DOI:
10.1039/C7MT00272F
(Paper)
Metallomics, 2018,
10, 194-200
Oral trivalent bismuth ions decrease, and trivalent indium or ruthenium ions increase, intestinal tumor burden in ApcΔ14/+ mice†
Received
26th September 2017
, Accepted 18th December 2017
First published on 18th December 2017
Abstract
Immature forms of the peptide hormone gastrin have been implicated in the development of colorectal cancer (CRC). The biological activity of glycine-extended gastrin (Ggly) is dependent on the binding of Fe3+ ions in vitro and in vivo. The aim of the present study was to determine the effect of blocking Fe3+ ion binding to Ggly, using Bi3+, In3+ or Ru3+ ions, on the development of intestinal tumors in APCΔ14/+ mice. APCΔ14/+ mice were treated orally with Bi3+, In3+ or Ru3+ ions for up to 60 days, serum trace metals were analyzed by inductively coupled plasma mass spectrometry, and the incidence and size of intestinal tumors were assessed. Bi3+ treatment significantly decreased the number of tumors larger than 3 mm in male mice. In3+ or Ru3+ treatment significantly increased the tumor burden in all animals and In3+ increased the number of tumors larger than 3 mm or 5 mm in male mice alone. The fact that binding of In3+ or Ru3+ ions to Ggly was orders of magnitude stronger than the binding of Bi3+ ions implies that the inhibitory effect of Bi3+ ions is not a consequence of a reduction in Ggly activity. However, further testing of higher doses of Bi3+ ions for longer periods as an oral treatment for intestinal tumors is warranted.
Introduction
Gastrins are a collective term for mature and immature peptides derived from the gastrin gene. Amidated gastrin (Gamide), the fully processed gut hormone, activates the cholecystokinin 2 (CCK2) receptor to stimulate gastric acid secretion and maintain the gastric mucosa.1,2 Immature forms of gastrin (e.g., progastrin and glycine-extended gastrin (Ggly)) are also biologically active independently of the CCK2 receptor and act primarily in the colon to stimulate cell division,3–6 migration, and survival.1,4 Immature gastrins are found in colonic polyps and adenocarcinomas,7 and have been implicated in the development of colorectal cancer (CRC). Thus greater concentrations of immature gastrins are found in the circulation of CRC patients,8,9 and hypergastrinemic patients have a 4-fold risk of developing CRC.8,10,11 Although several candidates for the Ggly receptor have been identified (e.g. annexin II,12 F1-ATPase α-subunit13), small molecule inhibitors have not as yet been developed.
Most human sporadic CRCs contain an inactivating mutation in the APC (adenomatous polyposis coli) tumor suppressor gene.14 APC mutant mice provide a model of spontaneous intestinal cancers in vivo without the use of carcinogens.14,15 Koh et al. crossed APCmin−/+ mice that spontaneously develop intestinal tumors, primarily in the small intestine, with either mice overexpressing glycine-extended gastrin (MTI/Ggly) or gastrin knockout mice (GASKO).16 The data showed that mice prone to develop intestinal tumors had enhanced tumor size and numbers when Ggly was over-expressed and significantly reduced tumor incidence when all gastrins were absent. The development of APCΔ14/+ mice, with a deletion of exon 14 engineered into one allele of their Apc tumor suppressor gene, has provided a better model of familial adenomatous polyposis, as the proportion of spontaneous tumors developing in their large intestine is greater than with APCmin−/+ mice.17
Strong links between excess iron and CRC have also been reported.18,19 Study of APCmin/+ mice with either iron-deficient, normal or excess iron diets found that high iron drove intestinal tumorigenesis, as tumor number and size correlated with dietary iron status.20 Using GASKO mice and CCK2 receptor knockout mice (which have high circulating gastrin concentrations), Kovac et al. found that gastrins have a direct role in hematopoiesis under iron deficiency.21 GASKO mice on a low iron diet had impaired erythropoiesis and developed splenomegaly. Hypoxia studies indicated that gastrins may play a protective role under low oxygen conditions, possibly via catalysis of iron binding to transferrin.22,23
Work to elucidate the mechanism of action of gastrins revealed that ferric ion binding to glutamates 7 and 8/9 in the sequence QGPWLEEEEEAYGWMDF was essential for biological activity of Ggly, but not Gamide.24–26 Bioactivity of Ggly was diminished or destroyed by deleting these glutamates or by treating with the chelator desferrioxamine (DFO).25,27 Trivalent Bi3+ ions can displace ferric ions from the iron-binding sites on gastrins, with a concomitant reduction in the biological activity of Ggly in vitro.28,29In vivo, Ggly-induced stimulation of proliferation in the defunctioned rectal mucosa of rats could be abrogated with oral Bi3+ treatments, and oral Bi3+ also decreased proliferation rates in the colonic mucosa in both hGAS (over-expressing progastrin) and MTI/Ggly mice (over-expressing glycine-extended gastrin).29 In addition, DFO restored the increased colorectal mucosa proliferation rates in mice overexpressing progastrin (hGAS) or Ggly (MTI/Ggly) to the levels observed in wild-type control mice.30
Recently trivalent In3+ and Ru3+ ions have been shown to form stable, high affinity complexes with gastrins.26,31 Ru3+ and In3+ ions were both bound to the iron-binding site of gastrins orders of magnitude more tightly than ferric ions. Because the biological activity of Ggly was dependent on the presence of ferric ions, the possibility arose of using Bi3+, In3+ or Ru3+ ions as competitive inhibitors of the biological activity of Ggly. As inhibitors of the Ggly receptor are not yet available, the aim of the present study was to determine the effect of blocking ferric ion binding to Ggly using Bi3+, In3+ or Ru3+ ions on the incidence and size of intestinal tumors in APCΔ14/+ mice.
Experimental
Reagents and dosage
All consumables were obtained from Sigma Aldrich (Castle Hill, Australia), unless noted otherwise. Bismuth citrate, indium citrate and ruthenium citrate were all suspended in phosphate-buffered saline (ThermoFisher Scientific, Waltham, MA, USA), and the concentrations described below are based on the metal ion. The dose of Bi3+ used was derived from previous work in which 141 mg Bi3+ per kg per day was active, but not toxic, in mice treated daily for 4 weeks.29 This dose is 70-fold less concentrated than the single 50% lethal dose (LD50) of 10
000 mg per kg in mice. A single Bi3+ dose of 2000 mg per kg (1/5 of LD50), or Bi3+ doses of 1000 mg per kg per day (1/10 of its LD50) repeated daily for 28 days in rats, also showed no adverse effects.32,33 A similar study with oral In3+ in rats also demonstrated that a single dose of 2000 mg per kg (1/5 of its LD50 of 10
000 mg per kg in mice), or an In3+ dose of 1000 mg per kg per day (1/10 of its LD50) repeated daily for 28 days in rats, had no adverse effects.33,34 Based on this study, the same concentration was used for In3+ as for Bi3+. The same logic was used to derive the Ru3+ dose of 6.5 mg per kg per day based on a concentration 70-fold less than the reported LD50 of 463 mg per kg in mice.35
Mouse model
APCΔ14/+ mice (C57Bl/6 background) were kindly provided by Dr Julie Pannequin (National Centre for Scientific Research, Institute of Functional Genomics, Montpellier, France). These mice, which have had a heterozygous deletion of exon 14 engineered into one allele of their Apc tumor suppressor gene, provide a model of familial adenomatous polyposis (autosomal dominant disease), with spontaneous tumors developing in their small and large intestines.17,36
APCΔ14/+ mice (8–10 weeks old) were divided into four treatment groups and orally gavaged with 0.1–0.15 mL of PBS (16 female and 17 male mice), bismuth citrate (9 female and 8 male mice, 141 mg Bi3+ per kg per day), indium citrate (7 female and 8 male mice, 141 mg In3+ per kg per day) or ruthenium citrate (7 female and 8 male mice, 6.5 mg Ru3+ per kg per day) once a day, 3 times a week, up to 20 weeks of age. Animals were weighed and sickness scores taken daily. Mice were humanely sacrificed at the end of the experiment by isoflurane inhalation and blood was collected by cardiac puncture. Intestines were collected, inverted on wooden skewers, formalin fixed and prepared as Swiss Rolls for counting of intestinal tumors as described.37 All animal work was approved by the Austin Health Animal Research Ethics Committee (AEC13/4957 and AEC15/5307).
Hematological parameters
Full blood examination was performed as previously described22 by the Austin Pathology Laboratory (Heidelberg, VIC, Australia) using an Advia 120 automated hematological analyzer (Bayer, Tarrytown, NY, USA). Serum trace metals were analyzed by inductively coupled plasma mass spectrometry (ICPMS) (Biometals Facility, Florey Institute of Neuroscience and Mental Health, University of Melbourne). Serum from mice was diluted with 1/10 1% (v/v) nitric acid and measured with an Agilent 7700 series ICPMS instrument under routine multi-element operating conditions using a helium gas reaction cell. A certified standard solution containing 200 ppb of Yttrium (Y89) was used as an internal control.
Statistics
Survival statistics were performed using log-rank and cox-regression (SPSS version 22.0, IBM, Armonk, NY, USA). All other statistics were analyzed using either student's t-test or one-way ANOVA (SigmaStat, Jandel Scientific, San Rafael, CA, USA). A P value < 0.05 was considered significant.
Results
Treatment increased metal ion concentrations in the serum
Metal salts were given by oral gavage for up to 60 days at the following doses: Bi3+, 141 mg per kg per day; In3+, 141 mg per kg per day; Ru3+, 6.5 mg per kg per day. At the end of the experiment, concentrations of each metal ion in the serum were measured by ICPMS. All metals were absorbed as the serum concentrations increased significantly in the treated animals (Fig. 1). However, the ratio of Bi3+ absorbed to the amount given orally was low (all: 1/980; female: 1/681; male: 1/1,986). The ratios of In3+ and Ru3+ absorbed to the amounts given orally were closer to unity (In3+, all: 1/35; female: 1/92; male: 1/25 and Ru3+, all: 1/27; female: 1/64; male: 1/18).
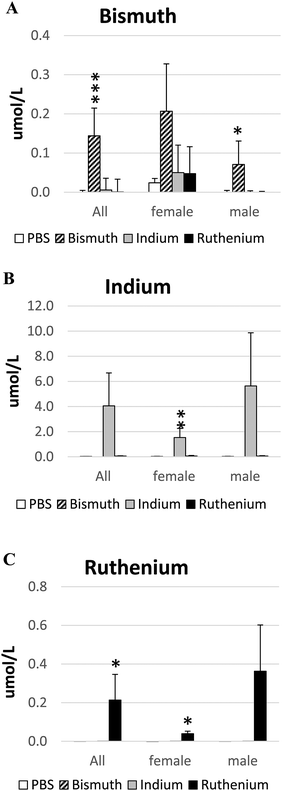 |
| Fig. 1 Serum metal ion analyses. The concentrations of Bi3+ (A), In3+ (B) or Ru3+ (C) in the serum from APCΔ14/+ mice treated with PBS (control), bismuth citrate, indium citrate or ruthenium citrate were analyzed by inductively coupled plasma mass spectrometry (ICPMS). *P < 0.05; **P < 0.01; ***P < 0.001. | |
Effect of metal ions on the health of APCΔ14/+ mice
APCΔ14/+ mice develop intestinal bleeding and anemia similar to APCmin/+ mice. However, APCΔ14/+ mice may show earlier signs of decreased health as they develop tumors in both their small and large intestines, while APCmin/+ mice typically develop tumors only in their small intestines.17 APCΔ14/+ mice in all treatment groups (PBS, Bi3+, In3+ or Ru3+) had increased sickness scores over time (Fig. S1, ESI†). Male mice appeared to be more sensitive with greater sickness scores (Fig. S1C, ESI†) and greater average weight loss (Fig. S2C, ESI†) than females. Overall, neither Bi3+ nor In3+ affected mouse survival (Fig. 2A); mice treated with Ru3+ died 2.0 times faster than PBS mice (P = 0.079) and the survival of Ru3+-treated animals was less than Bi3+- or In3+-treated animals.
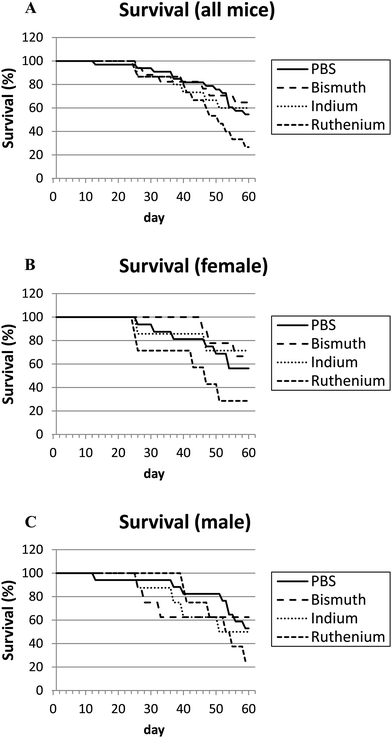 |
| Fig. 2 Survival plots. APCΔ14/+ mice were treated with PBS (control), bismuth citrate, indium citrate or ruthenium citrate over 60 days. Survival graphs are shown for all (A), female only (B) and male only (C) mice. The difference between PBS- and Ru3+-treated mice neared significance as cox-regression analysis indicated Ru3+-treated mice died 2.0 times faster than PBS-treated mice (P = 0.079). | |
APCΔ14/+ mice have hypoxemia
Anemia is a common outcome of the APCΔ14/+ mutation due to intestinal bleeding from large polyps. The PBS control and all three treatment groups had significantly decreased hemoglobin concentrations (139 ± 3), hematocrits (0.47 ± 0.01) and red cell counts (9.2 ± 0.2) compared to the values shown in the brackets for untreated C57Bl/6 mice of a similar age (our unpublished data) (Fig. 3A–C). Males, particularly those treated with Ru3+, appeared to be more affected. Serum iron concentrations were not significantly different to each other or to the value for untreated wild type animals (26.2 ± 1.5), but In3+ and Ru3+ appeared to increase or decrease iron levels respectively (Fig. 3E). Treatment with In3+ significantly increased WCC compared to the other groups (Fig. 3D), in agreement with previous findings.34,38 Platelet numbers were significantly reduced in Bi3+-treated APCΔ14/+ mice, to values closer to those observed in untreated wild-type mice (Fig. 3F).
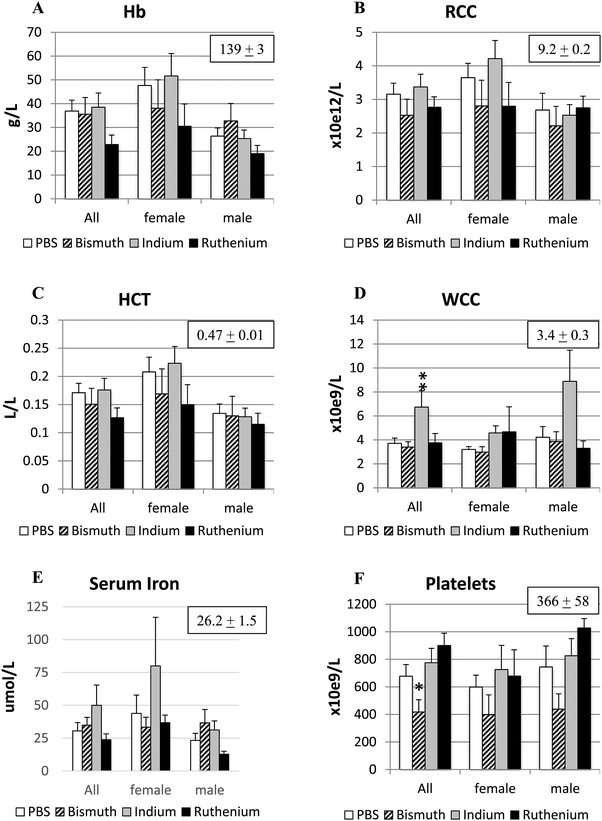 |
| Fig. 3 Hematological parameters. Blood samples from APCΔ14/+ mice treated with PBS (control), bismuth citrate, indium citrate or ruthenium citrate were analyzed for hemoglobin (A), red blood cell count (B), hematocrit (C), white blood cell count (D), and platelet count (F) by the Austin Pathology Laboratory (Heidelberg, VIC, Australia). Serum iron levels (E) were analyzed by ICPMS. Boxed numbers are the corresponding mean ± SEM values from untreated wild-type C57Bl/6 mice of similar ages. *P < 0.05; **P < 0.01. | |
Bi3+ treatment decreased, and In3+ or Ru3+ treatment increased, tumor burden in the small intestine
Intestinal tumor size and numbers were determined in the small and large intestines from APCΔ14/+ mice treated with PBS, Bi3+, In3+ or Ru3+. Bi3+ treatment significantly decreased the number of tumors larger than 3 mm in male mice (Fig. 4C). In3+ or Ru3+ treatment significantly increased the tumor burden in all animals and in male mice alone (Fig. 4A and C). No significant differences were observed in the colon (Fig. 5).
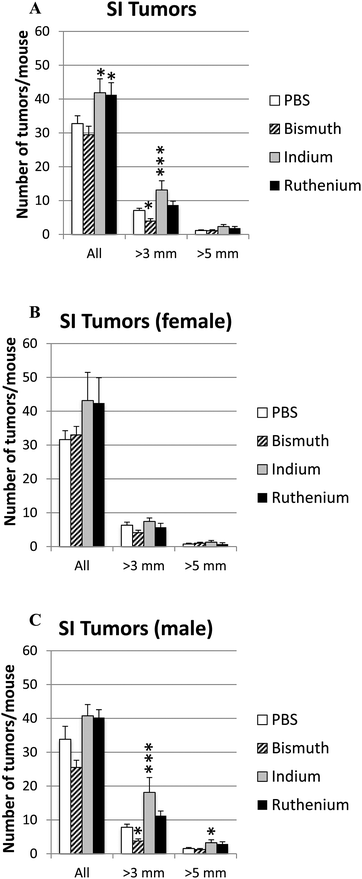 |
| Fig. 4 Tumor burden in small intestine. APCΔ14/+ mice were treated with PBS, Bi3+, In3+ or Ru3+, and the total number and number of tumors over 3 mm or 5 mm in the small intestine were counted for all mice (A), female only (B) and male only (C). Significance was determined compared to the PBS-treated mice. *P < 0.05; ***P < 0.001. | |
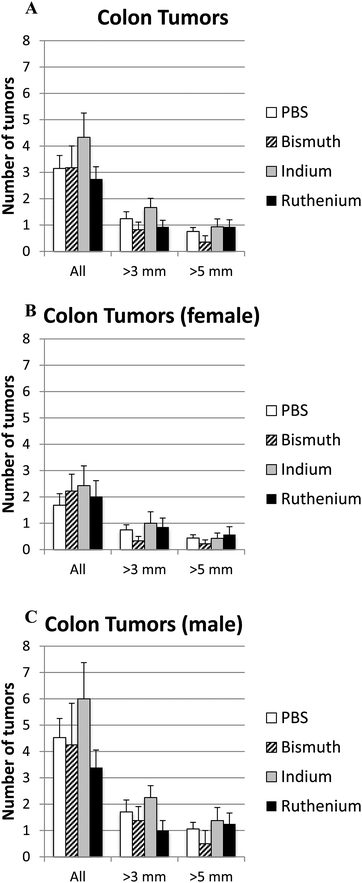 |
| Fig. 5 Tumor burden in colon. APCΔ14/+ mice were treated with PBS, Bi3+, In3+ or Ru3+, and the total number and number of tumors over 3 mm or 5 mm in the colon were counted for all mice (A), female only (B) and male only (C). No significance difference was detected. | |
Discussion
Previous work has revealed that APCmin−/+ mice, which are prone to develop intestinal tumors, had enhanced tumor size and numbers when Ggly was over-expressed and significantly reduced tumor incidence when all gastrins were absent.16 The observation that Bi3+ ions blocked the binding of Fe3+ ions to gastrins, and thereby decreased the bioactivity of Ggly in vivo and in vitro,28,29 suggested that Bi3+ treatment might also decrease tumor burden in APC mice. Further work revealed that the binding of In3+ or Ru3+ ions to gastrins was orders of magnitude stronger than the binding of Bi3+ ions.26 The effect of blocking ferric ion binding to Ggly using Bi3+, In3+ or Ru3+ ions on the incidence and size of intestinal tumors in APCΔ14/+ mice was therefore investigated.
Bi3+ treatment significantly decreased the number of small intestinal tumors larger than 3 mm in male mice (Fig. 4C). No reduction in tumor numbers was observed in the colon, or on tumors larger than 5 mm in either small intestine or colon, possibly because of the smaller numbers involved. The mechanism is likely to involve competition by Bi3+ for Fe3+ ion uptake and/or inhibition of intracellular processes involving iron. The effectiveness of Bi3+ treatment might possibly be improved by increasing the dosage or route of administration, as bismuth citrate was poorly absorbed and/or retained in the circulation (Fig. 1), in agreement with previous reports on its poor absorption.39,40 The observation that Bi3+ did not affect circulating iron concentrations or most blood parameters at the dosage used (Fig. 3) suggests that greater dosages might be tolerated, although the significant reduction in platelet counts following Bi3+ treatment suggests that a cautious approach would be appropriate.
In contrast to Bi3+, both In3+ and Ru3+ treatments significantly increased tumor numbers in the small intestine of APCΔ14/+ mice. Again no effect was observed on tumors in the colon, possibly because of the smaller numbers involved. The fact that binding of In3+ or Ru3+ ions to gastrins was orders of magnitude stronger than the binding of Bi3+ ions,26 therefore implies that the inhibitory effect of Bi3+ ions is not a consequence of a reduction in Ggly activity. The failure to observe an effect does not appear to be caused by poor absorption as the absorption ratios (μmol L−1 in the serum: amount given orally) for In3+ and Ru3+ were greater than for Bi3+. Furthermore both In3+ and Ru3+ treatments were effective in other respects. Thus In3+ treatment significantly increased white cell counts, in agreement with previous reports that In3+ can stimulate the immune system,34,38 and Ru3+ treatment decreased survival. The latter effect may be caused by displacement by Ru3+ of circulating iron from transferrin and hemoglobin,41,42 as ruthenium is in the same column of the periodic table as iron.
Conclusions
Bi3+ treatment significantly decreased the number of tumors larger than 3 mm in male APCΔ14/+ mice. Comparison of the effects of Bi3+, In3+ and Ru3+ treatment on tumor numbers suggests that the inhibitory effect of Bi3+ ions is unlikely to be gastrin-dependent. However, further testing of higher doses of Bi3+ ions for longer periods as an oral treatment for intestinal tumors is warranted. The use of In3+ or Ru3+ ions for preventative reduction of intestinal tumor burden appears inadvisable based on the present findings, and their use in other tumor treatments should be approached with caution, especially if the patient is anemic.
Conflicts of interest
There are no conflicts to declare.
Acknowledgements
We thank Jennifer Hunt for preparation of the metal ion solutions and Dannel Yeo for invaluable assistance with the survival statistics. This work was supported by the National Health and Medical Research Council of Australia [grant number 1020983 to GSB].
References
- A. Aly, A. Shulkes and G. S. Baldwin, Biochim. Biophys. Acta, 2004, 1704, 1–10 CAS.
- G. J. Dockray, A. Varro, R. Dimaline and T. Wang, The gastrins: their production and biological activities, Annu. Rev. Physiol., 2001, 63, 119–139 CrossRef CAS PubMed.
- F. Hollande, A. Imdahl, T. Mantamadiotis, G. D. Ciccotosto, A. Shulkes and G. S. Baldwin, Gastroenterology, 1997, 113, 1576–1588 CrossRef CAS.
- T. C. Wang, T. J. Koh, A. Varro, R. J. Cahill, C. A. Dangler, J. G. Fox and G. J. Dockray, J. Clin. Invest., 1996, 98, 1918–1929 CrossRef CAS PubMed.
- T. J. Koh, G. J. Dockray, A. Varro, R. J. Cahill, C. A. Dangler, J. G. Fox and T. C. Wang, J. Clin. Invest., 1999, 103, 1119–1126 CrossRef CAS PubMed.
- A. Ferrand and T. C. Wang, Cancer Lett., 2006, 238, 15–29 CrossRef CAS PubMed.
- C. Do, C. Bertrand, J. Palasse, M. B. Delisle, E. Cohen-Jonathan-Moyal and C. Seva, BMC Cancer, 2013, 13, 531 CrossRef PubMed.
- C. Do, C. Bertrand, J. Palasse, M. B. Delisle, A. Shulkes, E. Cohen-Jonathan-Moyal, A. Ferrand and C. Seva, Cancer Prev. Res., 2012, 5, 675–684 CrossRef PubMed.
- A. C. Paterson, F. A. Macrae, C. Pizzey, G. S. Baldwin and A. Shulkes, J. Gastroenterol. Hepatol., 2014, 29, 480–486 CrossRef CAS PubMed.
- C. M. Thorburn, G. D. Friedman, C. J. Dickinson, J. H. Vogelman, N. Orentreich and J. Parsonnet, Gastroenterology, 1998, 115, 275–280 CrossRef CAS.
- D. A. Westwood, O. Patel, C. Christophi, A. Shulkes and G. S. Baldwin, Int. J. Colorectal Dis., 2017, 32, 1061–1064 CrossRef PubMed.
- P. Singh, Cancer Lett., 2007, 252, 19–35 CrossRef CAS PubMed.
- A. Kowalski-Chauvel, S. Najib, I. G. Tikhonova, L. Huc, F. Lopez, L. O. Martinez, E. Cohen-Jonathan-Moyal, A. Ferrand and C. Seva, J. Biol. Chem., 2012, 287, 41458–41468 CrossRef CAS PubMed.
- L. N. Kwong and W. F. Dove, Adv. Exp. Med. Biol., 2009, 656, 85–106 CrossRef CAS PubMed.
- A. E. McCart, N. K. Vickaryous and A. Silver, Pathol., Res. Pract., 2008, 204, 479–490 CrossRef PubMed.
- T. J. Koh, C. J. Bulitta, J. V. Fleming, G. J. Dockray, A. Varro and T. C. Wang, J. Clin. Invest., 2000, 106, 533–539 CrossRef CAS PubMed.
- S. Colnot, M. Niwa-Kawakita, G. Hamard, C. Godard, S. Le Plenier, C. Houbron, B. Romagnolo, D. Berrebi, M. Giovannini and C. Perret, Lab. Invest., 2004, 84, 1619–1630 CrossRef CAS PubMed.
- H. Padmanabhan, M. J. Brookes and T. Iqbal, Nutr. Rev., 2015, 73, 308–317 CrossRef PubMed.
- A. C. Chua, B. Klopcic, I. C. Lawrance, J. K. Olynyk and D. Trinder, World J. Gastroenterol., 2010, 16, 663–672 CrossRef CAS PubMed.
- S. Radulescu, M. J. Brookes, P. Salgueiro, R. A. Ridgway, E. McGhee, K. Anderson, S. J. Ford, D. H. Stones, T. H. Iqbal, C. Tselepis and O. J. Sansom, Cell Rep., 2012, 2, 270–282 CrossRef CAS PubMed.
- S. Kovac, G. J. Anderson, W. S. Alexander, A. Shulkes and G. S. Baldwin, Endocrinology, 2011, 152, 3062–3073 CrossRef CAS PubMed.
- M. Laval, G. S. Baldwin, A. Shulkes and K. M. Marshall, Increased gastrin gene expression provides a physiological advantage to mice under hypoxic conditions, Am. J. Physiol.: Gastrointest. Liver Physiol., 2015, 308, G76–84 CrossRef CAS PubMed.
- S. C. Longano, J. Knesel, G. J. Howlett and G. S. Baldwin, Interaction of gastrin with transferrin: effects of ferric ions, Arch. Biochem. Biophys., 1988, 263, 410–417 CrossRef CAS PubMed.
- G. S. Baldwin, C. C. Curtain and W. H. Sawyer, Biochemistry, 2001, 40, 10741–10746 CrossRef CAS PubMed.
- J. Pannequin, K. J. Barnham, F. Hollande, A. Shulkes, R. S. Norton and G. S. Baldwin, J. Biol. Chem., 2002, 277, 48602–48609 CrossRef CAS PubMed.
- G. S. Baldwin, G. N. George and M. J. Pushie, PLoS One, 2015, 10, e0140126 Search PubMed.
- J. Pannequin, J. P. Tantiongco, S. Kovac, A. Shulkes and G. S. Baldwin, J. Endocrinol., 2004, 181, 315–325 CrossRef CAS PubMed.
- J. Pannequin, S. Kovac, J. P. Tantiongco, R. S. Norton, A. Shulkes, K. J. Barnham and G. S. Baldwin, J. Biol. Chem., 2004, 279, 2453–2460 CrossRef CAS PubMed.
- S. Kovac, S. W. Loh, S. Lachal, A. Shulkes and G. S. Baldwin, Biochem. Pharmacol., 2012, 83, 524–530 CrossRef CAS PubMed.
- A. Ferrand, S. Lachal, G. Bramante, S. Kovac, A. Shulkes and G. S. Baldwin, Am. J. Physiol.: Gastrointest. Liver Physiol., 2010, 299, G220–227 CrossRef CAS PubMed.
- M. Laval, K. M. Marshall, J. Sachinidis, A. Scott, M. Eutick and G. S. Baldwin, J. Biol. Inorg. Chem., 2017, 22, 999–1006 CrossRef CAS PubMed.
- Y. Sano, H. Satoh, M. Chiba, M. Okamoto, K. Serizawa, H. Nakashima and K. Omae, J. Occup. Health, 2005, 47, 293–298 CrossRef CAS PubMed.
- F. P. Castronovo, Jr. and H. N. Wagner, Jr., J. Nucl. Med., 1973, 14, 677–682 Search PubMed.
- K. Asakura, H. Satoh, M. Chiba, M. Okamoto, K. Serizawa, M. Nakano and K. Omae, J. Occup. Health, 2008, 50, 471–479 CrossRef CAS PubMed.
- European Medicines Agency, Guidelines on the specification limits for residues of metal catalysts, 2007, http://www.ema.europa.eu/docs/en_GB/document_library/Scientific_guideline/2009/09/WC500003586.pdf.
- N. Huynh, K. Wang, M. Yim, C. J. Dumesny, M. S. Sandrin, G. S. Baldwin, M. Nikfarjam and H. He, BMC Cancer, 2017, 17, 431 CrossRef PubMed.
- K. H. Liu, N. Huynh, O. Patel, A. Shulkes, G. Baldwin and H. He, Cancer Lett., 2013, 340, 22–29 CrossRef CAS PubMed.
- M. E. Blazka, D. Dixon, E. Haskins and G. J. Rosenthal, Fundam. Appl. Toxicol., 1994, 22, 231–239 CrossRef CAS PubMed.
- A. J. Wagstaff, P. Benfield and J. P. Monk, Drugs, 1988, 36, 132–157 CrossRef CAS PubMed.
- S. L. Gorbach, Gastroenterology, 1990, 99, 863–875 CrossRef CAS.
- F. Kratz, M. Hartmann, B. Keppler and L. Messori, J. Biol. Chem., 1994, 269, 2581–2588 CAS.
- D. R. Frasca, L. E. Gehrig and M. J. Clarke, J. Inorg. Biochem., 2001, 83, 139–149 CrossRef CAS PubMed.
Footnote |
† Electronic supplementary information (ESI) available. See DOI: 10.1039/c7mt00272f |
|
This journal is © The Royal Society of Chemistry 2018 |