. H&E staining of PKD kidneys in mice treated with quercetin showed smaller cysts than those in PKD control mice. The cystic index values, calculated as the ratio of the cystic area to the whole renal area, were significantly decreased by quercetin treatment. These results suggest that quercetin is a candidate agent to ameliorate PKD. To elucidate the mechanisms underlying the effects of quercetin on PKD, we conducted further investigations.
Previous studies have shown that increased cAMP levels promote fluid secretion from ADPKD cyst epithelial cells via cystic fibrosis transmembrane conductance regulator (CFTR) channels and promote cyst proliferation by upregulating the MAPK/ERK signalling pathway.30–32 However, we did not observe an obvious change in cAMP levels in PKD mice after quercetin treatment. Additionally, we examined the effects of quercetin on the MAPK/ERK signalling pathway by measuring the phosphorylation level of ERK, which plays a critical role in the pathway and is required for both cAMP response element-binding protein (CREB) phosphorylation and its transcriptional activity.33 Our results showed that quercetin significantly reduced p-ERK in kidneys of PKD mice.
Another signalling pathway, PI3K/AKT, has also been reported to be upregulated in PKD and drives abnormal proliferation.34 AKT can be phosphorylated by PI3K and leads to the activation of mTOR.35 They play essential roles in protein translation, cell growth, and proliferation, and they are also upregulated in several types of tumours.36,37 To determine whether quercetin shows effects on the AKT pathway, we measured the phosphorylation levels of AKT. The results showed that quercetin downregulates the level of p-AKT in kidneys of PKD mice, which suggests that the compound inhibits cyst development by reversing the upregulation of the p-AKT pathway.
The integrity of adherens junctions and cell polarity is altered in ADPKD.38 E-cadherin, a cell membrane protein, is involved in maintaining cell–cell adhesions39 and the integrity of the planar polarity.40 It has been reported that E-cadherin can be regulated by the ERK41 and AKT signalling pathways.42 Our results showed that quercetin increases E-cadherin expression, which is localized on the proximal tubules in PKD mouse kidneys. The number of proximal tubules detected in PKD kidneys was higher in mice treated with quercetin than that in PKD kidneys from untreated control mice. These results indicate that quercetin may protect proximal tubules and maintain E-cadherin expression by regulating ERK and AKT signalling pathways.
Conclusions
In conclusion, our study demonstrated, for the first time, that quercetin significantly blocks renal cyst enlargement in vitro and in vivo. Quercetin may inhibit cyst development by regulating the ERK and AKT pathways. Quercetin shows great potential to be developed as a candidate drug for treating ADPKD.
Conflicts of interest
There are no conflicts of interest to declare.
Acknowledgements
This work was supported by the National Natural Science Foundation of China (Grant No. 81603179), the China Postdoctoral Science Foundation (Grant No. 2016 M601893) and Innovative Entrepreneurship Training Program for National College Students (Grant 201710313022). We acknowledge the State Key Laboratory of Natural and Biomimetic Drugs from Peking University for Pkd1fl/+;Ksp-Cre mice and technical support.
Notes and references
- J. Ghata and B. D. Cowley Jr., Polycystic Kidney Disease, Compr. Physiol., 2017, 7, 945–975 CrossRef PubMed.
- P. C. Harris and V. E. Torres, Polycystic kidney disease, Annu. Rev. Med., 2009, 60, 321–337 CrossRef CAS PubMed.
- S. J. Leuenroth and C. M. Crews, Targeting cyst initiation in ADPKD, J. Am. Soc. Nephrol., 2009, 20, 1–3 CrossRef PubMed.
- J. Zhou, Polycystins and primary cilia: primers for cell cycle progression, Annu. Rev. Physiol., 2009, 71, 83–113 CrossRef CAS PubMed.
- E. Cornec-Le Gall, M. P. Audrezet, J. M. Chen, M. Hourmant, M. P. Morin, R. Perrichot, C. Charasse, B. Whebe, E. Renaudineau, P. Jousset, M. P. Guillodo, A. Grall-Jezequel, P. Saliou, C. Ferec and Y. Le Meur, Type of PKD1 mutation influences renal outcome in ADPKD, J. Am. Soc. Nephrol., 2013, 24, 1006–1013 CrossRef PubMed.
- S. Khan, A. Giuliani, C. Crepaldi, C. Ronco and M. H. Rosner, Peritoneal Dialysis for Patients with Autosomal Dominant Polycystic Kidney Disease, Peritoneal Dial. Int., 2017, 37, 384–388 CrossRef PubMed.
- T. Xu, N. S. Wang, L. L. Fu, C. Y. Ye, S. Q. Yu and C. L. Mei, Celecoxib inhibits growth of human autosomal dominant polycystic kidney cyst-lining epithelial cells through the VEGF/Raf/MAPK/ERK signaling pathway, Mol. Biol. Rep., 2012, 39, 7743–7753 CrossRef CAS PubMed.
- J. P. Calvet, Strategies to inhibit cyst formation in ADPKD, Clin. J. Am. Soc. Nephrol., 2008, 3, 1205–1211 CrossRef PubMed.
- N. G. Santoso, L. Cebotaru and W. B. Guggino, Polycystin-1, 2, and STIM1 interact with IP(3)R to modulate ER Ca release through the PI3K/Akt pathway, Cell. Physiol. Biochem., 2011, 27, 715–726 CrossRef CAS PubMed.
- L. de Stephanis, A. Bonon, K. Varani, G. Lanza, R. Gafa, P. Pinton, M. Pema, S. Somlo, A. Boletta and G. Aguiari, Double inhibition of cAMP and mTOR signalling may potentiate the reduction of cell growth in ADPKD cells, Clin. Exp. Nephrol., 2017, 21, 203–211 CrossRef CAS PubMed.
- H. Happe, W. N. Leonhard, A. van der Wal, B. van de Water, I. S. Lantinga-van Leeuwen, M. H. Breuning, E. de Heer and D. J. Peters, Toxic tubular injury in kidneys from Pkd1-deletion mice accelerates cystogenesis accompanied by dysregulated planar cell polarity and canonical Wnt signaling pathways, Hum. Mol. Genet., 2009, 18, 2532–2542 CrossRef CAS PubMed.
- E. Y. Park, Y. M. Woo and J. H. Park, Polycystic kidney disease and therapeutic approaches, BMB Rep., 2011, 44, 359–368 CrossRef CAS PubMed.
- Y. Sun, H. Zhou and B. X. Yang, Drug discovery for polycystic kidney disease, Acta Pharmacol. Sin., 2011, 32, 805–816 CrossRef CAS PubMed.
- H. A. Blair and G. M. Keating, Tolvaptan: A Review in Autosomal Dominant Polycystic Kidney Disease, Drugs, 2015, 75, 1797–1806 CrossRef CAS PubMed.
- S. Jagtap, K. Meganathan, V. Wagh, J. Winkler, J. Hescheler and A. Sachinidis, Chemoprotective mechanism of the natural compounds, epigallocatechin-3-O-gallate, quercetin and curcumin against cancer and cardiovascular diseases, Curr. Med. Chem., 2009, 16, 1451–1462 CrossRef CAS PubMed.
- A. Murakami, H. Ashida and J. Terao, Multitargeted cancer prevention by quercetin, Cancer Lett., 2008, 269, 315–325 CrossRef CAS PubMed.
- M. Comalada, D. Camuesco, S. Sierra, I. Ballester, J. Xaus, J. Galvez and A. Zarzuelo, In vivo quercitrin anti-inflammatory effect involves release of quercetin, which inhibits inflammation through down-regulation of the NF-kappaB pathway, Eur. J. Immunol., 2005, 35, 584–592 CrossRef CAS PubMed.
- C. B. Pocernich, M. L. Lange, R. Sultana and D. A. Butterfield, Nutritional approaches to modulate oxidative stress in Alzheimer's disease, Curr. Alzheimer Res., 2011, 8, 452–469 CrossRef CAS PubMed.
- D. N. Syed, V. M. Adhami, M. I. Khan and H. Mukhtar, Inhibition of Akt/mTOR signaling by the dietary flavonoid fisetin, Anticancer Agents Med. Chem., 2013, 13, 995–1001 CrossRef CAS PubMed.
- V. M. Adhami, D. N. Syed, N. Khan and H. Mukhtar, Dietary flavonoid fisetin: a novel dual inhibitor of PI3K/Akt and mTOR for prostate cancer management, Biochem. Pharmacol., 2012, 84, 1277–1281 CrossRef CAS PubMed.
- L. Tradtrantip, N. D. Sonawane, W. Namkung and A. S. Verkman, Nanomolar potency pyrimido-pyrrolo-quinoxalinedione CFTR inhibitor reduces cyst size in a polycystic kidney disease model, J. Med. Chem., 2009, 52, 6447–6455 CrossRef CAS PubMed.
- H. Zhou, J. Gao, L. Zhou, X. Li, W. Li, X. Li, Y. Xia and B. Yang, Ginkgolide B inhibits renal cyst development in in vitro and in vivo cyst models, Am. J. Physiol. Renal. Physiol., 2012, 302, F1234–F1242 CrossRef CAS PubMed.
- P. C. Harris and V. E. Torres, Genetic mechanisms and signaling pathways in autosomal dominant polycystic kidney disease, J. Clin. Invest., 2014, 124, 2315–2324 CrossRef CAS PubMed.
- C. Collins, A. K. Denisin, B. L. Pruitt and W. J. Nelson, Changes in E-cadherin rigidity sensing regulate cell adhesion, Proc. Natl. Acad. Sci. U. S. A., 2017, 114, E5835–E5844 CrossRef CAS PubMed.
- D. Kashyap, S. Mittal, K. Sak, P. Singhal and H. S. Tuli, Molecular mechanisms of action of quercetin in cancer: recent advances, Tumour Biol., 2016, 37, 12927–12939 CrossRef CAS PubMed.
- K. W. Ren, Y. H. Li, G. Wu, J. Z. Ren, H. B. Lu, Z. M. Li and X. W. Han, Quercetin nanoparticles display antitumor activity via proliferation inhibition and apoptosis induction in liver cancer cells, Int. J. Oncol., 2017, 50, 1299–1311 CrossRef PubMed.
- C. L. Luo, Y. Q. Liu, P. Wang, C. H. Song, K. J. Wang, L. P. Dai, J. Y. Zhang and H. Ye, The effect of quercetin nanoparticle on cervical cancer progression by inducing apoptosis, autophagy and anti-proliferation via JAK2 suppression, Biomed. Pharmacother., 2016, 82, 595–605 CrossRef CAS PubMed.
- V. E. Torres and P. C. Harris, Strategies targeting cAMP signaling in the treatment of polycystic kidney disease, J. Am. Soc. Nephrol., 2014, 25, 18–32 CrossRef CAS PubMed.
- T. Yamaguchi, G. A. Reif, J. P. Calvet and D. P. Wallace, Sorafenib inhibits cAMP-dependent ERK activation, cell proliferation, and in vitro cyst growth of human ADPKD cyst epithelial cells, Am. J. Physiol. Renal. Physiol., 2010, 299, F944–F951 CrossRef CAS PubMed.
- C. Yuajit, C. Muanprasat, A. R. Gallagher, S. V. Fedeles, S. Kittayaruksakul, S. Homvisasevongsa, S. Somlo and V. Chatsudthipong, Steviol retards renal cyst growth through reduction of CFTR expression and inhibition of epithelial cell proliferation in a mouse model of polycystic kidney disease, Biochem. Pharmacol., 2014, 88, 412–421 CrossRef CAS PubMed.
- B. Yang, N. D. Sonawane, D. Zhao, S. Somlo and A. S. Verkman, Small-molecule CFTR inhibitors slow cyst growth in polycystic kidney disease, J. Am. Soc. Nephrol., 2008, 19, 1300–1310 CrossRef CAS PubMed.
- T. Yamaguchi, S. Nagao, D. P. Wallace, F. A. Belibi, B. D. Cowley, J. C. Pelling and J. J. Grantham, Cyclic AMP activates B-Raf and ERK in cyst epithelial cells from autosomal-dominant polycystic kidneys, Kidney Int., 2003, 63, 1983–1994 CrossRef CAS PubMed.
- E. R. Simo-Cheyou, V. Youreva and A. K. Srivastava, cAMP attenuates angiotensin-II-induced Egr-1 expression via PKA-dependent signaling pathway in vascular smooth muscle cells, Can. J. Physiol. Pharmacol., 2017, 95, 928–937 CrossRef CAS PubMed.
- D. C. Fischer, U. Jacoby, L. Pape, C. J. Ward, E. Kuwertz-Broeking, C. Renken, H. Nizze, U. Querfeld, B. Rudolph, D. E. Mueller-Wiefel, C. Bergmann and D. Haffner, Activation of the AKT/mTOR pathway in autosomal recessive polycystic kidney disease (ARPKD), Nephrol., Dial., Transplant., 2009, 24, 1819–1827 CrossRef CAS PubMed.
- M. Pema, L. Drusian, M. Chiaravalli, M. Castelli, Q. Yao, S. Ricciardi, S. Somlo, F. Qian, S. Biffo and A. Boletta, mTORC1-mediated inhibition of polycystin-1 expression drives renal cyst formation in tuberous sclerosis complex, Nat. Commun., 2016, 7, 10786 CrossRef CAS PubMed.
- J. T. Zhong, J. Yu, H. J. Wang, Y. Shi, T. S. Zhao, B. X. He, B. Qiao and Z. W. Feng, Effects of endoplasmic reticulum stress on the autophagy, apoptosis, and chemotherapy resistance of human breast cancer cells by regulating the PI3K/AKT/mTOR signaling pathway, Tumour Biol., 2017, 39, 1010428317697562 Search PubMed.
- H. Xu, Y. Feng, Z. Jia, J. Yang, X. Lu, J. Li, M. Xia, C. Wu, Y. Zhang and J. Chen, AXIN1 protects against testicular germ cell tumors via the PI3K/AKT/mTOR signaling pathway, Oncol. Lett., 2017, 14, 981–986 Search PubMed.
- J. X. Xu, T. S. Lu, S. Li, Y. Wu, L. Ding, B. M. Denker, J. V. Bonventre and T. Kong, Polycystin-1 and Galpha12 regulate the cleavage of E-cadherin in kidney epithelial cells, Physiol. Genomics, 2015, 47, 24–32 CrossRef PubMed.
- L. Jia, F. Liu, S. H. Hansen, M. B. Ter Beest and M. M. Zegers, Distinct roles of cadherin-6 and E-cadherin in tubulogenesis and lumen formation, Mol. Biol. Cell, 2011, 22, 2031–2041 CrossRef CAS PubMed.
- T. Nagaoka, A. Inutsuka, K. Begum, K. Bin hafiz and M. Kishi, Vangl2 regulates E-cadherin in epithelial cells, Sci. Rep., 2014, 4, 6940 CrossRef CAS PubMed.
- D. Yao, C. Dai and S. Peng, Mechanism of the mesenchymal-epithelial transition and its relationship with metastatic tumor formation, Mol. Cancer Res., 2011, 9, 1608–1620 CrossRef CAS PubMed.
- W. H. Lee, L. Y. Choong, T. H. Jin, N. N. Mon, S. Chong, C. S. Liew, T. Putti, S. Y. Lu, C. Harteneck and Y. P. Lim, TRPV4 plays a role in breast cancer cell migration via Ca2+-dependent activation of AKT and downregulation of E-cadherin cell cortex protein, Oncogenesis, 2017, 6, e338 CrossRef CAS PubMed.
Footnote |
† These authors contributed equally to this work. |
|
This journal is © The Royal Society of Chemistry 2018 |
Click here to see how this site uses Cookies. View our privacy policy here.