DOI:
10.1039/C7FO01389B
(Paper)
Food Funct., 2018,
9, 294-306
Structure characterization of a novel polysaccharide from Hericium erinaceus fruiting bodies and its immunomodulatory activities
Received
7th September 2017
, Accepted 6th November 2017
First published on 9th November 2017
Abstract
A novel polysaccharide fraction (HEP-S) was extracted and isolated from the fruiting bodies of Hericium erinaceus. Structural characterization revealed that HEP-S had an average molecular weight of 1.83 × 104 Da and consisted of rhamnose, fucose, mannose, glucose and galactose at a molar ratio of 1.47
:
0.93
:
1.36
:
8.68
:
4.08. Periodate oxidation–Smith degradation and NMR analysis showed that the main linkage types of HEP-S were composed of (1→)-α-D-Glc, (1→3,4)-α-D-Glc, (1→6)-α-D-Gal, (1→3,4)-β-D-Man, (1→3,6)-α-Rha and (1→2)-β-L-Fuc. The immunomodulatory assay indicated that HEP-S could significantly enhance the pinocytic and phagocytic capacity and promote the secretion of nitric oxide and pro-inflammatory cytokines by activating the corresponding mRNA and protein expression in RAW 264.7 cells involving a toll-like receptor 2 membrane receptor. Besides, HEP-S was also found to improve the adaptive immune function by enhancing T and B lymphocyte proliferation and increasing the interleukin-2, interleukin-4 and interferon-γ secretion in spleen lymphocytes. These results suggested that HEP-S could be used as a potential immunoregulatory agent in functional foods.
1. Introduction
Hericium erinaceus (H. erinaceus) belongs to the Aphyllophorales order and Hydnaceae family, and is a traditional food source and medicinal fungus in Asian countries and even the USA and Europe.1 Recently, H. erinaceus has attracted great attention in the fields of functional food and biomedical science due to its various beneficial effects. Usually, polysaccharides are well documented to be a major active ingredient of H. erinaceus, which possess various pharmacological activities such as antitumor activity,2,3 anti-proliferation property,4 antioxidant property,4–6 hepatoprotective effect6 and anti-chronic atrophic gastritis activity.7 To date, a couple of polysaccharides with various bioactivities have been purified from the fruiting bodies and mycelia of H. erinaceus, but little information is available about their chemical structure and immunomodulatory activities.
For a living organism, immunity is defined as the ability to recognize and destroy external harmful substances,8 which plays a crucial role in our health. Classically, immune responses are divided into innate and adaptive immunity with different roles and functions. It is well known that the innate immune response is the first line of host protection in the defense against invading and infecting microbial pathogens and is mediated primarily by macrophages, granulocytes, monocytes and dendritic cells.9 The adaptive immune response, on the other hand, is responsible for the elimination of pathogens and for the generation of immunological memory.10,11 In most cases, macrophages and lymphocytes are usually used as the ideal cell models to evaluate the immunity of bioactive components.
Macrophages are distributed throughout phylogenetically conserved cells in all the multicellular organisms. They can neutralize foreign substances, cancer cells and infectious microbes through phagocytosis, chemotaxis, and surveillance and by releasing proinflammatory cytokines and cytotoxic components, such as nitric oxide (NO), tumor necrosis factor-alpha (TNF-α), reactive oxygen species (ROS), interleukin (IL)-6, etc.12–15 Meanwhile, as the major cellular components of the immune response, lymphocytes can constitute memory cells for a specific antigen, which can be activated after a process known as antigen presentation by antigen-presenting cells.16 Indeed, cell proliferation activity is one of the most important functions of lymphocytes, and the cellular proliferation induced by concanavalin-A (ConA) and lipopolysaccharide (LPS) is commonly used to detect T and B lymphocyte immunity, respectively.17 In particular, multiple cytokines, e.g. IL-2, IL-4 and IFN-γ, can be used to test the effect of modulation on lymphocytes.
In the present study, a novel polysaccharide fraction (HEP-S) was extracted, isolated and purified from the fruiting bodies of H. erinaceus and its primary structural characteristics including molecular weight, linkage information and monosaccharide composition were analyzed. The immunomodulatory activity of HEP-S was then examined using the murine macrophage RAW 264.7 cells and spleen lymphocyte model system. The effects on the pinocytic and phagocytic capacity, production of ROS, TNF-α, NO and IL-6, relevant messenger ribonucleic acid (mRNA) expression and protein expression of the cells were detected. Furthermore, the membrane receptors of HEP-S on the RAW 264.7 cell line were also explored. Finally, we also determined the secretion level of cytokines, including IL-2, IL-4 and IFN-γ, in spleen lymphocytes.
2. Materials and methods
2.1. Materials and chemicals
H. erinaceus was obtained from Pingnan H. erinaceus plantation (Pingnan country, Fujian Province, China). The RAW 264.7 cell line was purchased from the Type Culture Collection of the Chinese Academy of Sciences (Shanghai, China). DEAE-Sepharose fast flow and Sephadex G-100 were purchased from GE Healthcare Life Science (Piscataway, NJ). Standard monosaccharides (including glucuronic acid, rhamnose, arabinose, fucose, xylose, mannose, glucose, galactose and inositol) were obtained from Shanghai Yuanye Bio-Technology Co. Ltd (Shanghai, China). Dulbecco's modified Eagle's medium (DMEM), fetal bovine serum (FBS), phosphate-buffered saline (PBS, pH 7.4), streptomycin and penicillin were acquired from Gibco Life Technologies (Waltham, MA, USA). 3-(4,5-Dimethylthiazol-2-yl)-5-(3-carboxymethoxyphenyl)-2-(4-sulfophenyl)-2H-tetrazolium (MTS) was obtained from Shanghai Bestbio Biotechnology Inc. (Shanghai, China). LPS, laminarin, neutral red, 3-(4,5-dimethylthiazol-2-yl)-2,5-diphenyltetrazolium bromide (MTT), ConA, glycerol, erythritol, ethylene glycol, various dextran standards and bovine serum albumin (BSA) were obtained from Sigma-Aldrich Co. (St Louis, USA). Trizol was acquired from Invitrogen Life Technologies (California, USA). First Strand cDNA Synthesis Kit and FastStart Universal SYBR Green Master (ROX) were obtained from F. Hoffmann-La Roche Ltd (Basel, Switzerland). Mouse IL-2, IL-4, IL-6, TNF-α, and IFN-γ enzyme-linked immunosorbent assay (ELISA) kits were purchased from Neobioscience Technology Co., Ltd (Shenzhen, China). NO detecting kit was purchased from Nanjing Jiancheng Institute of Biotechnology (Nanjing, China). ROS assay kit was obtained from Beijing Solarbio Science and Technology Co., Ltd (Beijing, China). Anti-mannose receptor antibody (anti-MR), anti-scavenger receptor I antibody (anti-SR), anti-toll-like 2 antibody (anti-TLR2), anti-toll-like 4 receptor antibody (anti-TLR4), anti-beta glucan receptor antibody (anti-GR), anti-complement receptor 3 antibody (anti-CR3) and anti-TNF-α antibody were obtained from Abcam, Inc. (Cambridge, MA, USA). Antibodies against β-actin, iNOS and IL-6 were provided by Cell Signaling Technology (Beverly, MA, USA). All other chemicals and solvents used in the experiment were of analytical reagent grade.
2.2. Extraction and purification of polysaccharide from the fruiting bodies of H. erinaceus
The dried fruiting bodies of H. erinaceus were pulverized and passed through a 60 mesh sieve. The powder was extracted with distilled water at a ratio of 1
:
20 (w/v) at 85 °C twice (2.5 h each time). The aqueous extracts were centrifuged at 3000g for 20 min to remove the residue. The supernatant was collected and concentrated to one-fifth of the initial volume at reduced pressure and then was precipitated by ethanol addition to a final concentration of 75% (v/v) at 4 °C overnight. The precipitate was separated and collected by centrifugation at 5000g for 20 min. After that, the protein in the precipitate was removed by using the Sevag method. Finally, the precipitate was dialyzed in distilled water for 48 h at 4 °C and then freeze-dried to obtain a crude polysaccharide.
The crude polysaccharide (150 mg) was dissolved in 10 mL of ultrapure water and loaded onto a pre-equilibrated DEAE-Sepharose fast flow anion exchange column (3.5 cm × 20 cm). The column was eluted with distilled water and 0.05, 0.1, 0.2, 0.3 and 0.5 M NaCl solutions at 1.0 mL min−1. HEP-S (0.05 M NaCl salt elution) was dialyzed, freeze-dried and further purified by Sephadex G-100 column chromatography (1.6 cm × 60 cm). The sample was eluted with ultrapure water at a rate of 0.5 mL min−1. The elution was detected using the phenol–sulfuric acid method. Then the solution was dialyzed in distilled water for 48 h at 4 °C. After freeze-drying, HEP-S was available for the subsequent experiments.
2.3. Structure characterization of HEP-S
2.3.1. Molecular weight determination of HEP-S.
The molecular weight of HEP-S was determined by High-Performance Gel Permeation Chromatography (HPGPC). Briefly, 5 mg of the sample was weighed and dissolved in 1 mL of 0.02 M phosphate solution. After passing through a 0.45 μm filter, 20 μL of the solution was injected into a 1525 GPC system (Waters, USA). The chromatographic experiment was performed on a TSK-GEL-G-5000 PWXL column (300 mm × 7.8 mm) equipped with a TSK-GEL-G-3000 column (300 mm × 7.8 mm) from Tosoh Organic Chemical Co., Ltd (Tokyo, Japan). The GPC system was eluted with 0.02 M phosphate solution at 0.6 μL min−1 at a column temperature of 35 °C. A standard curve was obtained by using various standard dextrans (from 5.2 kDa to 668 kDa).
2.3.2. FT-IR spectrum and uronic acid content determination of HEP-S.
The freeze-dried HEP-S sample was ground and mixed with spectroscopic grade KBr powder. The mixture was pressed into a 1 mm pellet for FT-IR analysis with an Affinity-1 IR spectrophotometer (Shimadzu, Japan) within 4000–400 cm−1 at a resolution of 4 cm−1.
The meta-hydroxydiphenyl colorimetric method was used to detect the uronic acid content of polysaccharides using D-glucuronic acid as a standard.18
2.3.3. Monosaccharide composition analysis of HEP-S.
The monosaccharide composition of the HEP-S fraction was analyzed according to the reported method with a minor modification.19 Briefly, the HEP-S sample (10 mg) was hydrolyzed with trifluoroacetic acid (4 M, 2 mL) at 110 °C for 5 h. The acetylation of HEP-S was carried out by the reaction with 10 mg of hydroxylamine hydrochloride and 1 mL of pyridine at 90 °C for 30 min. Next, the resulting solution was mixed and incubated with 1 mL of acetic anhydride at 90 °C for 30 min.
The acetate derivative was analyzed using an Agilent 7890A gas chromatography system (Agilent Technologies, USA) with a HP-5 capillary column (30 m × 0.32 mm × 0.25 μm). The initial temperature was set at 150 °C, and was linearly increased to 200 °C at 2.5 °C min−1, and then maintained for 12 min. The injector and detector temperature was set at 250 °C. A set of monosaccharides (glucuronic acid, rhamnose, arabinose, fucose, xylose, mannose, glucose, and galactose) were used as standards and 1.0 mg mL−1 of inositol was used as the internal standard.
2.3.4. Periodate oxidation–Smith degradation of HEP-S.
The HEP-S sample was treated according to the reported method with some modifications.13 Briefly, HEP-S (20 mg) was dissolved in 10 mL of distilled water and then mixed with NaIO4 (30 mM, 10 mL) in the dark at room temperature. During the incubation period, the absorption at 223 nm was measured at different time intervals until the absorbance became invariable. After that, glycol (2 mL) was pipetted to terminate the periodate oxidation, and 2 mL of the above-treated solution was titrated with NaOH solution (0.01 M); then the rest of the solution was used for Smith degradation and extensively dialyzed against distilled water for 48 h. After that, NaBH4 (70 mg) was added to react in the dark at room temperature for 24 h. The solution was adjusted to pH 6.0 with acetic acid (50%) and then dialyzed for another 72 h. The collected residue was freeze-dried and then hydrolyzed with trifluoroacetic acid (4 M, 2 mL) for 5 h at 110 °C. After that, the hydrolysate was acetylated and analyzed using an Agilent 7890A chromatography system as mentioned above.
2.3.5. NMR spectroscopy analysis of HEP-S.
The sample of HEP-S (about 50 mg) was dissolved in 1 mL D2O in a NMR tube. 1H and 13C NMR spectra were recorded at 600 and 150 MHz, respectively, on a Bruker NMR spectrometer (AVANCE III HD 600, Bruker, Germany) at 25 °C. Two-dimensional spectra (heteronuclear single quantum coherence, HSQC, and heteronuclear multiple bond correlation, HMBC) were recorded using the standard Bruker pulse procedures.
2.4. Effects of HEP-S on lymphocyte immunomodulatory activities
2.4.1. Animals.
Female Balb/c mice (4–6 weeks old, 12–15 g) were obtained from the Laboratory Animal Center of Sun Yat-Sen University, Guangzhou, China (Certification number: SYXK 2007-0081). All experiments were performed in compliance with the Guide for the Care and Use of Laboratory Animals published by the U.S. National Institutes of Health (NIH Publication 85-23, 1996). All procedures were approved by the Ethical Committee of South China University of Technology, China.
2.4.2 Preparation of spleen lymphocytes.
Spleens from the mice were passed through a 200 mesh stainless steel sieve to obtain a homogeneous cell suspension under aseptic conditions. Then the cell suspension was washed and centrifuged at 1000g for 5 min to obtain the cell pellet. Red blood cells were lysed with 0.83% ammonium chloride lysis solution and centrifuged at 200g for 10 min. The collected lymphocytes were washed three times with PBS and then suspended and incubated at 37 °C for 4 h. Finally, the suspended cell populations were collected for the subsequent experiments.
2.4.3. Determination of lymphocyte proliferation.
The effects of HEP-S on the cell proliferation of lymphocytes were evaluated by using the MTS assay.20 Lymphocytes were seeded into 96-well culture plates at a concentration of 5 × 104 cells per well and incubated at 37 °C for 24 h. Then, the cells were treated with 100 μL of HEP-S (at final concentrations of 100, 250, 500, and 1000 μg mL−1), HEP-S + ConA (at a final concentration of 5 μg mL−1) or HEP-S + LPS (at a final concentration of 10 μg mL−1). Serum free culture medium was used as the control. After 24 h incubation, 20 μL of MTS was pipetted into each well and incubated at 37 °C for 1 h. Then the absorbance at 490 nm was measured using a microplate reader (Biotek, USA). The cell proliferation was calculated as follows: cell proliferation ratio (%) = A/B × 100%, where A is the absorbance of the treated group and B is the absorbance of the control group.
2.4.4. Effects of HEP-S on IL-2, IL-6 and IFN-γ secretion in lymphocytes.
Lymphocytes were seeded into 96-well culture plates at a concentration of 5 × 105 cells per well and incubated at 37 °C for 48 h. Then the cells were treated with 100 μL of HEP-S at different final concentrations (100, 250, 500, and 1000 μg mL−1). Serum free culture medium was used as the control group. After 24 h incubation, the supernatants of the spleen lymphocytes were collected and the levels of IL-2, IL-4 and IFN-γ secretion were determined by using ELISA kits.
2.5. Effects of HEP-S on macrophage immunomodulatory activities
2.5.1. MTT assay.
The effect of HEP-S on the viability of RAW 264.7 cells was investigated by using the MTT assay. Briefly, 5 × 103 cells in 100 μL per well were plated into 96-well plates for 24 h and then treated with different concentrations of HEP-S (100–1000 μg mL−1) or LPS (25 μg mL−1) for another 24 h. Serum free culture medium was used as the control group. After that, 20 μL of MTT solution (5 mg mL−1 in PBS) was pipetted into each well for 4 h and then dissolved in 100 μL of DMSO solution. Then the cell plate absorbance was determined by using a VICTOR X microplate reader (PerkinElmer Inc., Waltham, MA, USA) at 570 nm. The cell viability was calculated as follows: cell viability (%) = A/B × 100%, where A is the absorbance of the treated group and B is the absorbance of the control group.21
2.5.2. Determination of pinocytic activity.
The effects of HEP-S on the pinocytic activity of RAW 264.7 macrophages were measured by using the neutral red assay. RAW 264.7 macrophages (1 × 106 cells per mL) were loaded into 96-well plates. After 24 h incubation, DEME medium, LPS (25 μg mL−1), or different concentrations of HEP-S (100, 250, 500, and 1000 μg mL−1) were pipetted into each well for another 24 h incubation. Then the medium was carefully removed, and 100 μL of 0.1% neutral red was added into each well and incubated for 1 h. After being washed with PBS three times, each of the wells was loaded with 100 μL of cell lysis solution (glacial acetic acid
:
ethanol = 1
:
1). The cell culture plate was statically placed for 2 h at room temperature. The absorbance at 540 nm was measured using a microplate reader (Biotek, USA).
2.5.3. Determination of phagocytic uptake.
The phagocytic capacity of macrophages was measured with a Vybrant Phagocytosis Assay Kit (FITC-labeled E. coli) using a fluorescence microscope.12 The cells (1 × 106 cells per mL) were seeded in 96-well plates for 24 h and then incubated with HEP-S at different concentrations (100, 250, and 1000 μg mL−1) or LPS (25 μg mL−1) for 24 h. After incubation, the medium was replaced with 100 μL of FITC-labeled E. coli (1 mg mL−1 in PBS) provided in the Vybrant Phagocytosis Assay Kit and incubated at 37 °C for 30 min. Phagocytosis was then terminated by the addition of 200 μL of ice-cold prepared trypan blue suspension, and the cells were washed three times with cold PBS. After fixation with 50 μL of 4% paraformaldehyde solution, the images of the cells were obtained using a fluorescence microscope (AXIOVERT 200, Zeiss, Germany).
2.5.4. Measurement of NO, TNF-α and IL-6 cytokine secretion.
Macrophages (1 × 106 cells per mL) were pipetted into 96-well plates and incubated with HEP-S at different concentrations as mentioned above. After the incubation, the supernatants were harvested for the subsequent experiments. The macrophage NO content was determined based on the Griess method. The levels of TNF-α and IL-6 were determined by using commercial ELISA kits according to the manufacturer's instructions.
2.5.5. Measurement of ROS generation.
ROS production by RAW 264.7 cells was measured using 2,7-dichlorofluorescin diacetate (DCFH-DA). Macrophages (1 × 106 cells per mL) were seeded into 96-well plates for 24 h. Next, the cells were incubated with DMEM culture medium, HEP-S (100, 250, 500, and 1000 μg mL−1) or LPS (25 μg mL−1). After 24 h incubation, all culture medium was carefully removed, followed by the addition of 100 μL DCFH-DA (10 μM) dissolved in the serum free medium for 20 min at 37 °C. Then, all dyes in the supernatant were removed, and the cells were washed three times with PBS. Fluorescence intensity was immediately detected at 488 nm excitation and at 525 nm emission using a microplate reader (Infinite M200 Pro, Tecan, Austria).
2.5.6. RT-PCR analysis.
Macrophages were treated with different concentrations of HEP-S or LPS as mentioned above for 24 h. Then the cells were collected and washed three times with cold PBS for RNA extraction. The total RNA was isolated using Trizol reagent and then was reversed into cDNA according to the previously reported methods.19 The reverse transcriptase-generated complementary DNA encoding TNF-α, iNOS, and IL-6 genes were amplified by quantitative real-time PCR assay (QPCR). The nucleotide sequences of the specific primers were used according to the sequences reported in the previous studies.22 Glyceraldehyde-3-phosphate dehydrogenase (GAPDH) was used as the internal reference. The expression levels of mRNA were calculated by using the 2−ΔΔCt method. The treated group without HEP-S was the negative control, and the treated group with LPS (25 μg mL−1) was the positive control group. Gene amplification was performed using an ABI StepOne Real-Time PCR system (Thermo Fisher Scientific Inc., USA).
2.5.7. Investigation of membrane receptors.
RAW 264.7 macrophages (1 × 106 cells per mL) were loaded into 96-well plates. After 24 h incubation, the cells were pre-treated with antibodies (anti-MR, anti-SR, anti-CR3, anti-GR, anti-TLR4, and anti-TLR2 at a concentration of 5 μg mL−1) and laminarin (the inhibitor of dectin-1, at a concentration of 500 μg mL−1) for 1 h prior to stimulation with HEP-S (500 μg mL−1). The serum free culture medium was the negative control group, and the treated group with LPS (25 μg mL−1) was the positive control group. After treatments, the supernatants of the cells were collected and the levels of NO, IL-6, and TNF-α were analyzed by using the methods as described above.
2.5.8. Western blot analysis.
Western blot analysis of iNOS, IL-6, TNF-α, and β-actin protein levels in RAW 264.7 cells was performed according to the previously reported method.23 The densitometry of each protein was performed using a chemiluminescence (ECL) kit (Millipore Co., Billerica, MA, USA). The densitometry data for each kinase were shown in graphic form.
2.6. Statistical analysis
The data were expressed as means ± standard deviation (S ± D) for three replicates. One way ANOVA was used to analyze the significant differences between the groups by SPSS 20.0. p < 0.05 was accepted as the level of significance.
3. Results and discussion
3.1. Extraction, purification and structural characterization of HEP-S
Crude polysaccharides were isolated from the dried fruiting bodies of H. erinaceus with a yield of 2.56 ± 0.14% (w/w). After purification with a DEAE-Sepharose fast flow column (Fig. 1a), two major fractional peaks, eluted with distilled water (HEP-W) and 0.05 M NaCl (HEP-S), were obtained. In this study, we mainly focused on the HEP-S fraction, and the other HEP-W fraction will be studied in our future work. Fig. 1(b) shows that HEP-S appeared as a single and symmetrical peak after being further purified by Sephadex G-100 column chromatography. After dialysis and lyophilization, the total sugar content and uronic acid content of HEP-S were detected to be 92.53% ± 0.78% (w/w) and 0.56% ± 0.08%, respectively.
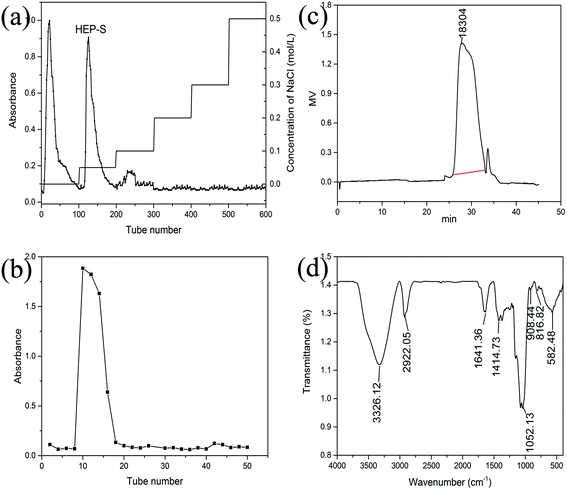 |
| Fig. 1 Chromatograms of HEP-S from H. erinaceus from (a) DEAE-Sepharose fast flow chromatography, (b) Sephadex G-100 chromatography and (c) HPGPC, and (d) FT-IR spectrum. | |
3.1.1. Molecular weight of HEP-S.
As shown in Fig. 1(c), a single absorption peak was observed and the average molecular mass of HEP-S was estimated to be 1.83 × 104 Da according to the dextran standard curve. This result was quite different from the previous research by Wang et al., who found that there were two polysaccharide fractions (HPA and HPB) in the fruiting body of H. erinaceus with molecular weights of 5.0 × 104 and 3.0 × 104 Da, respectively.24 The differences might be due to the different extraction methods, varieties and habitats of the studied H. erinaceus.
3.1.2. FT-IR spectrum.
Fig. 1(d) shows the infrared spectrum, in which most of the absorption bands could be assigned according to the previous literature reports.12,13 The strong and broad absorption peaks at 3326.12, 2922.05 and 1641.36 cm−1 belonged to the stretching vibration of the O–H, C–H and bound water, respectively. Meanwhile, the absorption peaks at 1414.73 cm−1 and 1052.13−1 indicated the presence of the stretching vibrations of the C
C stretching and pyranose ring, respectively. A characteristic absorption at 908.44 cm−1 was also observed, indicating the β-pyranoside linkage. A characteristic peak at 582.48 cm−1 indicated the existence of α-configurations.
3.1.3. Monosaccharide composition of HEP-S.
As shown in Fig. 2(b), the polysaccharide HEP-S was found to mainly consist of rhamnose, fucose, mannose, glucose and galactose at a molar ratio of 1.47
:
0.93
:
1.36
:
8.68
:
4.08, indicating that HEP-S was a kind of a heteropolysaccharide with glucose occupying the biggest proportion. These results were different from that of Zhang et al., who found that the polysaccharide from H. erinaceus consisted of fucose, galactose and glucose at a molar ratio of 1
:
4
:
1.25
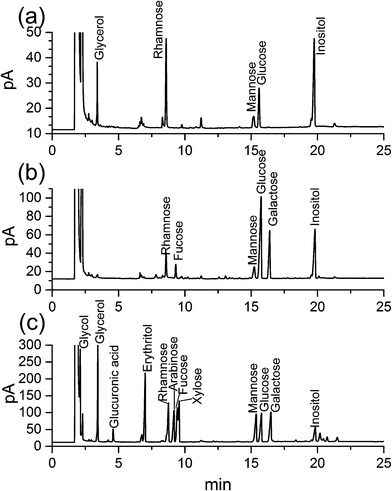 |
| Fig. 2 (a) Gas chromatograms (GC) of HEP-S after Smith degradation; (b) GC of the monosaccharide composition of HEP-S and (c) GC of the standard monosaccharides. | |
3.1.4. Periodate oxidation–Smith degradation analysis.
The type of glycosidic linkage in HEP-S was preliminarily investigated by the consumption of NaIO4 and the generation of HCOOH during periodate oxidation–Smith degradation. The results showed that every molar of the sugar residue consumed 1.162 mol of NaIO4 and generated 0.422 mol of HCOOH. The mole number of periodate was two times more than that of formic acid, indicating the existence of (1→), (1→6), (1→2,4), (1→3,4) and (1→2) linkage in HEP-S.
After further Smith degradation of the oxidized HEP-S, glycerol, rhamnose, mannose, glucose were detected by GC at a molar ratio of 2.05
:
3.43
:
0.96
:
1.99 (Fig. 2a). The presence of glycerol indicated the existence of (1→), (1→6) and (1→2)-linked glycosidic bonds. In addition, the presence of monosaccharides revealed the appearance of (1→3), (1→3,4) and (1→3,6)-linked glycosidic bonds.26,27
3.1.5. NMR analysis of HEP-S.
1H NMR, 13C NMR, HSQC and HMBC spectra (Fig. 3) were used to confirm the structure of HEP-S. Signals in the 1H and 13C spectra of HEP-S were completely assigned based on the HSQC experiments (Fig. 3(c)), while the anomeric relevant C–H signals of HEP-S were measured based on the HMBC spectrum (Fig. 3(d)). Moreover, the signals of HEP-S on the NMR spectra were further assigned on the basis of monosaccharide composition, glycosidic linkage analysis, and chemical shifts and were analyzed according to the results reported in the previous studies.28–36 Hence, the resonance signals between δ 95.0 and 110.0 in 13C NMR belonged to the anomeric carbon atoms of monosaccharides. The anomeric proton signal (δ 5.35, 5.17, 5.02, 4.68, 4.94, and 4.59) and the anomeric carbon proton signals (δ 98.17, 102.67, 102.66, 98.97, 98.05, and 100.26) corresponded to H-1 and C-1 of six anomeric residues, including (1→)-α-D-Glc (A), (1→3,4)-α-D-Glc (B), (1→6)-α-D-Gal (C), (1→3,4)-β-D-Man (D), (1→3,6)-α-Rha (E), and (1→2)-β-L-Fuc (F), respectively. Based on the data from 1H NMR and 13C NMR spectra, all the assignments of proton and 13C resonances are summarized in Table 1.
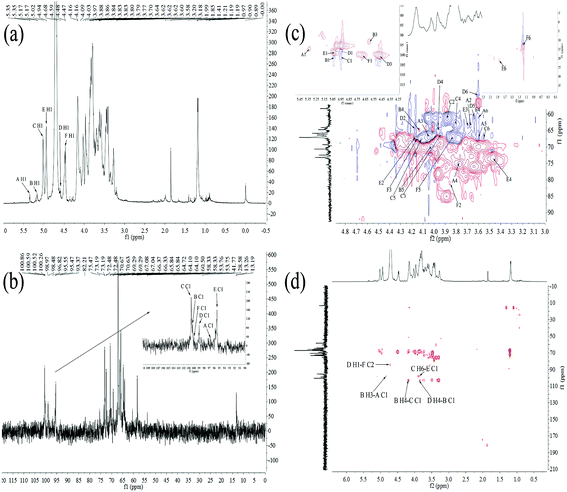 |
| Fig. 3
1H NMR spectrum (a), 13C NMR spectrum (b), HSQC spectrum (c) and HMBC spectrum (d) of HEP-S. | |
Table 1
1H and 13C NMR chemical shifts of HEP-S (δ, ppm)
Residue |
H1/C1 |
H2/C2 |
H3/C3 |
H4/C4 |
H5/C5 |
H6/C6 |
α-D-Glc-(1→A |
5.35/98.17 |
3.58/65.80 |
3.97/67.08 |
3.77/75.47 |
3.84/67.08 |
3.64/60.63 |
→3,4)-α-D-Glc-(1→B |
5.17/102.67 |
—/— |
4.81/98.48 |
4.16/64.10 |
4.09/69.29 |
—/— |
→6)-α-D-Gal-(1→C |
5.02/102.66 |
3.86/58.33 |
4.09/66.35 |
3.84/64.72 |
3.97/67.00 |
3.83/67.04 |
→3,4)-β-D-Man-(1→D |
4.68/98.97 |
4.14/64.72 |
4.47/100.52 |
3.84/66.37 |
3.64/60.50 |
3.70/53.75 |
→3,6)-α-Rha-(1→E |
4.94/98.05 |
4.15/66.33 |
3.80/65.84 |
3.20/73.19 |
—/— |
1.85/28.58 |
→2)-β-L-Fuc-(1→F |
4.59/100.26 |
3.88/82.21 |
4.17/66.33 |
3.62/60.50 |
3.86/65.80 |
1.21/13.26 |
In the 1H–13C HMBC spectrum of HEP-S (Fig. 3(d)), cross signal 3.83/98.05 ppm was attributed to C-1 (δ 98.05 ppm) for residue E and H-6 (δ 3.83 ppm) for residue C, indicating that C-1 of residue E was linked to O-6 of residue C.37 Meanwhile, the signals of C-1 at 102.66 ppm of C were related to the H-4 signals at 4.16 ppm of B, indicating the presence of →6)-α-D-Galp-(1→4)-α-D-Glcp-(1→. The cross signal of C-1 at 102.67 ppm of B and H-4 at 3.84 ppm of D showed the presence of →3,4)-α-D-Glcp-(1→4)-β-D-Manp-(1→. The C-2 signal at 82.21 ppm of F was correlated with the H-1 signal at 4.68 ppm of D. Thus, it was possible to conclude that a repeating unit of HEP-S contained a backbone structure of E-(1→6)-C-(1→4)-B-(1→4)-D-(1→2)-F. Meanwhile, the presence of the cross signal 4.81/98.17 ppm indicated that C-1 (δ 98.17 ppm) of residue A was linked to O-3 (δ 4.81 ppm) of residue B. Furthermore, the chemical shifts at 170–180 ppm were also detected in the HMBC spectrum (Fig. 3(d)), further indicating the presence of uronic acid.38
Based on the above results, HEP-S could be identified to be a branched polysaccharide and the main backbone of HEP-S was identified as →3,6)-α-Rhap-(1→6)-α-D-Galp-(1→4)-α-D-Glcp-(1→4)-β-D-Manp-(1→2)-β-L-Fucp-(1→ with a branch that was composed of α-D-Glcp-residues. The structure was markedly different from other reported polysaccharides derived from the fruiting bodies of H. erinaceus. For example, a heteropolysaccharide (HEPF1)25 was confirmed to contain a (1→6)-linked α-D-galactopyranosyl backbone with branches that were composed of fucose attached to O-2, it also contained 6-O-substituted-β-D-oligoglucosyl units and a minor terminal 3-O-methyl rhamnose residue. In fact, different structural characteristics might contribute to different bioactivities of H. erinaceus polysaccharides.
3.2. Immunomodulatory activities of HEP-S on spleen lymphocytes
3.2.1. Effect of HEP-S on spleen lymphocyte proliferation.
As shown in Fig. 4(a), the lymphocyte proliferation appeared to be significantly increased in the HEP-S treated group in a dose-dependent manner as compared to the control group (p < 0.01). Meanwhile, there was also a marked increase in the ConA- or LPS-induced proliferation in the treated lymphocytes. These data suggested that HEP-S could improve the cell immune function through the proliferation of T and B lymphocytes.
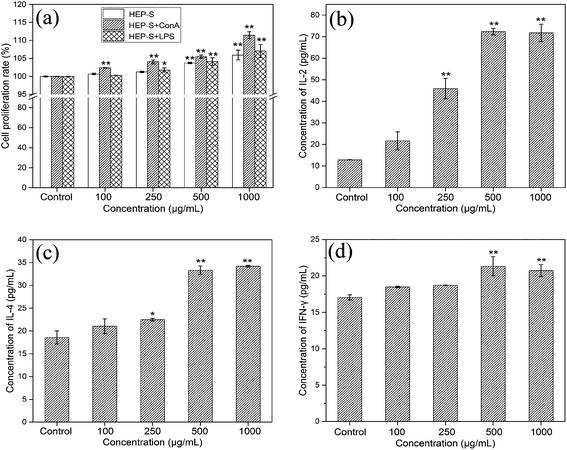 |
| Fig. 4 Effects of HEP-S at different concentrations on spleen lymphocytes: (a) cell proliferation ratio; (b–d) effects of HEP-S on the secretion of IL-2, IL-4 and IFN-γ cytokines. Significant differences with the negative control group are designated as *p < 0.05 and **p < 0.01. The data are expressed as means ± SD (n = 3). | |
3.2.2. Effects of HEP-S on IL-2, IL-4 and IFN-γ secretion in spleen lymphocytes.
Cytokines are intercellular signaling proteins that are involved in the differentiation, maturation and activation of cells; thus, monitoring the levels of cytokine secretion is a powerful approach to evaluate the activity on immune response.16 In this study, the secretion of cytokines was measured and compared by using ELISA kits. As shown in Fig. 4(b–d), HEP-S significantly promoted the secretion of IL-2, IL-4 and IFN-γ at different concentrations from 100 μg mL−1 to 1000 μg mL−1 in a dose-dependent manner. Compared to the control group, the cells treated with low concentrations of HEP-S (at 100 μg mL−1 and 250 μg mL−1) secreted little cytokines; when the concentration of HEP-S reached 500 μg mL−1, the production of IL-2, IL-4 and IFN-γ in the treated cells was increased by 459.55%, 79.28% and 25.16%, respectively (p < 0.01). These results demonstrated that the polysaccharide HEP-S can activate lymphocytes and stimulate cell proliferation as well as cytokine secretion in vitro.
3.3. Immunomodulatory activities of HEP-S on RAW 264.7 cells
3.3.1. Effect of HEP-S on the viability of RAW 264.7 cells.
To evaluate the toxic effect of HEP-S on murine macrophages, cell viability was detected by using the MTT assay. As shown in Fig. 5(a), no significant changes in the viability of cells treated with different concentrations of HEP-S were observed, even at a high concentration of 1000 μg mL−1. Hence, the concentration of HEP-S for the subsequent experiments was below 1000 μg mL−1.
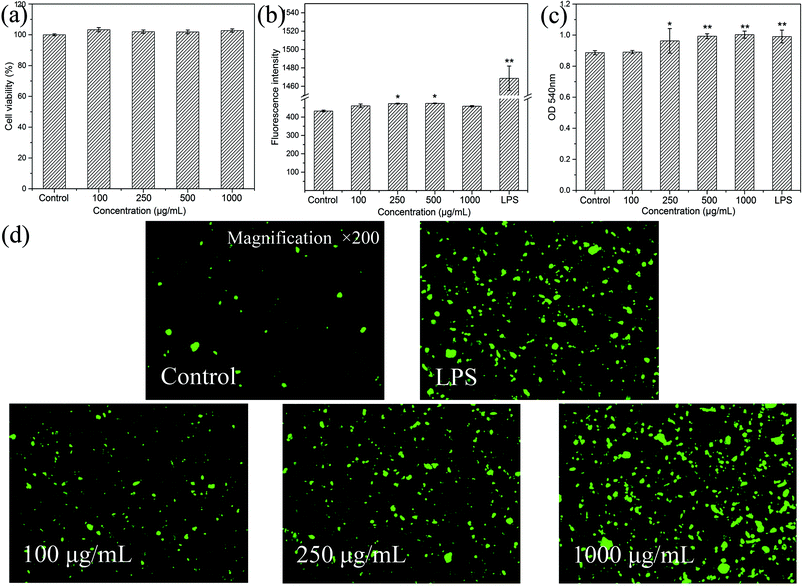 |
| Fig. 5 Effects of HEP-S at different concentrations on RAW 264.7 macrophages: (a) cell viability; (b) intracellular ROS generation; (c) effect of HEP-S on taking up neutral red of cells and (d) fluorescence microscopy images (10 × 20) of cells phagocytosing FITC-labeled E. coli. Significant differences with the negative control group are designated as *p < 0.05 and **p < 0.01. The data are expressed as means ± SD (n = 3). | |
3.3.2. Effect of HEP-S on ROS production in RAW 264.7 cells.
The generation of ROS by HEP-S treated RAW 264.7 cells was assessed by measuring DCF fluorescence intensity (Fig. 5(b)). When compared with the control group (433.00 ± 4.35), the fluorescence intensity of the cells treated with HEP-S at 250 μg mL−1 and 500 μg mL−1 was significantly increased (473.33 ± 2.31, 474.667 ± 1.52, respectively, p < 0.05), indicating that HEP-S treatment enhanced the up-regulation of intracellular ROS production. Some researchers have indicated that the level of ROS is correlated with the generation and secretion of inflammatory cytokines and chemokines.39,40 In particular, a variety of polysaccharides have been reported to be capable of enhancing the immune function via ROS regulation in mitochondria.23,39 Based on the results in this study, we can also conclude that a better capacity to increase ROS production in mitochondria could be one of the reasons for HEP-S to stimulate a strong immunomodulatory activity on RAW 264.7 cells.
3.3.3. Effects of HEP-S on the pinocytic and phagocytic capacity of RAW 264.7 cells.
One of the most distinguished features of macrophage activation is the increase in the pinocytic and phagocytic activity.41 Compared to the control group, the absorbance intensity of the cells treated with HEP-S was significant increased in a dose-dependent manner (Fig. 5(c)). In addition, we also determined the effect of HEP-S on the phagocytic capacity to take up FITC-labeled E. coli by RAW 264.7 cells. The fluorescence microscopy images showed a greater phagocytic uptake of FITC-labeled E. coli by cells after treatment with HEP-S or LPS than by the untreated cells (Fig. 5(d)). These results suggested that HEP-S can enhance the pinocytic and phagocytic capacity of RAW 264.7 cells in a certain concentration range.
3.3.4. Effects of HEP-S on NO, IL-6 and TNF-α secretion and mRNA and protein expression in RAW 264.7 cells.
It is well known that macrophages can defend against microbial infections and other external pathogens by secreting NO and a variety of inflammatory cytokines such as IL-6, IL-12, IL-1β, TNF-α, etc.13,19 In this study, the effects of HEP-S on NO, IL-6 and TNF-α secretion levels of RAW 264.7 cells were analyzed. As shown in Fig. 6(a–c), compared to the control group, HEP-S (at 100–1000 μg mL−1) significantly stimulated the cell secretion of NO, IL-6 and TNF-α in a clearly dose-dependent manner (p < 0.05). Specifically, the levels of NO, IL-6 and TNF-α treated with 500 μg mL−1 HEP-S were 89.29%, 94.61% and 36.58% of LPS (25 μg mL−1), respectively. Meanwhile, the concentrations of NO, IL-6 and TNF-α after treatment with 100 μg mL−1 HEP-S were detected to be 14.41 μM, 6177.50 pg mL−1 and 993.42 pg mL−1 higher than those in the control group, respectively. The results demonstrated that HEP-S exhibited significant immune-stimulating activities by up-regulating the NO, IL-6 and TNF-α secretion in RAW 264.7 cells. Similarly, Lee et al.1 reported a water-soluble polysaccharide fraction (HEB-AP Fr-I), purified from the liquid culture broth of H. erinaceus, which was also able to stimulate the secretion of NO and inflammatory cytokines in murine macrophages at specific concentrations.
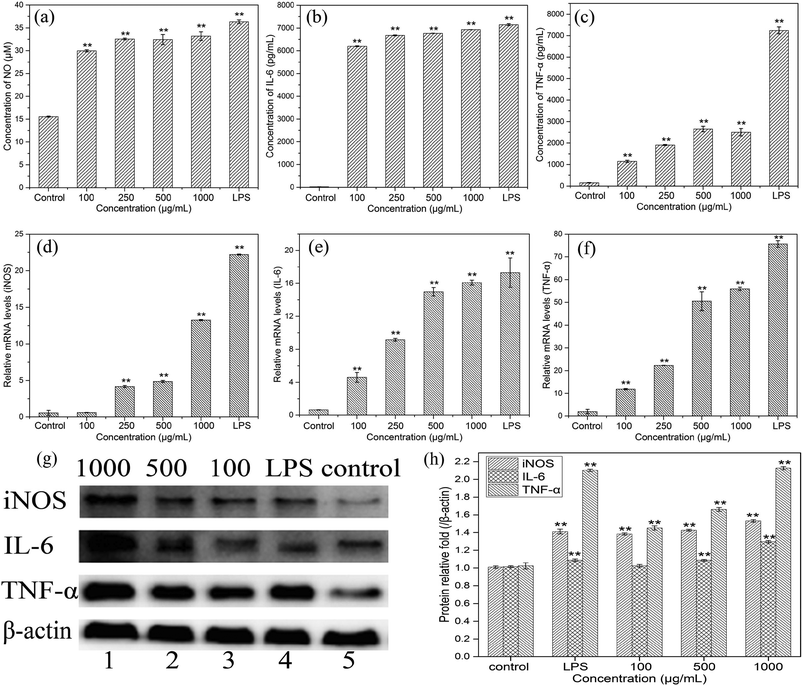 |
| Fig. 6 Effects of HEP-S at different concentrations on RAW 264.7 macrophages: (a–c) NO, IL-6 and TNF-α secretion levels; (d–f) iNOS, IL-6 and TNF-α mRNA expression levels; and (g–h) iNOS, IL-6 and TNF-α protein expression levels. Values are normalized to the level of β-actin. Significant differences with the negative control group are designated as *p < 0.05 and **p < 0.01. The data are expressed as means ± SD (n = 3). | |
To evaluate the effects of HEP-S on the mRNA expression of inducible nitric oxide synthase (iNOS) and cytokines in RAW 264.7 cells, the gene transcription of iNOS, IL-6 and TNF-α was further examined by RT-PCR. As shown in Fig. 6(d–f), compared to the control group, the cells treated with HEP-S or LPS also showed a significant increase in the mRNA levels of iNOS, IL-6 and TNF-α, which was consistent with the NO, IL-6 and TNF-α secretion levels.
In order to further elucidate the effects of HEP-S on the protein expression of iNOS, IL-6 and TNF-α in RAW 264.7 cells, western blot analysis was used in this study. As shown in Fig. 6(g and h), compared to the negative control group (lane 5), the cells treated with HEP-S or LPS showed significant expression in protein levels (p < 0.05). The results suggested that the increase in the NO, IL-6 and TNF-α secretion levels in the activated murine macrophages might be due to the enhancement of the corresponding mRNA and protein expression.
3.3.5. Pattern recognition receptors of HEP-S in RAW 264.7 cells.
It has been reported that macrophage activation is considered to be mediated primarily based on the recognition of pathogen-associated molecular patterns (PAMPs) through a limited number of pattern recognition receptors (PRRs).10,12,13,42 Studies have also indicated that macrophages might bind polysaccharides and exhibit their immunomodulating effects mainly through toll-like receptors (TLR2 and TLR4), complement receptor 3 (CR3), mannose receptor (MR), beta glucan receptor (GR), scavenger receptor I (SR) or dectin-1.13,43,44
To determine whether TLR2, CR3, TLR4, MR, GR, SR and dectin-1 play a role in HEP-S-mediated stimulation in RAW 264.7 cells, these antibodies were used in this study. The results are shown in Fig. 7(a–c). It was found that after the treatment of anti-TLR2 and HEP-S, the cells showed a significant decrease in the levels of NO, IL-6, and TNF-α as compared to the group only treated with HEP-S (p < 0.01). However, no significant decrease in the levels of NO, IL-6, and TNF-α was observed in the groups treated with anti-CR3, anti-TLR4, anti-MR, anti-GR, anti-SR and laminarin (dectin-1 inhibitor). Meanwhile, it was noted that the levels of NO, IL-6, and TNF-α in the group treated with anti-TLR2 were still maintained significantly higher than those in the control group (p < 0.01), suggesting that TLR2 was one of the pattern recognition receptors for HEP-S in macrophages to induce transcriptional activation and expression of cytokines, while other immune receptors also exist. Indeed, these seven receptors were a small part of RPPs on macrophages, so the receptors of HEP-S need to be further investigated in our future work.
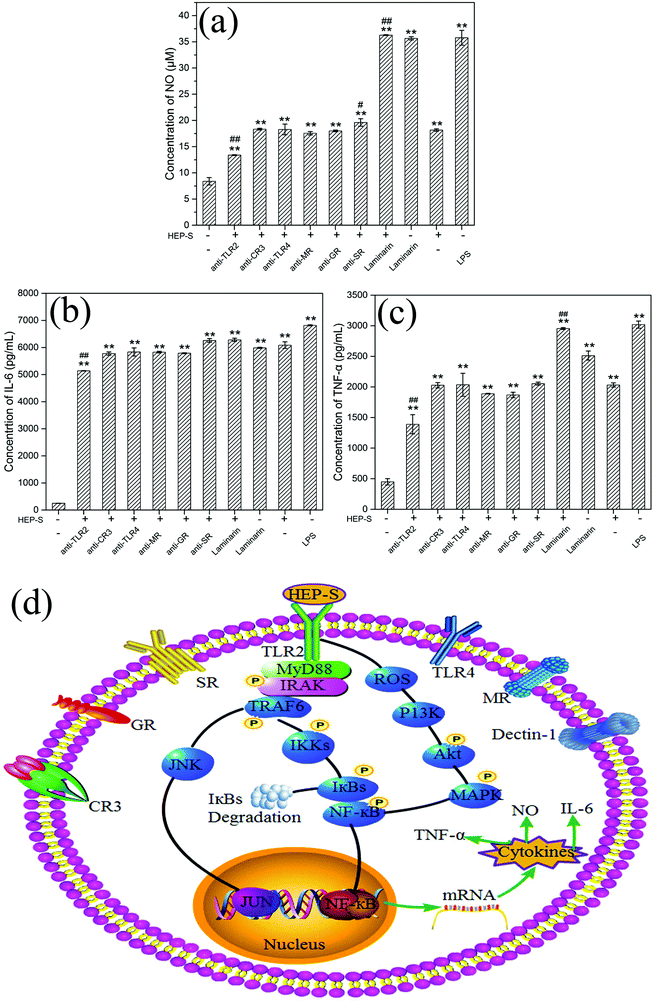 |
| Fig. 7 Roles of TLR2, CR3, TLR4, MR, GR, SR and dectin-1 on NO (a), IL-6 (b) and TNF-α (c) secretion in RAW 264.7 cells. Significant differences are designated as *p < 0.05 and **p < 0.01, the groups treated with antibodies versus the negative control group (without HEP-S); #p < 0.05 and ##p < 0.01, the groups treated with antibodies versus the group treated with only HEP-S (500 μg mL−1). The data are expressed as means ± SD (n = 3). (d) The proposed signal transduction pathways involved in macrophage activation by HEP-S. | |
It has been reported that TLR2 can interact with a series of microbial ligands and macromolecules, which subsequently allows macrophages to exhibit self-polarization and differentiation to further promote the maturation and development of immunity.45,46 A number of studies have demonstrated the TLR2-dependent activation of macrophages by mushroom polysaccharides. For instance, Xu et al. reported that a new heteropolysaccharide fraction from Lentinula edodes (L2) stimulated IL-6 levels via the TLR2 activation of the signaling pathway in RAW 264.7 cells.43 Gantner et al. also indicated that lentian exhibited its immunomodulating effects via binding with TLR2.47
Based on the previous study,48 TLR2 has been proven to be involved in the activation of IL-1R-associated kinase (IRAK) via an adaptor myeloid differentiation protein 88 (MyD88) with the subsequent activation of TNF receptor associated factor 6 (TRAF-6), Jun N-terminal kinase (JNK) and nuclear factor (NF)-κB. Meanwhile, increasing evidence indicated that ROS could not only play roles in host defense but also act as a cellular secondary messenger in many biological functions, such as transcription factor activation, protein phosphorylation and cytokine production.49–51 Yu et al. indicated that ROS was involved in the activation of phosphoinositide-3-kinase (PI3K), activation of the protein kinase B (Akt) and mitogen-activated protein kinase (MAPK), and NF-κB signal transduction pathways (PI3K/Akt/MAPKs/NF-κB pathway).52 The activation of these transcription pathways leads to gene transcription and the production of iNOS and cytokines. Based on these discussions, we can infer that the possible mechanism of HEP-S-induced macrophage immunomodulatory activity is mainly via two signaling pathways (Fig. 7).
4. Conclusions
In this study, a new fraction of a heteropolysaccharide (HEP-S) with an average molecular weight of 1.83 × 104 Da was isolated from the fruiting bodies of Hericium erinaceus and its primary chemical structure was characterized. The bioactivity tests showed that HEP-S possessed a significant immunomodulatory activity by enhancing the pinocytic and phagocytic capacity and increasing NO, IL-6, and TNF-α secretion by activating the corresponding mRNA expression and protein expression in RAW 264.7 cells, and TLR2 was confirmed to be one of the major membrane receptors of HEP-S. Besides, HEP-S was also found to improve the adaptive immune function by enhancing T and B lymphocyte proliferation and increasing the IL-2, IL-4 and IFN-γ secretion in spleen lymphocytes. These results suggested that HEP-S can function as an immunostimulator to stimulate both the innate and adaptive immune responses and might be a potential candidate for application in immunological diseases and functional foods.
Funding
The authors are grateful for the financial support from the Guangdong Provincial Department of Science and Technology, China (Grant No. 2013B090600008).
Conflicts of interest
The authors declare no conflicts of interest in this research manuscript.
References
- J. S. Lee, J. Y. Cho and E. K. Hong, Study on macrophage activation and structural characteristics of purified polysaccharides from the liquid culture broth of Hericium erinaceus, Carbohydr. Polym., 2009, 78, 162–168 CrossRef CAS.
- J. S. Lee and E. K. Hong,
Hericium erinaceus, enhances doxorubicin-induced apoptosis in human hepatocellular carcinoma cells, Cancer Lett., 2010, 297, 144–154 CrossRef CAS PubMed.
- S. P. Kim, M. Y. Kang, J. H. Kim, S. H. Nam and M. Friedman, Composition and mechanism of antitumor effects of Hericium erinaceus, mushroom extracts in tumor-bearing mice, J. Agric. Food Chem., 2011, 59, 9861–9869 CrossRef CAS PubMed.
- X. Y. Li, Z. Y. Wang, L. Wang, E. Walid and H. Zhang, In vitro antioxidant and anti-proliferation activities of polysaccharides from various extracts of different mushrooms, Int. J. Mol. Sci., 2012, 13, 5801–5817 CrossRef CAS PubMed.
- Z. H. Han, J. M. Ye and G. F. Wang, Evaluation of in vivo antioxidant activity of Hericium erinaceus polysaccharides, Int. J. Biol. Macromol., 2013, 52, 66–71 CrossRef CAS PubMed.
- Z. Zhang, G. Lv, H. Pan, A. Pandey, W. He and L. Fan, Antioxidant and hepatoprotective potential of endo-polysaccharides from Hericium erinaceus grown on tofu whey, Int. J. Biol. Macromol., 2012, 51, 1140–1146 CrossRef CAS PubMed.
- M. Wang, Y. Gao, D. Xu and Q. Gao, A polysaccharide from cultured mycelium of Hericium erinaceus, and its anti-chronic atrophic gastritis activity, Int. J. Biol. Macromol., 2015, 81, 656–661 CrossRef CAS PubMed.
- J. Trowsdale and P. Parham, Mini-review: Defense strategies and immunity-related genes, Eur. J. Immunol., 2004, 34, 7–17 CrossRef CAS PubMed.
- S. Z. Xie, R. Hao, X. Q. Zha, L. H. Pan, J. Liu and J. P. Luo, Polysaccharide of Dendrobium huoshanense activates macrophages via toll-like receptor 4-mediated signaling pathways, Carbohydr. Polym., 2016, 146, 292–300 CrossRef CAS PubMed.
- T. H. Mogensen, Pathogen recognition and inflammatory signaling in innate immune defenses, Clin. Microbiol. Rev., 2009, 22, 240–273 CrossRef CAS PubMed.
- H. Rabb, The T cell as a bridge between innate and adaptive immune systems: implications for the kidney, Kidney Int., 2002, 61, 1935–1946 CrossRef CAS PubMed.
- M. M. Zhang, G. Wang, F. R. Lai and H. Wu, Structural characterization and immunomodulatory activity of a novel polysaccharide from Lepidium meyenii, J. Agric. Food Chem., 2016, 64, 1921–1931 CrossRef CAS PubMed.
- W. Z. Liao, Z. Luo, D. Liu, Z. X. Ning, J. G. Yang and J. Y. Ren, Structure characterization of a novel polysaccharide from Dictyophora indusiata and its macrophage immunomodulatory activities, J. Agric. Food Chem., 2015, 63, 535–544 CrossRef CAS PubMed.
- F. F. Wu, C. H. Zhou, D. D. Zhou, S. Y. Ou and H. H. Huang, Structural characterization of a novel polysaccharide fraction from Hericium erinaceus and its signaling pathways involved in macrophage immunomodulatory activity, J. Funct. Foods, 2017, 37, 574–585 CrossRef CAS.
- K. L. Cheong, L. Z. Meng, X. Q. Chen, L. Y. Wang, D. T. Wu, J. Zhao and S. P. Li, Structural elucidation, chain conformation and immuno-modulatory activity of glucogalactomannan from cultured Cordyceps sinensis, fungus UM01, J. Funct. Foods, 2016, 25, 174–185 CrossRef CAS.
- Q. Yu, S. P. Nie, J. Q. Wang, D. F. Huang, W. J. Li and M. Y. Xie, Signaling pathway involved in the immunomodulatory effect of Ganoderma atrum, polysaccharide in spleen lymphocytes, J. Agric. Food Chem., 2015, 63, 2734–2740 CrossRef CAS PubMed.
- J. X. Wang, X. Tong, P. B. Li, H. Cao and W. W. Su, Immuno-enhancement effects of Shenqi Fuzheng Injection on cyclophosphamide-induced immunosuppression in Balb/c mice, J. Ethnopharmacol., 2012, 139, 788–795 CrossRef PubMed.
- T. M. Filisetti-Cozzi and N. C. Carpita, Measurement of uronic acids without interference from neutral sugars, Anal. Biochem., 1991, 197, 157 CrossRef CAS PubMed.
- Z. Lin, W. Liao and J. Ren, Physicochemical characterization of a polysaccharide fraction from Platycladus orientalis, (L.) franco and its macrophage immunomodulatory and anti-hepatitis B virus activities, J. Agric. Food Chem., 2016, 64, 5813–5823 CrossRef CAS PubMed.
- R. Dong, X. Wang, H. Wang, Z. Liu, J. Liu and J. E. Saavedra, Effects of JS-K, a novel anti-cancer nitric oxide prodrug, on gene expression in human hepatoma Hep3B cells, Biomed. Pharmacother., 2017, 88, 367–373 CrossRef CAS PubMed.
- J. Ren, Y. Zheng, Z. Lin, X. Han and W. Liao, Macroporous resin purification and characterization of flavonoids from Platycladus orientalis (L.) Franco and their effects on macrophage inflammatory response, Food Funct., 2017, 8, 86–95 CAS.
- M. Wang, X. B. Yang, J. W. Zhao, C. J. Lu and W. Zhu, Structural characterization and macrophage immunomodulatory activity of a novel polysaccharide from Smilax glabra Roxb, Carbohydr. Polym., 2016, 156, 390–402 CrossRef PubMed.
- J. S. Lee, D. S. Kwon, K. R. Lee, J. M. Park, S. J. Ha and E. K. Hong, Mechanism of macrophage activation induced by polysaccharide from Cordyceps militaris culture broth, Carbohydr. Polym., 2015, 120, 29–37 CrossRef CAS PubMed.
- Z. J. Wang, D. H. Luo and Z. Y. Liang, Structure of polysaccharides from the fruiting body of Hericium erinaceus Pers, Carbohydr. Polym., 2004, 57, 241–247 CrossRef CAS.
- A. Q. Zhang, P. L. Sun, J. S. Zhang, C. H. Tang, J. M. Fan, X. M. Shi and Y. J. Pan, Structural investigation of a novel fucoglucogalactan isolated from the fruiting bodies of the fungus Hericium erinaceus, Food Chem., 2007, 104, 451–456 CrossRef CAS.
- Y. Zhang, M. Gu, K. P. Wang, Z. X. Chen, L. Q. Dai, J. Y. Liu and F. Zeng, Structure, chain conformation and antitumor activity of a novel polysaccharide from Lentinus edodes, Fitoterapia, 2010, 81, 1163–1170 CrossRef CAS PubMed.
- X. C. Liu, Z. Y. Zhu, Y. L. Tang, M. Wang, Z. Wang, A. J. Liu and Y. M. Zhang, Structural properties of polysaccharides from cultivated fruit bodies and mycelium of Cordyceps militaris, Carbohydr. Polym., 2016, 142, 63–72 CrossRef CAS PubMed.
- S. P. Nie, S. W. Cui, A. O. Phillips, M. Y. Xie, G. O. Phillips, S. Al-Assaf and X. L. Zhang, Elucidation of the structure of a bioactive hydrophilic polysaccharide from Cordyceps sinensis by methylation analysis and NMR spectroscopy, Carbohydr. Polym., 2011, 84, 894–899 CrossRef CAS.
- L. B. Ye, J. R. Li, J. S. Zhang and Y. J. Pan, NMR characterization for polysaccharide moiety of a glycopeptide, Fitoterapia, 2010, 81, 93–96 CrossRef CAS PubMed.
- Q. Guo, S. W. Cui, J. Kang, H. Ding, Q. Wang and C. Wang, Non-starch polysaccharides from American ginseng: physicochemical investigation and structural characterization, Food Hydrocolloids, 2015, 44, 320–327 CrossRef CAS.
- Y. Deng, L. X. Chen, B. X. Han, D. T. Wu, K. L. Cheong, N. F. Chen, J. Zhao and S. P. Li, Qualitative and quantitative analysis of specific polysaccharides in Dendrobium huoshanense, by using saccharide mapping and chromatographic methods, J. Pharm. Biomed. Anal., 2016, 129, 163–171 CrossRef CAS PubMed.
- Y. Zhang, T. Zhou, H. Wang, Z. Cui, F. Cheng and K. P. Wang, Structural characterization and in vitro antitumor activity of an acidic polysaccharide from Angelica sinensis (Oliv.) Diels, Carbohydr. Polym., 2016, 147, 401–408 CrossRef CAS PubMed.
- B. O. Petersen, I. C. Skovsted, B. S. Paulsen, A. R. Redondo and S. Meier, Structural determination of Streptococcus pneumoniae, repeat units in serotype 41A and 41F capsular polysaccharides
to probe gene functions in the corresponding capsular biosynthetic loci, Carbohydr. Res., 2014, 400, 26–32 CrossRef CAS PubMed.
- P. Hu, Z. Li, M. Chen, Z. Sun, Y. Ling, J. Jiang and C. Huang, Structural elucidation and protective role of a polysaccharide from Sargassum fusiforme on ameliorating learning and memory deficiencies in mice, Carbohydr. Polym., 2016, 139, 150–158 CrossRef CAS PubMed.
- K. Marszewska, M. Czerwicka, S. J. Forsythe, K. Ossowska, H. Dziadziuszko and Z. Kaczyński, Structural studies of O-polysaccharide isolated from Cronobacter sakazakii, Sequence Type 12 from a case of neonatal necrotizing enterocolitis, Carbohydr. Res., 2015, 407, 55–58 CrossRef CAS PubMed.
- A. S. Shashkov, W. Zhang, A. V. Perepelov, A. Weintraub, B. Liu, G. Widmalm and Y. A. Knirel, Structure of the O-polysaccharide of Escherichia coli O132, Carbohydr. Res., 2016, 427, 44–47 CrossRef CAS PubMed.
- J. Jiang, F. Kong, N. Li, D. Zhang, C. Yan and H. Lv, Purification, structural characterization and in vitro antioxidant activity of a novel polysaccharide from Boshuzhi, Carbohydr. Polym., 2016, 147, 365 CrossRef CAS PubMed.
- W. Cui, G. Mazza and C. Biliaderis, Chemical structure, molecular size distributions, and rheological properties of flaxseed gum, J. Agric. Food Chem., 1994, 42, 1891–1895 CrossRef CAS.
- W. Wang, Y. Zou, Q. Li, R. Mao, X. Shao, D. Jin, D. Zheng, T. Zhao, H. Zhu and L. Zhang, Immunomodulatory effects of a polysaccharide purified from Lepidium meyenii Walp. on macrophages, Process Biochem., 2016, 51, 542–553 CrossRef CAS.
- L. A. O'neill and D. G. Hardie, Metabolism of inflammation limited by AMPK and pseudo-starvation, Nature, 2013, 493, 346–355 CrossRef PubMed.
- S. Gordon, Phagocytosis: an immunobiologic process, Immunity, 2016, 44, 463–475 CrossRef CAS PubMed.
- W. J. Wu, M. M. Zhang, Y. Ren, X. Cai, Z. Yin, X. Zhang, T. Min and H. Wu, Characterization and immunomodulatory activity of a novel peptide, ECFSTA, from Wheat Germ Globulin, J. Agric. Food Chem., 2017, 65, 5561–5569 CrossRef CAS PubMed.
- X. F. Xu, H. D. Yan and X. W. Zhang, Structure and immuno-stimulating activities of a new heteropolysaccharide from Lentinula edodes, J. Agric. Food Chem., 2012, 60, 11560–11566 CrossRef CAS PubMed.
- M. M. Zhang, W. J. Wu, R. Yao, X. F. Li, Y. Q. Tang, M. Tian, F. R. Lai and H. Wu, Structural characterization of a novel polysaccharide from Lepidium meyenii (maca) and analysis of its regulatory function in macrophage polarization in vitro, J. Agric. Food Chem., 2017, 65, 1146–1157 CrossRef CAS PubMed.
- A. Mantovani, A. Sica, S. Sozzani, P. Allavena, A. Vecchi and M. Locati, The chemokine system in diverse forms of macrophage activation and polarization, Trends Immunol., 2004, 25, 677–686 CrossRef CAS PubMed.
- S. Fuu, C. Pojung, H. Kuangyang, C. Kahlock, W. T. Huang, T. Chiaoyin, Y. F. Chen, H. C. Cheng and H. H. Chang, Purification, cloning, and functional characterization of a novel immunomodulatory protein from Antrodia camphorata, (bitter mushroom) that exhibits TLR2-dependent NF-κB activation and M1 polarization within murine macrophages, J. Agric. Food Chem., 2009, 57, 4130–4141 CrossRef PubMed.
- B. N. Gantner, R. M. Simmons, S. J. Canavera, S. Akira and D. M. Underhill, Collaborative induction of inflammatory responses by dectin-1 and Toll-like receptor 2, J. Exp. Med., 2003, 197, 1107–1117 CrossRef CAS PubMed.
- I. A. Schepetkin and M. T. Quinn, Botanical polysaccharides: macrophage immunomodulation and therapeutic potential, Int. Immunopharmacol., 2006, 6, 317–333 CrossRef CAS PubMed.
- K. Ito, A. Hirao, F. Arai, K. Takubo, S. Matsuoka, K. Miyamoto, M. Ohmura, K. Naka, K. Hosokawa and Y. Ikeda, Reactive oxygen species act through p38 MAPK to limit the lifespan of hematopoietic stem cells, Nat. Med., 2006, 12, 446–451 CrossRef CAS PubMed.
- Y. H. Hong, H. B. Peng, V. La Fata and J. K. Liao, Hydrogen peroxide-mediated transcriptional induction of macrophage colony-stimulating factor by TGF-beta1, J. Immunol., 1997, 159, 2418–2423 CAS.
- N. Kaul, R. Gopalakrishna, U. Gundimeda, J. Choi and H. J. Forman, Role of protein kinase C in basal and hydrogen peroxide-stimulated NF-κB activation in the murine macrophage J774A. 1 cell line, Arch. Biochem. Biophys., 1998, 350, 79–86 CrossRef CAS PubMed.
- Q. Yu, S. P. Nie, J. Q. Wang, P. F. Yin, D. F. Huang, W. J. Li and M. Y. Xie, Toll-like receptor 4-mediated ROS signaling pathway involved in Ganoderma atrum polysaccharide-induced tumor necrosis factor-α secretion during macrophage activation, Food Chem. Toxicol., 2014, 66, 14–22 CrossRef CAS PubMed.
|
This journal is © The Royal Society of Chemistry 2018 |
Click here to see how this site uses Cookies. View our privacy policy here.