DOI:
10.1039/C7FO01332A
(Paper)
Food Funct., 2018,
9, 534-540
Amaranth addition to enzymatically modified wheat flour improves dough functionality, bread immunoreactivity and quality
Received
29th August 2017
, Accepted 30th November 2017
First published on 5th December 2017
Abstract
Consumers with gluten-related disorders require gluten-free (GF) foods to avoid an immune response. Alternative to the use of non-gluten containing grains to prepare GF bread, the gluten reactivity has been greatly reduced using a proline specific cleavage enzyme, however, the gluten functionality was lost. The aim of this study was to evaluate the effect of adding an amaranth flour blend (AFB) to enzymatically modified wheat-flour proteins on dough functionality and to evaluate the immunoreactivity and acceptability of the prepared bread. First, wheat flour (20% w/v, substrate) was hydrolyzed using 8.4 U mg−1 protein Aspergillus niger prolyl-endopeptidase (AnPEP) for 8 h at 40 °C under constant agitation. Four types of breads were prepared with the same formulation except for the type of flour (14% w.b.): wheat flour (WF), WF-AFB unmodified not incubated, WF-AFB unmodified incubated and WF-AFB modified. The protein composition and free thiols were analyzed before and after amaranth addition, and the flour and bread proteins were run using SDS-PAGE and immune-detected in blots with IgA from celiac disease patients. The immunoreactive gluten content, specific volume and bread acceptability were evaluated. The polymeric proteins and free thiol groups of WF decreased after AnPEP treatment. The electrophoretic patterns of the modified flour and bread proteins were different and the IgA-immunodetection in blots was highly reduced, particularly for the higher molecular weight subunits. The addition of AFB to the modified wheat flour prepared using AnPEP improved the dough functionality by increasing the thiol groups and allowed the preparation of a sensorially acceptable bread with only 60 mg kg−1 immunoreactive gluten.
Introduction
Gluten-related disorders, which are more frequent nowadays than 2 or 3 decades ago, describe a set of conditions related to the ingestion of gluten or wheat-containing foods. Among these diseases are celiac disease, wheat allergy and non-celiac gluten sensitivity or wheat intolerance syndrome, whose therapy is to maintain a gluten-free (GF) diet.1 Therefore, the development and improvement of GF products have increased in the last few years.
GF foodstuffs often have a poor nutritional quality and a deficient content of fiber, vitamins, folates and iron due to the lack of nutrient fortification when compared with the gluten-containing products.2,3 GF breads generally present low volume, poor flavor, dry crumb and a short shelf-life when compared to regular bread. GF bread consumers usually complain more about the taste and odour rather than appearance.4 Therefore, the development of GF bread with improved sensory attributes is required.
Among the strategies used to develop GF products is the enzymatic gluten hydrolysis with fungal and bacterial peptidases, which results in a high reduction of the immunoreactivity but poor functionality.5 Another approach is enzymatic transpeptidation used to modify the epitopes in isolated gluten or whole wheat flour.6,7 A more specific way to hydrolyse immunogenic peptides, which gastrointestinal enzymes are unable to split, is the use of prolyl-endopeptidases, which effectively cleave proline-rich gluten sequences.8,9
The modification of wheat dough using an Aspergillus niger prolyl-endopeptidase supplemented with an amaranth blend has been previously reported.8 Using this modification, loaves of bread with 1680 mg kg−1 reactive gluten and an acceptable appearance were obtained. It is evident that more research is needed to fulfill the demand of GF breads with acceptable sensory qualities. Therefore, the objective of this study was to determine the effect of adding an amaranth flour blend (AFB) on the dough functionality, improving the enzymatic modification conditions of wheat-flour proteins and to evaluate the immunoreactivity and quality of the prepared breads.
Materials and methods
Wheat flour was obtained from the Villa Juarez variety provided by INIFAP (Ciudad Obregon, Mexico). Raw and popped amaranth grains were obtained locally (Invernadero de Tulyehualco, Tulyehualco, Ciudad de Mexico, CDMX, and Puebla, Mexico, respectively) and milled using a coffee/spice grinder (Model Krups GX 410011 V, Groupe SEB Mexico, S. A. de C. V., Ciudad de Mexico, CDMX). The Aspergillus niger prolyl-endopeptidase (AnPEP) used to modify the wheat gluten was donated by DSM Food Specialties (B. V., Delft, The Netherlands). Shortening, dry yeast, sugar and salt were obtained from a local market. All chemicals used were reagent grade.
Wheat flour proteins modification by AnPEP
Proteins-modification of wheat flour was carried out using a commercial enzyme (AnPEP). First, the enzyme activity was measured using a spectrophotometric method10 at 40 °C at pH 6.0 for 30 min, and the produced p-nitroaniline monitored at 410 nm (molar extinction coefficient of 8800 M−1 cm−1). One activity unit (1 U) was the enzyme activity that releases 1 mol of p-nitroaniline in 1 min at 40 °C and pH 6.0 using Z-Gly-Pro-pNA (Sigma-Aldrich, Germany) as the substrate. To determine the specific activity, the protein content of the AnPEP preparation was quantified.11
The reaction conditions for wheat flour modification using AnPEP were previously selected based on the decrease in gluten immunoreactivity with modifications.8 Wheat flour (20%, w/v, substrate) was suspended in distilled water and mixed with the AnPEP preparation (8.4 U mg−1 protein), and incubated under continuous agitation (150 rpm) for 8 h at 40 °C. After incubation, each sample was centrifuged at 1250g for 15 min. The pellet was collected and freeze-dried; then, the gluten immunoreactivity was determined using ELISA-R5 RIDASCREEN® Gliadin kit (RBiopharm, Darmstadt, Germany) according to the labeled instructions.
Protein composition by SE-HPLC
Proteins were extracted from the freeze-dried modified flour at 40 °C as described by Batey et al.12 The protein extracts were analyzed using an Agilent 1100 Series (Agilent Technologies, Santa Clara, CA) by automatic injection and were fractionated into three main peaks using a Biosep-SEC-S4000 column (Phenomenex, Torrence, CA). The eluent was 50% acetonitrile with 0.05% (v/v) trifluoroacetic acid at a flow rate of 0.5 mL min−1. The solutes were detected at 214 nm. The SE-HPLC profile was divided in 3 peaks corresponding to polymeric protein (glutenin), gliadins and albumins/globulins. The percentages of total polymeric protein (TPP), total unextractable polymeric protein (TUP) and unextractable polymeric protein in the flour (UPF) were calculated.12
Quantification of the sulfhydryl (–SH) groups
The quantification of the free-SH groups in the modified wheat flour and the amaranth flour blend were carried out using Ellman's reagent 5–5′-dithiobis-2-nitrobenzoic acid (DTNB).13 Ellman's reagent containing 12 mg of DTNB in Tris-HCl buffer pH 8.5 was mixed with the flour samples (60 mg). Immediately the suspension was mixed with a vortex for 10 min and centrifuged at 20
800g for 5 min at 20 °C. The absorbance at 412 nm was recorded (Agilent Cary 60 UV-Vis, Agilent Technologies Inc., Santa Clara, CA). The values are reported as the mean of three measurements.
Breadmaking
The ingredients used for breadmaking are listed in Table 1. The four breads were prepared on the basis of 30 g of flour (14% wet basis), except for breads c and d, which were prepared using the wet pellet obtained after centrifugation of the treated wheat flour (20% w/v in water) after incubation without (bread c) and with (bread d) AnPEP for 8 h at 40 °C under continuous agitation (150 rpm). The pellets were weighed with a composition of 60% (w/w) wheat flour and the other 40% was supplemented with the amaranth flour blend (20
:
80; raw
:
popped amaranth flours) according to Heredia-Sandoval et al.8 The ingredients were mixed in a 35 g mixograph (National Manufacturing Co., Lincoln, NE) for 2.5 min. The dough pieces were molded and fermented for 52 min at 85% relative humidity and 30 °C. Finally, the doughs were baked for 17 min at 215 °C.
Table 1 Bread formulations of wheat flour unmodified or modified using AnPEP supplemented with the amaranth blend
Ingredients |
Breads |
|
a |
b |
c |
d |
Wheat flour (g) |
30.00 |
18.00 |
— |
— |
Unmodified wheat flour pellet (g) |
— |
— |
32.70 |
— |
Modified wheat flour pellet (g) |
— |
— |
— |
33.70 |
Amaranth flour blend (g) |
— |
12.00 |
12.00 |
12.00 |
Sugar (g) |
1.00 |
1.00 |
1.00 |
1.00 |
Salt (g) |
0.26 |
0.26 |
0.26 |
0.26 |
Yeast (g) |
0.60 |
0.60 |
0.60 |
0.60 |
Shortening (mL) |
1.00 |
1.00 |
1.00 |
1.00 |
Water (mL) |
18.20 |
16.40 |
0.70 |
0.70 |
Specific volume
The weight and loaf volume after baking were recorded. The specific volume, expressed in cm3 g−1, was calculated by dividing loaf volume and weight (method 10-10B).14
Sensory evaluation and electronic nose analysis
An untrained panel of students and employees (n = 81; 37 males and 44 females) aged between 16 and 62 years volunteered to perform the sensory evaluation of the modified bread. The breads were baked the day before the sensory tests and served at room temperature under normal illumination. A slice of the modified bread (5 mm thick) was placed into a small sealed polyethylene bag and provided to each participant. The panel evaluated the odour and taste attributes, and overall acceptability using a 15 cm hedonic scale, where 15 = like extremely, 7.5 = neither like nor dislike and 0 = extremely dislike. Furthermore, the panelists gave their opinion about the modified bread and expressed their willingness to buy it or not. All participants provided written informed consent for their inclusion in the study. The protocol was approved by the Bioethics Committee of Centro de Investigación en Alimentación y Desarrollo, A.C. (CE/011/2016).
Simultaneously to the sensory analysis, the bread samples prepared with the wheat flour and the modified flour plus the AFB were analyzed with an electronic nose (e-nose), α-Gemini (HS100, Alpha M.O.S., Toulouse, France), which employs 6 metal oxide semiconductor (MOS) sensors.15 Samples (1 g) of each bread were placed into headspace vials (10 mL). Each sample was incubated at 80 °C for 5 min; then, 1 mL of air was withdrawn from the headspace and injected into the sensor chamber and flushed over the sensors at a flow rate of 150 mL min−1. Data were recorded for 1.5 min using the instrument. The sensors were then flushed with dry air for 2 min. After a delay or 2 min, the next sample was analyzed. A discriminant factorial analysis was performed using the AlphaSOFT (version 12.3.3) software to evaluate the sensors response spectra of the volatiles of the bread made with wheat flour (WF) and the modified bread made with AnPEP supplemented with the amaranth blend. Each sample was analyzed in triplicate.
Humoral immune response and immunoreactivity of gluten
The humoral immune response was evaluated using a sera pool from 8 celiac disease patients and the immunoreactive gluten in the pellets as well as in the loaves of bread were quantified using ELISA-R5 RIDASCREEN® as previously described.
Sodium dodecyl sulfate polyacrylamide gel electrophoresis (SDS-PAGE) was performed16 under denaturing and reducing conditions on a 12.5% (w/v) acrylamide separating gel (Bio-Rad, Richmond, CA). The extracts from the wheat flour and breads were obtained by adding 100 mg of sample to 300 μL of the 5× extraction buffer (0.3 M Tris-HCl (pH 6.8), 0.35 M SDS, 50% (v/v) glycerol, 0.05% (w/v) bromophenol blue and 0.05% (w/v) b-mercaptoethanol in Milli-Q water) and 1200 μL of Milli-Q water into an eppendorf tube (2 mL) and vortexing for 20 min. Subsequently, each sample was heated at 95 °C for 10 min and centrifuged at 12
000g for 10 min. The electrophoretic patterns of the proteins were obtained by running the samples at 200 V for 45 min with molecular mass standards (SDS-PAGE Standards, Broad Range; Bio-Rad, Segrate, Italy). Gels were stained with Coomassie blue or electro-transferred to nitrocellulose membranes by semi-dry blotting. The membranes were blocked (50 mM Tris, 150 mM NaN3, pH 7.2, 2% Tween 20) for 2 min
Immunodetection on nitrocellulose membranes was carried out according to Calderón de la Barca et al.17 The membranes were incubated overnight at 4 °C, diluted with 1
:
50 (v/v) in TBST (50 mM Tris, 150 mM NaCl, 0.05% Tween 20 and 5 mM NaN3; incubation and washing buffer) sera pool from 8 celiac disease patients. After three washes, additional 2 h of incubation with HRP-conjugated rabbit anti-human IgA (DAKO, Glostrup, DK), 1
:
2000 (v/v) in TBST was carried out. After three washes, the HRP activity was developed using 1 DAB (3,3′-diaminobenzidine tetrahydrochloride; Sigma, St Louis, MO) tablet in 15 mL of 12 mM Tris, pH 7.5 and 12 μL of H2O2. Color development was stopped by water washing.
Statistical analysis
Analysis of variance (ANOVA) was performed with the significance level set to p < 0.05 (NCSS, 2000).18 Tukey's procedure was used to test for any differences among the mean values.
Results and discussion
Wheat flour protein modification using AnPEP
The immunoreactive gluten content in wheat flour (freeze-dried pellet) after incubation at 40 °C without AnPEP (negative control) was similar to the previous sample8 after incubation at 35 °C. However, a higher reduction of immunoreactive gluten compared to the untreated flour was obtained after modification at 40 °C instead of 35 °C with a significant (p < 0.05) decrease of 98% (273
000 vs. 4966 mg kg−1). Although the optimum temperature of AnPEP activity is 50 °C,19 we decided to work at 40 °C to partially degrade gluten and preserve the properties for breadmaking to some extent.
Protein composition using SE-HPLC
The effect of AnPEP modification in the protein composition was analyzed using SE-HPLC. The percentages of TPP, TUP and UPF in the three flours are shown in Table 2. No differences (p > 0.05) in these polymeric fractions of wheat flour were observed in the negative control (incubated wheat flour without AnPEP). However, these fractions were significantly reduced (p < 0.05) in the modified wheat flour. In comparison with the wheat flour, there were reductions of about 57.4, 57.2 and 81.6% for TPP, TUP and UPF, respectively. For breadmaking, dough needs a balance between strength and extensibility to obtain an optimum performance and these parameters are determined by the protein composition.20 Islas-Rubio et al.21 have reported a positive correlation between UPF and dough strength. Therefore, a decrease in the gluten strength due to protein hydrolysis by AnPEP was expected.
Table 2 Protein composition of the wheat flours incubated with AnPEP (8.4 U mg−1 protein) for 8 h at 40 °Ca
Sample |
Polymeric proteins (%) |
TPP |
TUP |
UPF |
TPP, total polymeric protein; TUP, total unextractable polymeric protein; UPF, unextractable polymeric protein in the flour. The analysis was performed using freeze-dried samples. The mean value of duplicate determinations. The different letters in the same column indicate the statistical significance (p < 0.05). |
Wheat flour (WF) |
50.6a |
5.8a |
2.9a |
WF unmodified, incubated |
51.1a |
5.6a |
2.8a |
WF modified |
21.6b |
2.5b |
0.5b |
Quantification of the sulfhydryl (–SH) groups
Table 3 shows the sulfhydryl group content in enzymatically modified wheat flour and amaranth flours. The free –SH groups in the modified wheat flour decreased using the AnPEP treatment. However, it is expected that the high –SH content of the raw and popped amaranth flours (14.3 and 12.6 –SH μmol per g of protein), which were both used to prepare the AFB (in 20
:
80 ratio), significantly (p < 0.05) contributed to the increase in the thiol groups in the supplemented AnPEP-treated wheat dough. In addition to the protein composition (TPP, UPP, UPF), the free sulfhydryl content of wheat flour plays an important role in determining the rheological properties of the dough and bread quality. Enzymatic modification produces changes in the gluten proteins, in the intra- and intermolecular protein–protein interactions22,23 and the thiol groups.24,25
Table 3 Sulfhydryl (–SH) group content in enzymatically modified wheat flour prepared with AnPEP (8.4 U mg−1 protein) at 40 °C and amaranth flours
Samples |
–SH (μmol g−1 of protein) |
AnPEP, Aspergillus niger prolyl-endopeptidase; amaranth flour blend, (RA : PA, 20 : 80 w/w); ND, not detected. Mean ± Standard deviation. Means with different letters in the same column are significantly different (p < 0.05). |
Wheat flour (WF) |
1.4 ± 0.1d |
WF incubated (no AnPEP) |
0.7 ± 0.0d |
WF incubated (AnPEP) |
ND |
Popped amaranth (PA) |
12.6 ± 0.8b |
Raw amaranth (RA) |
14.3 ± 1.4ab |
Amaranth flour blend |
15.9 ± 0.4a |
WF (AnPEP) + amaranth flour blend |
10.7 ± 0.1c |
Although the gluten hydrolysis by AnPEP weakened the gluten network, as shown by the decrease in TPP, TUP and UPF, to some extent the AFB addition contributed to the viscoelasticity of the protein network. Therefore, it was possible to obtain a bread with an acceptable specific volume (2.5 cm3 g−1, Table 4), in the range of GF bread but without the use of any additives.
Table 4 Specific volume and immunoreactive gluten content of unmodified (UM) or modified (M) breads prepared with AnPEP for 8 h at 40 °C and supplemented with 40% of the amaranth flour blenda
Bread samples |
Specific volume (cm3 g−1) |
Immunoreactive gluten (mg kg−1) |
WF, wheat flour, AnPEP, Aspergillus niger prolyl-endopeptidase. Mean value of duplicate determinations. The different letters in the same column indicate the statistical significance (p < 0.05). |
a: WF (no amaranth) |
3.9a |
156 200a |
b: UM, no incubated |
3.4ab |
53 600b |
c: UM, incubated at 40 °C |
2.8bc |
58 141b |
d: Modified at 40 °C |
2.5c |
60c |
The –SH content in the amaranth flours were significantly (p < 0.05) higher when compared with wheat flour. The free sulfhydryl groups in raw amaranth flour were statistically similar (p > 0.05) to those of the popped amaranth. The addition of the amaranth flour blend to the modified wheat flour increases (p < 0.05) the –SH content in the mixture. The well-balanced amino acid composition in the amaranth proteins, particularly the high levels of sulfur-containing amino-acids, make it a good option to improve the protein functionality with its the capacity to form intermolecular disulfide bridges during food processing.26
Bread making, sensory evaluation and e-nose analysis
Fig. 1 shows the images of transversal slices and complete crusts of the loaves of the as-prepared bread. In general, the bread loaves present a similar homogeneity of the crumbs, however, the modified bread using AnPEP (Fig. 1d) shows a dry and not very homogeneous crust when compared with the other breads (Fig. 1a–c). This could be due to the reduction of the water holding capacity27 in the modified flour due to the changes in the protein composition and in the proportion of protein to starch, which can affect the protein–protein,28 protein–starch,29 protein–water and starch–water interactions.30 As a consequence, the dough rheology can be affected. A reduction in the water holding capacity negatively affects bread quality, producing breads with a dry crust and low weight.
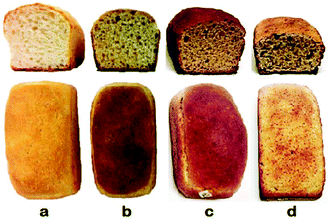 |
| Fig. 1 Breads prepared with wheat flour (WF) supplemented with the amaranth flour blend unmodified (UM) or modified by AnPEP (8.4 U mg−1 protein) for 8 h of incubation at 40 °C and 52 min of fermentation. a, WF (no amaranth); b, UM, no incubated; c, UM incubated at 40 °C; d, modified at 40 °C. | |
The specific volume of the bread loaves are shown in Table 4. The specific volumes ranged from 2.5 to 3.9 cm3 g−1, similar to other gluten-free breads (1.2–3.5 cm3 g−1) prepared without food additives.31 The specific volume of the wheat flour bread (Table 4, bread a) was higher (p < 0.05) when compared with the breads made with incubated wheat flour (unmodified or modified) and supplemented with the amaranth flour blend (Table 4, breads c and d).
The mean odor score was higher than that of taste (11.1 vs. 9.8). The texture, odor and taste are the greatest challenges in the production of gluten-free baked goods because these attributes determine their acceptability.32 Nowadays, the quality of most gluten free products available on market tends to be poor when compared with products elaborated with gluten-containing ingredients.33 The modified bread was rated as acceptable (an overall acceptability mean score of 10.2). It appears that the odor attribute has a higher contribution to the overall acceptability than taste. Most of the panelists did not detect any strange odor or taste in the modified bread even though the modified bread contained 40% of the amaranth flour blend (see the formulation of bread d, in Table 1). Recently, a gluten-free bread containing chia (5, 10, or 14%) and rice flours was developed34 and received overall acceptability scores between 8.1 and 8.6 based on a 10 cm hedonic scale. Similar to our modified bread, the taste score of this bread was lower than that of the odor. It is noteworthy to mention the lower levels of substitution observed with the chia flour when compared to the 40% amaranth flour blend used in the modified bread. In addition to the sensory qualities, the health benefits of food products are an important factor to increase their acceptance.35–37 In regard to the panelists’ willingness to buy the modified bread, 75% (61 out of 81 panelists) stated they would buy the product.
Fig. 2 shows the sensors response spectra of the volatiles of the wheat flour bread and the modified bread. The types of compounds detected in both breads were similar, but their concentrations were lower for the modified bread. The response (intensity) of the P30-1 and P30-2 sensors was higher than that found for the other sensors. These sensors primarily detect alcohols and other organic compounds.15 Acids, alcohols, aldehydes, esters, ketones, pyrroles and pyrazines are the main volatile compounds present in wheat bread, to which its characteristic odor is attributed.38 According to Pico et al.,39 similar volatile compounds profiles (ethanol, hexanal, 2-methylbutanol, 3-methyl-1-butanol, 1-pentanol, 2-heptenal, hexanoic acid and 2,4-decadienal) were observed in the wheat and amaranth bread crumbs, but varied in concentration. Thus, the similar spectra volatiles between both breads can explain the odor score and acceptability given by the panelists for the modified bread sensory evaluation. To relate the odor score with the volatile compounds, it is necessary to identify the individual compounds using more precise methods such as gas chromatography mass spectrometry (GC-MS) or solid-phase microextraction with GC.
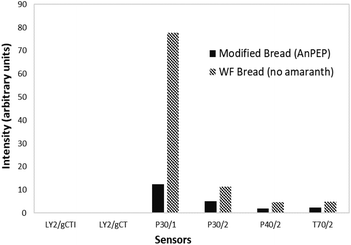 |
| Fig. 2 Sensors response spectra of the volatiles of bread made using wheat flour (WF) and modified bread using AnPEP (8.4 U mg−1 protein) for 8 h of incubation at 40 °C supplemented with the amaranth flour blend. | |
Humoral immune response and immunoreactive gluten
In regards to the immunoreactive gluten content of the modified bread (Table 4), a significant decrease (p < 0.05) when compared to the wheat flour breads was shown (156
200 vs. 60 mg kg−1) due to the effect of the AnPEP modification and the addition of the amaranth flour blend. Furthermore, the AnPEP and other proteases naturally present in the wheat and amaranth flours could be active during the breadmaking process and further contribute to the decrease in the immunoreactivity. Although a significant decrease was found, the gluten content was not reduced below the threshold of 20 mg kg−1 for gluten-free foods,40 but can be considered as a “gluten reduced” or “very low gluten content” product. Mazzarella et al.41 reported that no symptoms were detected in celiac disease patients after 3 months of consumption of a gluten modified bread (50 g day−1) with a gluten content of 1100 mg kg−1.
The electrophoretic patterns of the unmodified (UM) or modified (M) proteins from wheat flour and bread are shown in Fig. 3a. The typical patterns of wheat proteins were observed (lanes 2 and 5), with molecular weights between 45 and 136 kDa.42,43 The high molecular weight glutenins (95 to 136 kDa) almost disappeared in the modified proteins from the wheat flour and breads (lanes 4 and 8). These results are in agreement to those obtained using SE-HPLC for wheat flour protein after AnPEP modification. A significant reduction in the polymeric proteins was observed. Moreover, proteins between 45 and 95 kDa persist in the modified wheat flour (lane 4) when compared to the unmodified flours (lanes 2 and 3) and these proteins correspond to low molecular weight glutenin subunits and gliadins.43
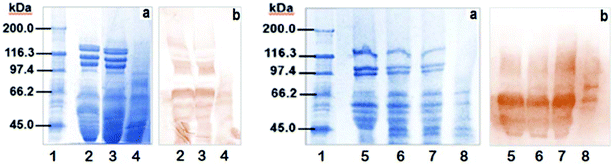 |
| Fig. 3 Electrophoretic pattern (a) and immunoglobulin A immunodetection (b) of the unmodified (UM) or modified (M) wheat flour (WF) and bread proteins incubated with AnPEP at 40 °C. (Lanes 1: molecular-weight stds; 2: WF, no incubation; 3: WF-UM, incubated: 4: WF-M; 5: WF bread; 6: WF supplemented bread; 7: UM supplemented bread, incubated: 8: M supplemented bread). | |
The electrophoretic patterns of the gluten protein from the breads (Fig. 3a) are quite similar to those of the flours with a visible decrease in the proteins extracted from the modified bread (lane 8). Moreover, two additional bands under 45 kDa in the amaranth supplemented breads (lanes 6, 7 and 8) when compared to the wheat flour bread (lane 5) correspond to the amaranth proteins, which are primarily 11S globulins with acidic (35–38 kDa) and basic polypeptide (22–25 kDa) subunits linked by disulfide bonds.44 Such globulins possess a high content of sulfur amino acids and thus have the ability to form S–S and S–H bonds, favoring dough functionality.45
Immunodetection with sera pool of the unmodified or modified proteins from the wheat flours is shown in Fig. 3, part b. The bands on the blot are more intense for the unmodified proteins (lanes 2 and 3) than for the modified proteins (lane 4), which was in agreement with the results of the immunoreactive gluten content using ELISA-R5. This could be due to gluten degradation after the AnPEP treatment. With respect to the gluten proteins in the breads (Fig. 3b), in lane 8 of the modified bread, the IgA from celiac patients detected just two subunits around 66 and 50 kDa, corresponding to γ and ω gliadins, which are the most immunogenic proteins in celiac disease.43,46 Treatment of wheat proteins with AnPEP decreases the reactivity of IgA from celiac patients’ sera. Nevertheless, it is still needed to evaluate the modified gluten proteins in the bread using a simulated gastrointestinal digestion in vitro or in an in vivo model.
Conclusions
Supplementation of modified wheat flour using AnPEP with an amaranth flour blend is a good option to obtain gluten-reduced breads for consumers demanding less immunogenic gluten bread without additives and acceptable quality. The modified wheat flour with the amaranth flour blend allowed the production of bread with only 60 mg kg−1 immunoreactive gluten.
Conflicts of interest
There are no conflicts to declare.
Acknowledgements
The authors are grateful to DSM Nutritional Products, Mexico, for providing the AnPEP enzyme, MA Camacho-Casas from INIFAP for donating the wheat grain and to LC Montoya-Ballesteros, MC Granados-Nevarez, JR Valenzuela-Miranda, and K Chavez-Quiroz for technical support. The scholarship granted by CONACYT for Heredia-Sandoval NG (243029) is acknowledged. This work was supported by the Scientific Research and Technological Development Fund of CIAD, A. C. (grant number 80235, 2014).
Notes and references
- S. Valenti, D. Corica, L. Ricciardi and C. Romano, Ann. Med., 2017, 1–13 Search PubMed.
- T. Mazzeo, F. Brambillasca, N. Pellegrini, R. Valmarana, F. Corti, C. Colombo and C. Agostoni, Int. J. Food Sci. Nutr., 2014, 65(1), 112–116 CrossRef PubMed.
- G. Vici, L. Belli, M. Biondi and V. Polzonetti, Clin. Nutr., 2016, 35(6), 1236–1241 CrossRef CAS PubMed.
- E. Carini, F. Scazzina, E. Curti, F. Fattori, T. Mazzeo and E. Vittadini, Int. J. Food Sci. Nutr., 2015, 66(8), 867–872 CrossRef CAS PubMed.
- C. Rizzello, M. De Angelis, R. Di Cagno, A. Camarca, M. Silano, I. Losito and C. Gianfrani, Appl. Environ. Microbiol., 2007, 73(14), 4499–4507 CrossRef CAS PubMed.
- N. Heredia-Sandoval, A. Islas-Rubio, F. Cabrera-Chávez and A. Calderón de la Barca, Food Funct., 2014, 5(8), 1813–1818 CAS.
- F. Cabrera-Chávez, A. Islas-Rubio, O. Rouzaud-Sández, N. Sotelo-Cruz and A. Calderón de la Barca, J. Cereal Sci., 2010, 52(2), 310–313 CrossRef.
- N. Heredia-Sandoval, A. Calderón de la Barca and A. Islas-Rubio, J. Cereal Sci., 2016, 71, 73–77 CrossRef CAS.
- H. Wieser and P. Koehler, J. AOAC Int., 2012, 95(2), 356–363 CrossRef CAS PubMed.
- M. Šebela, P. Řehulka, J. Kábrt, H. Řehulková, T. Oždian, M. Raus, V. Franc and J. Chmelík, J. Mass Spectrom., 2009, 44(11), 1587–1595 CrossRef PubMed.
- O. Lowry, N. Rosebrough, A. Farr and R. Randall, J. Biol. Chem., 1951, 193, 265–275 CAS.
- I. Batey, R. Gupta and F. MacRitchie, Cereal Chem., 1991, 68, 207–209 CAS.
- G. Ellman, Arch. Biochem. Biophys., 1959, 82, 70–75 CrossRef CAS PubMed.
-
AACC, American Association of Cereal Chemists, Approved Methods of the AACC, St. Paul, MN, USA, 10th edn, 2000 Search PubMed.
- Alpha M.O.S., Sensors Range, Technical Note N-SAS-03, 2002.
- U. Laemmli, Nature, 1970, 227, 680–685 CrossRef CAS PubMed.
- A. Calderón de la Barca, G. Yepiz-Plascencia and T. Bøg-Hansen, Life Sci., 1996, 59(23), 1951–1960 CrossRef.
-
NcSS, Number Cruncher Statistical Systems, Kaysville, UT, USA, 2000 Search PubMed.
- L. Edens, P. Dekker, R. Van Der Hoeven, F. Deen, A. de Roos and R. Floris, J. Agric. Food Chem., 2005, 53(20), 7950–7957 CrossRef CAS PubMed.
- R. Bangur, I. Batey, E. McKenzie and F. MacRitchie, J. Cereal Sci., 1997, 25(3), 237–241 CrossRef CAS.
- A. Islas-Rubio, F. MacRitchie, S. Gandikota and G. Hou, Rev. Fitotec. Mex., 2005, 28(3), 243–251 Search PubMed.
- E. Lutz, H. Wieser and P. Koehler, J. Agric. Food Chem., 2012, 60(14), 3708–3716 CrossRef CAS PubMed.
- H. Wieser, Food Microbiol., 2007, 24(2), 115–119 CrossRef CAS PubMed.
- D. Andrews, R. Caldwell and K. Quail, Cereal Chem., 1995, 72(3), 326–329 CAS.
-
P. Sluimer, Principles of breadmaking functionality of raw materials and process steps, American Association of Cereal Chemists Inc., St. Paul, MN, USA, 2005 Search PubMed.
- O. Castellani, E. Martínez and M. Añón, J. Agric. Food Chem., 1999, 47(8), 3001–3008 CrossRef CAS PubMed.
- T. Traynham, D. Myers, A. Carriquiry and L. Johnson, J. Am. Oil Chem. Soc., 2007, 84(2), 151 CrossRef CAS.
-
C. Wrigley, J. Andrews, F. Bekes, P. Gras, B. Gupta, F. MacRitchie and J. Skerritt, in Interactions: The Keys of Cereal Quality, ed. R. J. Hamer and R. C. Hoseney, American Association of Cereal Chemists, Inc., St. Paul, MN, 1998, pp. 17–41 Search PubMed.
-
K. Preston, in Interactions: The Keys of Cereal Quality, ed. R. J. Hamer and R. C. Hoseney, American Association of Cereal Chemists, Inc., St. Paul, MN, 1998, pp. 81–90 Search PubMed.
-
W. Bushuk, in Interactions: The Keys of Cereal Quality, ed. R. J. Hamer and R. C. Hoseney, American Association of Cereal Chemists, Inc., St. Paul, MN, 1998, pp. 1–16 Search PubMed.
- C. Brites, M. Trigo, C. Santos, C. Collar and C. Rosell, Food Bioprocess Technol., 2010, 3(5), 707–715 CrossRef.
- E. Pagliarini, M. Laureati and V. Lavelli, Eur. Food Res. Technol., 2010, 231(1), 37–46 CrossRef CAS.
- N. O'Shea, E. Arendt and E. Gallagher, J. Food Sci., 2014, 79(6), 1067–1076 CrossRef PubMed.
- L. T. Sandri, F. G. Santos, C. Fratelli and V. D. Capriles, Food Sci. Nutr., 2017, 5(5), 1021–1028 CrossRef CAS PubMed.
- R. Baixauli, A. Salvador, G. Hough and S. M. Fiszman, Food Qual. Pref., 2008, 19(7), 628–635 CrossRef.
- E. Campo, L. del Arco, L. Urtasun, R. Oria and A. Ferrer-Mairal, J. Cereal Sci., 2016, 67, 75–82 CrossRef.
- V. D. Capriles, F. G. dos Santos and J. A. G. Arêas, J. Cereal Sci., 2016, 67, 83–91 CrossRef CAS.
- M. Pacyński, R. Z. Wojtasiak and S. Mildner-Szkudlarz, LWT–Food Sci. Technol., 2015, 63(1), 706–713 CrossRef.
- J. Pico, J. L. Bernal and M. Gómez, J. Food Sci. Technol., 2017, 54(6), 1433–1441 CrossRef CAS PubMed.
- Codex Alimentarius Commission, Draft revised standard for gluten-free foods, http://www.codexalimentarius.net accessed July 2017.
- G. Mazzarella, V. Salvati, G. Iaquinto, R. Stefanile, F. Capobianco, D. Luongo and M. Rossi, Clin. Dev. Immunol., 2012, 2012, 329150 Search PubMed.
- P. Shewry, A. Tatham, J. Forde, M. Kreis and B. Miflin, J. Cereal Sci., 1986, 4(2), 97–106 CrossRef CAS.
- Y. Wan, C. Gritsch, M. Hawkesford and P. Shewry, Ann. Bot., 2014, 113(4), 607–615 CrossRef CAS PubMed.
- F. Janssen, A. Pauly, I. Rombouts, K. J. Jansens, L. Deleu and J. A. Delcour, Compr. Rev. Food Sci. Food Saf., 2017, 16(1), 39–58 CrossRef CAS.
- C. Lamacchia, S. Chillo, S. Lamparelli, N. Suriano, E. La Notte and M. A. Del Nobile, J. Food Eng., 2010, 96(1), 97–106 CrossRef CAS.
- R. Ciccocioppo, A. Di Sabatino and G. Corazza, Clin. Exp. Immunol., 2005, 140(3), 408–416 CrossRef CAS PubMed.
|
This journal is © The Royal Society of Chemistry 2018 |
Click here to see how this site uses Cookies. View our privacy policy here.