DOI:
10.1039/C8QM00120K
(Research Article)
Mater. Chem. Front., 2018,
2, 1225-1237
Valeriana wallichii root extract as a green & sustainable corrosion inhibitor for mild steel in acidic environments: experimental and theoretical study†
Received
21st March 2018
, Accepted 2nd May 2018
First published on 3rd May 2018
Abstract
Corrosion inhibition analysis and the adsorption behaviour of Valeriana wallichii root extract for mild steel in 0.5 M H2SO4 solution has been investigated utilizing weight loss, electrochemical impendence spectroscopy (EIS), potentiodynamic polarization, SEM, AFM UV-visible spectroscopy, FT-IR, and quantum chemical calculations. Both the electrochemical and weight loss estimations indicated that the root extract of Valeriana wallichii shows excellent inhibition performance of up to 93.47% for mild steel at 500 mg L−1 concentration in 0.5 M H2SO4 solution at 298 K. Naphthoic acid, iridoid and an iridoid analogue are the major phytochemical constituents in the extract of Valeriana wallichii, which possess oxygen atoms (lone pair electrons) and π electrons that facilitate adsorption onto the metal surface. The adsorption of this extract obeys the Langmuir adsorption isotherm. Polarization investigations confirmed that the root extract of Valeriana wallichii acts as a mixed type of inhibitor. The surface studies confirm corrosion retardation in the presence of the extract due to the formation of a protective layer on the surface of mild steel.
1. Introduction
The most widely used alloy in industrial processes, fabrication and construction is mild steel because of its excellent mechanical competency, its easy availability, and most importantly, its low production cost.1 Acid cleaning processes on steel surfaces lead to corrosion problems. Corrosion can be controlled by using inhibitors.2 However, most of the synthetic inhibitors are expensive, toxic and hazardous to the environment.3,4 This has prompted researchers to find some non-toxic, eco-friendly, biodegradable, cheap and effective green inhibitors.5 Considering the above facts, plant extracts, which consist of various organic compounds with heteroatoms, like nitrogen and oxygen, and conjugated bonds, are a good option.6–8 The organic molecules used as the inhibitor are adsorbed on the metal surface through these heteroatoms and their conjugated system. This adsorption of the inhibitor molecules can either be physical, by electrostatic interaction (physisorption), or chemical, through covalent bond formation (chemisorption).9,10 The importance of the mechanism of corrosion inhibition, surface analysis and its relation to the electronic and anatomical properties of the inhibitor molecule has also attracted attention through quantum chemical calculations.10–16 Many researchers have already reported different plants as green corrosion inhibitors for acidic media with good inhibition efficiency; for example: Salvia officinalis shows 96% inhibition efficiency at 2500 mg L−1,17Osmanthus fragans shows 94% inhibition efficiency at 340 mg L−1,18Musa paradisiaca shows 90% inhibition efficiency at 300 mg L−1,19Mangrove tannins shows 89% inhibition efficiency at 6000 mg L−1,20Jasminum nudiflorum shows 92% inhibition efficiency at 1000 mg L−1,21Lawsonia inermis shows 92% inhibition efficiency at 1200 mg L−1,22Dendrocalamus brandisii shows 90% inhibition efficiency at 1000 mg L−1,23 aqueous coffee grounds shows 83% inhibition efficiency at 400 mg L−1,24Phyllanthus amarus shows 81% inhibition efficiency at 4000 mg L−1,25 black radish shows 92% inhibition efficiency at 1000 mg L−1,26Ginkgo shows 80% inhibition efficiency at 100 mg L−1,27Kola nitida shows 78% inhibition efficiency at 1200 mg L−1,28 radish roots show 79% inhibition efficiency at 100
000 mg L−1,29Murraya koenigii shows 96% inhibition efficiency at 600 mg L−1,30 bamboo leaf shows 89% inhibition efficiency at 200 mg L−131 and Butea monosperma shows 98% inhibition efficiency at 500 mg L−1.32
Valeriana wallichii is a rhizome herb. It belongs to the Valerianaceae family and is also known as Jatamansi, Hardwickii and Tagar. It is commonly found all throughout India, Pakistan, Nepal and China. The roots of Valeriana wallichii are very easily available in the market. Valeriana wallichii contains naphthoic acids, iridoid and an iridoid analogue as the major phytochemical compounds.33,34 The presence of various flavonoids in Valeriana wallichii root extract means that it shows an outstanding sleep-wake profile, and hence it is used for the treatment of primary insomnia.35,36 The presence of various flavonoids, which are very rich in heteroatoms and aromatic rings, means that it also shows anti-corrosive properties. An image of Valeriana wallichii roots and their major phytochemical constituents is shown in Fig. 1.
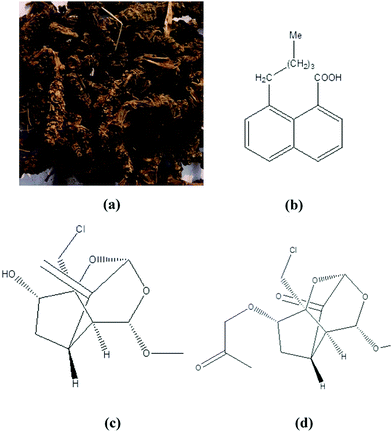 |
| Fig. 1 (a) Picture of Valeriana wallichii roots and their major chemical constituents: (b) naphthoic acid, (c) iridoid and (d) an iridoid analogue. | |
The present study is aimed at acquiring an extract from the Valeriana wallichii roots and investigating it as a corrosion inhibitor for mild steel in 0.5 M H2SO4 by utilizing methods like gravimetric measurements, electrochemical impedance spectroscopy, and potentiodynamic polarization measurement. In addition, the effect of structural parameters of the inhibitors on the inhibition efficiency, their adsorption mechanism on the metal surface and the correlation between the experimental data and the quantum chemical parameters have been studied.
2. Experimental
2.1. Preparation of the working electrode
The mild steel rod (1 cm diameter) used in this study has the nominal (wt%) composition presented in Table 1. The mild steel was incised and sectioned with a Clarke power hacksaw and ESM 700 excel shaping machine to give mild steel specimens with an average length of 1 cm to use as the test specimens for gravimetric measurements and for electrochemical studies. The same steel specimens were soldered to coat Cu-wires for electrical connections and after that mounted over epoxy resin with a laid open zone of 1 cm2. Before any measurements, different grades of emery paper, 100, 320, 600, 800, 1000 and 1200, were used to abrade the surface of the working electrode mechanically. After that, the mild steel specimens were rinsed with distilled water and acetone, and finally dried in warm air flow.
Table 1 Nominal composition (wt%) of mild steel
Element symbol |
C |
Mn |
Si |
P |
Cu |
Ni |
Cr |
Fe |
% Composition |
0.08 |
0.43 |
0.39 |
0.12 |
0.43 |
0.27 |
0.45 |
97.60 |
2.2. Preparation of the inhibitor and electrolytes
The raw product of Valeriana wallichii roots was purchased from a market and was validated by Dr Arbeen Ahmad Bhatt under accession number HERB/BOT/LPU-480 in the Department of Biotechnology, Lovely Professional University, Punjab, India. First, the roots of Valeriana wallichii were cleaned with distilled water to remove any remains of mud, and then the roots were dried for 48–72 h in a thermostat at 60 °C, ground and converted to powder form. Then, 100 g of powdered Valeriana wallichii was refluxed with ethanol at 75 °C for 24 h. The refluxed products were filtered, and the pH of the aqueous solution was found to be 9. The filtered solution was evaporated to 100 ml of dark brown residue and then dried in a vacuum drying oven at 60 °C for 48 h. At the point of dark brown colour, the deposit (around 5 g of gummy material) was procured after complete drying and saved in a vacuum desiccator. Sulphuric acid was diluted to 0.5 M H2SO4 as the corrosive medium. The test solutions with different concentrations of Valeriana wallichii root extract (100, 200, 300, 400 and 500 mg L−1) were obtained by diluting the extract with 0.5 M H2SO4. The corrosive solution (0.5 M H2SO4) was prepared from reagent grade, 98% H2SO4 (Sigma-Aldrich) and distilled water. The solutions of the inhibitor in 0.5 M H2SO4 were prepared with various concentrations (100–500 mg L−1). The greatest solvency of the Valeriana wallichii extracts in 0.5 M H2SO4 was observed up to 500 mg L−1.
2.3. Gravimetric measurements
Weight loss estimations of the mild steel specimens were performed as described in ASTM G 31-72.37 Gravimetric measurements (weight loss) were done for 24 h at 298 K, 308 K and 318 K. The blank acid 0.5 M H2SO4 solutions that contained 100, 200, 300, 400 and 500 mg L−1Valeriana wallichii root extract were prepared, and then pre-weighed metal specimens were immersed in the test solutions. Following a drenching period, the metal specimens were removed, deliberately rinsed with acetone, dried under nitrogen flow and weighed using a Shimadzu BL-220H/D455006313 electronic balance. The temperature of the experiments was kept constant by keeping the beakers in a PPI Fini X48 water circulated thermostat (±0.5 K exactness). In this study, the average values of three replicates are reported.
2.4. Electrochemical measurements
Electrochemical studies were conducted using a computer-controlled CH instrument electrochemical workstation (Model: CHI-760) in a corrosion cell kit with three electrode configurations. Platinum was used as a counter electrode, mild steel was used as the working electrode and a saturated calomel electrode (SCE) was used as a reference electrode. The temperature remained constant (298 K) for all the experiments in 0.5 M H2SO4. The inhibitor at a concentration of 100, 200, 300, 400 or 500 mg L−1 was added to the corrosive medium. The mild steel specimens were inserted into epoxy resin leaving a working zone of 1 cm2. The electrode potential was measured against the SCE and the polarization curves were recorded at an examination rate of 1 mV s−1 with various potentials from the detected open circuit potential (OCP), following 60 minutes of immersion. The cathodic and anodic curves of the linear Tafel segments were extrapolated to the corrosion potential to achieve the corrosion current densities. Electrochemical impedance spectroscopy (EIS) estimations were carried out in a frequency range of 100 kHz to 0.01 Hz with a single amplitude perturbation of 5 mV at OCP with a scan rate of 1 mV s−1. Potentiodynamic polarization curves were recorded with a scan rate of 1 mV s−1 starting at −250 mV with respect to the OCP and continuing up to +250 mV vs. SCE. All the experiments were performed in quiescent conditions, while the temperature was maintained constant (298 K) using a water bath. Reliable values were achieved by repeating all electrochemical measurements three times. By applying both techniques, the inhibition efficiency was determined.
2.5. UV-visible spectroscopy
With the help of a Shimadzu UV-1800 UV-Visible absorption spectrophotometer, the UV spectra of Valeriana wallichii in 0.5 M H2SO4 were recorded. The spectra were observed in two different situations, i.e. the acidic solution of the inhibitor in which the mild steel specimens were not immersed and the solution in which the steel specimens were immersed for 24 h at 298 K. Both these spectra were compared to explain the mechanism of inhibition.
2.6. FT-IR and 1H NMR spectroscopy
For a better understanding of the inhibition mechanism, the FTIR spectra of the Valeriana wallichii root extract were recorded. The Valeriana wallichii extract, blended with KBr powder, was transformed into a pellet for FTIR characterization in a Shimadzu FTIR 8400S spectrophotometer at a wavenumber of 500–4000 cm−1. 1H NMR (CDCl3) spectra of the inhibitor were recorded on a Bruker Advance II 400 MHz system for identification of the major phytochemical constituents present in the Valeriana wallichii extract.
2.7. Quantum chemical studies
On examining the impact of the molecular structure and electronic properties of the inhibitor molecules on the inhibition efficiency, quantum chemical calculations were carried out utilizing density functional theory (DFT) adopting the B3LYP function with the 6-31G+(d,p) basis set for all molecules, using the Hyperchem 8.0 project programming bundle. Key parameters, for example, the energy of the LUMO (ELUMO) and the HOMO (EHOMO), the energy gap (ΔE) between the LUMO and the HOMO, the dipole moment (μ), the electronegativity (χ), the softness (σ) and the hardness (η) of the molecules, the energy distinction between electron exchange of the molecule and back-donation starting with the atom (ΔE Back Donation), the chemical potential (π) and the number of transferred electrons (ΔN) were obtained from the optimized structures in the gas phase. It should be noted that doing the theoretical calculations in the gas phase is a useful method because the results obtained in the gas phase show no significant variation from those obtained in the aqueous phase, while at the same time the time required for the calculation can be significantly decreased.18
2.8. Surface morphology studies (SEM & AFM analysis)
The morphology of the mild steel surface was observed under a scanning electron microscope (Model: LEO-435VP) and then an atomic force microscope (Model: NT-MDT-INTEGRA) instrument after immersing the pre-treated mild steel specimens in 0.5 M H2SO4 solution without and with 500 mg L−1Valeriana wallichii root extract for 24 hours at 298 K, rinsing the surface of the mild steel specimen with double-distilled water and acetone, and then drying. This gives important information about the morphology of the surface of the mild steel.
3. Results and discussion
3.1. Weight loss measurements
The weight loss method, being the simplest technique, is considered as the “gold standard” of corrosion testing. However, since the mass can essentially only be measured to an accuracy of 0.1 mg at best, the reliability of the weight loss estimations is limited because of their variability. As a result, these estimations were performed three times at 298 K, 308 K and 318 K with various inhibitor concentrations (100, 200, 300, 400 and 500 mg L−1) for 24 h, so that they can provide an average rate.2
The corrosion rate was determined in the absence and presence of inhibitor using the following formula:
|  | (1) |
where
W is the weight loss of the mild steel strip (g),
CRW is the corrosion rate (mm year
−1),
t is the immersion time (h),
K is a constant equal to 8.76 × 10
4,
ρ is the density in g cm
−3, which is 7.86 g cm
−3 for mild steel according to ASTM G 31-72,
37 and
A is the surface area (cm
2) of mild steel, which is calculated using
eqn (2).
| 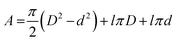 | (2) |
The inhibition efficiency (IE%) and surface coverage (
θ) values were calculated with the help of the following formulae:
| 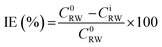 | (3) |
|  | (4) |
where
C0RW and
CiRW are the corrosion rate of mild steel in the absence and presence of inhibitor, respectively.
θ is the surface coverage value. In
eqn (2),
D and
l are the diameter and the thickness of the specimen, respectively, while
d is the diameter of the hole for holding.
9
The weight loss measurement values were used to plot the Langmuir adsorption isotherm and to calculate the thermodynamic parameters. The results are presented in Table 2. The inhibition efficiency increases with an increase in inhibitor concentration. This phenomenon is caused by the adsorption of active molecules of the Valeriana wallichii extract on the mild steel surface. The highest value of inhibition efficiency is obtained at 500 mg L−1 inhibitor concentration at 298 K.
Table 2 Corrosion parameters for mild steel in 0.5 M H2SO4 without and with various concentrations of Valeriana wallichii extract for 24 h at different temperatures
Temperature (K) |
Inhibitor concentration (mg L−1) |
C
R (mm year−1) |
Efficiency (IE%) |
θ
|
298 |
0 |
11.33 |
— |
— |
100 |
2.48 |
78.09 |
0.7809 |
200 |
2.14 |
81.10 |
0.8110 |
300 |
1.95 |
82.77 |
0.8277 |
400 |
1.16 |
89.68 |
0.8968 |
500 |
0.96 |
91.45 |
0.9145 |
|
308 |
0 |
15.29 |
— |
— |
100 |
3.82 |
74.96 |
0.7496 |
200 |
3.60 |
76.45 |
0.7645 |
300 |
3.08 |
79.80 |
0.7980 |
400 |
2.78 |
81.78 |
0.8178 |
500 |
2.44 |
84.01 |
0.8401 |
|
318 |
0 |
19.14 |
— |
— |
100 |
7.14 |
62.67 |
0.6267 |
200 |
5.89 |
69.20 |
0.6920 |
300 |
5.40 |
71.78 |
0.7178 |
400 |
4.98 |
73.96 |
0.7396 |
500 |
4.58 |
76.03 |
0.7603 |
3.2. UV-visible spectroscopy analysis
The UV spectra of the Valeriana wallichii extract with 0.5 M H2SO4 prior to and after immersion of the mild steel specimens in the solution were compared and are shown in Fig. 2. The spectrum of the Valeriana wallichii extract shows adsorption peaks at 278 nm and 321 nm. These peaks can be assigned as π–π* and n–π* transitions, respectively. From the absorption spectra, the absorbance of the solution in which the steel specimen was not immersed is higher in contrast with the solution into which the mild steel specimen was immersed for a period of 24 h. Shifting of the value of the absorption maximum (λmax) or a change in the value of the absorbance point demonstrates the creation of a complex between Fe2+ particles and inhibitor molecules. In conclusion, the results clearly indicate that when the mild steel specimen was immersed in the acidic solution of Valeriana wallichii extract, a few molecules from the solution are adsorbed on the metal surface.38
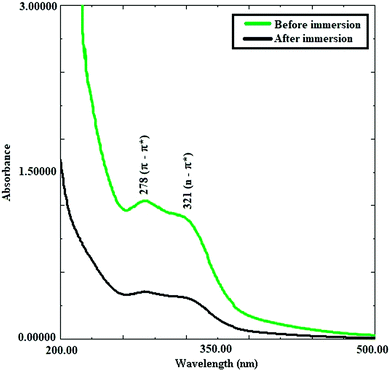 |
| Fig. 2 UV spectra of the Valeriana wallichii extract after and before immersion of mild steel strips for 24 h at 298 K. | |
3.3. FT-IR and 1H NMR studies
FTIR analysis has been performed to obtain some understanding of the possible interactions between the adsorbed molecules and the mild steel surface in an acidic environment. Generally, FTIR provides us with a basic idea of the functional groups present in an inhibitor molecule. Fig. 3 shows the FTIR spectrum of the root extract of Valeriana wallichii, which was investigated to evaluate the main functional groups. It was observed that the peak at 3312.37 cm−1 is due to O–H stretching of alcohols. The additional peak at 2943.34 cm−1 is due to C–H stretching and the peak at 1130.23 cm−1 indicates C–O stretching. In addition, the major phytochemical constituents of the Valeriana wallichii extract were confirmed by 1H NMR (Fig. S1, ESI†). 1H NMR (CDCl3, 400 MHz); δ = 6–7 ppm (H aromatic rings), δ = 1.27 (–CH3), δ = 5.40 (–OH phenolic), δ = 7.4 (–COOH). The presence of almost all the characteristic peaks as previously reported verifies the presence of phenolic compounds in the Valeriana wallichii root extract.33,34 The FT-IR and 1H NMR spectra of the Valeriana wallichii extract show that the anticorrosive properties of the Valeriana wallichii extract are due to the presence of oxygen atoms and aromatic rings.
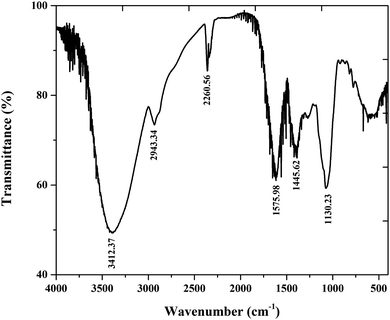 |
| Fig. 3 FT-IR spectra of the Valeriana wallichii extract. | |
3.4. Adsorption isotherm & thermodynamic parameters
The interaction between the inhibitor molecules and the mild steel surface is better demonstrated by adsorption isotherms. The inhibition effectiveness of an inhibitor depends on how well the inhibitor particles adsorb on the mild steel surface. The adsorption procedure includes reinstatement of water particles at the metal solution interface as stated by this equation:39 | Org(sol) + nH2O(ads) → Org(ads) + nH2O(sol) | (5) |
where Org(sol) is the inhibitor molecules in the solution, Org(ads) is the inhibitor molecules adsorbed on the metal surface, and n is the amount of water molecules replaced by inhibitor molecules. It is already known that the 1st stage of the corrosion restraint of metals is the adsorption of natural inhibitor particles between the metal and the solution. The surface coverage depends on the inhibition effectiveness, described as (θ = IE (%)/100), and the concentration of the inhibitor. The portion of the surface covered by the adsorbed molecules increases with increasing inhibitor concentration. To evaluate the thermodynamic and activation parameters of the corrosion process of mild steel in 0.5 M H2SO4, weight loss estimations were carried out at temperatures of 298 K, 308 K and 318 K for 24 h. The obtained information shows that the inhibition effectiveness decreases with increasing temperature. This is because of the dissolution process of the mild steel and the partial desorption of inhibitor molecules from the mild steel surface. The log of CR is a straight-line function with 1/T (Arrhenius eqn (6)). | 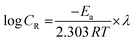 | (6) |
where Ea is the viable activation energy, R is the gas constant, and λ is the Arrhenius pre-exponential factor. The graph of log
CRvs. 1/T is a straight line as indicated in Fig. 4(b) with a slope of −Ea/2.303R.
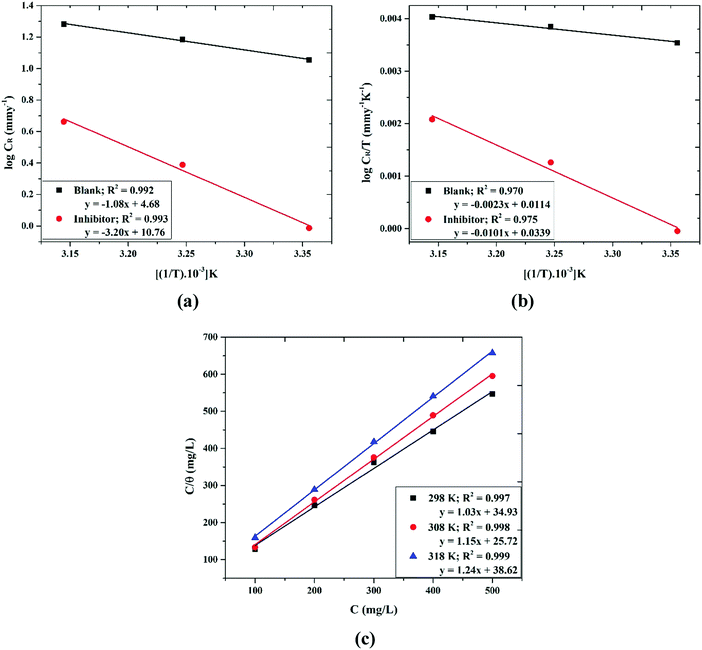 |
| Fig. 4 Adsorption isotherm plots for (a) log CRvs. 1/T, (b) log(CR/T) vs. 1/T and (c) the Langmuir adsorption isotherm (C/θ vs. C) based on weight loss measurements of the Valeriana wallichii extract on a mild steel surface in 0.5 M H2SO4 for 24 h. | |
The values of Ea recorded in Table 3(a) indicate that the thermodynamic activation function (Ea) of the corrosion of mild steel in 0.5 M H2SO4 in the presence of Valeriana wallichii extract is higher compared to that of the pure acid solution, demonstrating that the extract shows low IE (%) at raised temperature.
Table 3 (a) Standard thermodynamic parameters for the adsorption of the Valeriana wallichii extract on the mild steel surface in 0.5 M H2SO4. (b) Equilibrium adsorption parameters for the adsorption of the Valeriana wallichii extract on the mild steel surface in 0.5 M H2SO4 at 298 K
(a) |
Inhibitor concentration (mg L−1) |
E
a (kJ mol−1) |
ΔH0 (kJ mol−1) |
ΔS0 (J K−1 mol−1) |
Blank |
20.67 |
44.80 |
−218.66 |
Valeriana wallichii (500 mg L−1) |
61.37 |
193.38 |
56.35 |
(b) |
Temperature (K) |
K
ads (L g−1) |
ΔG0ads (kJ mol−1) |
298 |
28.62 |
−25.42 |
308 |
38.88 |
−27.06 |
318 |
25.89 |
−26.86 |
An elective plan for Arrhenius mathematical statement 7:
| 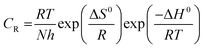 | (7) |
where
h is Plank's constant,
N is Avogadro's number, Δ
S0 is the entropy of activation and Δ
H0 is the enthalpy of activation. A chart of log(
CR/
T)
vs. 1/
T offered a straight line, as shown in
Fig. 4(c), with a slope of −Δ
H0/2.303
R and an intercept of log(
R/
Nh) + Δ
S0/2.303
R, from which Δ
S0 and Δ
H0 were ascertained and recorded in
Table 3(a).
The positive signs of enthalpy (ΔH0) in both systems reflect the endothermic route for the dissolution process, and the shift towards a positive value of entropy (ΔS0) indicates that disorder increases on going from the reactants to the activated complex. To further dissect the adsorption nature of the Valeriana wallichii extract on the mild steel surface, the Langmuir adsorption isotherm was utilized to fit the experimental data obtained from the weight loss estimations. The Langmuir adsorption isotherm is a plot of C/θ vs. C, and is shown in Fig. 4(a). The adsorption of inhibitor molecules on the mild steel surface according to the Langmuir adsorption isotherm is provided by the following equations:
|  | (8) |
|  | (9) |
where
θ is the surface coverage,
C is the inhibitor concentration and
Kads is the equilibrium adsorption constant.
40
The straight fitted lines with slopes of nearly 1 in Fig. 4(a) demonstrate that the absorption of the inhibitor molecules on the mild steel surface obeys the Langmuir adsorption isotherm and the inhibitor particles cover a monolayer on the surface of the mild steel.40 The higher value of Kads calculated from eqn (8) indicates the better adsorption of the inhibitor molecules on the surface of mild steel. Right away the Kads can be correlated with the standard free energy of adsorption by the following mathematical statement:
| ΔG0ads = −R × T ln(Cwater × Kads) | (10) |
where
R is the gas constant,
T is the supreme temperature, Δ
G0ads is the standard free energy of adsorption and
Cwater is the concentration of water in the solution. It should be noted that the unit of
Cwater depends on the
Kads. As per
Table 3(b), the unit of
Kads is L g
−1, which in turn shows that the unit of
Cwater is g L
−1 with a value of approximately 1 × 10
3. The related outcomes are obtained and listed in
Table 3(b). It has been shown that if the value of Δ
G0ads is −40 kJ mol
−1 or more negative, then a coordinate bond type metallic bond may be formed between the inhibitor molecules and the metal surface. This bond is formed due to chemisorption wherever charge sharing, or charge exchange, happens from the natural species to the metal surface. Different to this, if the value is around −20 kJ mol
−1 or less negative, than physisorption happens due to electrostatic attraction between the inhibitor and the charged metal surface. Here, in the present study, the value of Δ
G0ads lies in the range of −20 kJ mol
−1 to −40 kJ mol
−1, which most likely indicates that each physisorption and chemisorption occurs inside the framework.
3.5. Potentiodynamic polarization measurements
The impact of the Valeriana wallichii extract concentration on the polarization behaviour of mild steel in 0.5 M H2SO4 at 298 K was analysed and the Tafel plots were recorded for different inhibitor concentrations, as shown in Fig. 5. The corrosion current densities were calculated by extrapolation of the straight parts of the anodic and cathodic curves to the points of intersection of the corresponding corrosion potential. The Tafel curves show a decrease in the current densities of the anodic and cathodic extensions in the presence of Valeriana wallichii extract. This ascertained development is the effect of the coating of adsorbed inhibitor molecules on the surface of the mild steel and the decreased dissolution of the steel surface zone. A higher concentration results in a lower current density (icorr) at 100–500 mg L−1. Surface coverage increases with increasing inhibitor concentration. From the Tafel plots, we obtained the values of the anodic and cathodic Tafel slope (βa & βc), the corrosion current density (icorr), the corrosion potential (Ecorr) and the corrosion inhibition efficiency (IE%) with the help of eqn (11), which are listed in Table 4. | 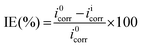 | (11) |
where iicorr and i0corr represent the corrosion current density values with and without inhibitor, respectively.
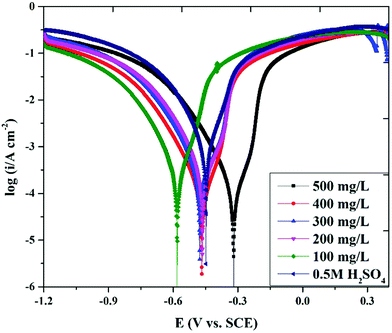 |
| Fig. 5 Potentiodynamic polarization curves for mild steel in 0.5 M H2SO4 with 0–500 mg L−1 concentrations of Valeriana wallichii extract at 298 K with a scan rate of 1 mV s−1. | |
Table 4 Polarization parameters for mild steel in 0.5 M H2SO4 without and with different concentrations of Valeriana wallichii extract at 298 K
Inhibitor concentration (mg L−1) |
−E
corr (mV vs. SCE) |
i
corr (μA cm−2) |
β
a (mV dec−1) |
−βc (mV dec−1) |
Efficiency (IE%) |
θ
|
0 |
465 |
890.90 |
141.66 |
164.26 |
— |
— |
100 |
582 |
195.10 |
60.17 |
111.15 |
78.10 |
0.7810 |
200 |
465 |
183.30 |
58.70 |
111.14 |
79.42 |
0.7942 |
300 |
477 |
177.30 |
75.76 |
111.64 |
80.10 |
0.8010 |
400 |
467 |
62.51 |
45.02 |
109.79 |
92.98 |
0.9298 |
500 |
319 |
58.15 |
36.29 |
114.63 |
93.47 |
0.9347 |
From Fig. 5, every anodic metal disintegration and cathodic hydrogen evolution reaction was inhibited on the addition of inhibitors to the corrosive medium. The inhibition of these reactions was increased on increasing the inhibitor concentration. The fact that the anodic and cathodic Tafel slopes are almost unaffected before and after including the Valeriana wallichii extract demonstrates that the anodic and cathodic reaction mechanisms are controlled by charge transfer.41 Thus, the principle constituents of the Valeriana wallichii extract were adsorbed onto the steel surface and inhibit the corrosion process of mild steel without any change in the reaction mechanism. The Valeriana wallichii extract contains a number of natural compounds, which are not only adsorbed on the steel surface through electrostatic interaction, but can also form strong coordination bonds with iron via their heteroatoms. The blending of physisorption and chemisorption means that the active molecules of the Valeriana wallichii extract are strongly adsorbed onto the steel surface, keeping the steel safe from corrosion, which can be verified by the reduction in the corrosion current density values. The values in Table 4 verify that on increasing the concentration of the Valeriana wallichii extract, the corrosion current density decreases.
3.6. Electrochemical impedance spectroscopy measurements
To study the impedance parameters of the mild steel specimens in 0.5 M H2SO4 with various concentrations of Valeriana wallichii inhibitor, EIS measurements were performed at 298 K. Before every test, the working electrode was immersed in the test solution for 60 minutes at 298 K to attain a steady potential. It is clear from Fig. 6(a) that EOCP remains almost constant after 90 minutes of immersion, which is indicative of a steady state. The temperature remained constant (298 K) for all the tests. To determine the impedance parameters from the experimental outcomes, the data were fitted to the electrical equivalent circuit. Fig. 6(b) shows the electrical equivalent circuit in the presence and absence of inhibitor. Astounding fit outcomes were acquired utilizing these circuits. In the equivalent circuit, Rs is the solution resistance, Rct is the charge exchange resistance and CPE refers to the consistent phase element.
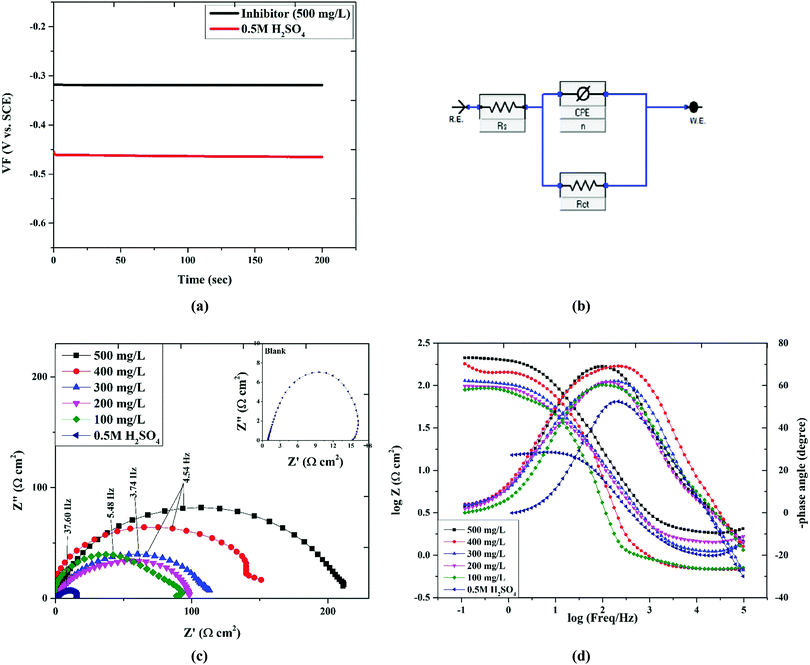 |
| Fig. 6 (a) OCP versus time curves for mild steel in 0.5 M H2SO4 solution in the absence and presence of 500 mg L−1Valeriana wallichii extract. (b) Equivalent circuit used to fit the impedance data of mild steel in 0.5 M H2SO4 solution in the absence and presence of the Valeriana wallichii extract. (c) Nyquist plots for mild steel in 0.5 M H2SO4 with 0–500 mg L−1 concentrations of Valeriana wallichii extract at OCP and 298 K. (d) Bode-Z and Bode-phase plots for mild steel in 0.5 M H2SO4 with 0–500 mg L−1 concentrations of Valeriana wallichii extract at OCP and 298 K. | |
The results are shown in Table 5. The EIS curves (Nyquist and Bode plots) for mild steel with and without Valeriana wallichii extract are shown in Fig. 6(c) and (d). An unfaltering potential (vs. SCE) was promptly accomplished, corresponding to the free Ecorr (vs. SCE) of the mild steel. In the Nyquist graph, due to the charge transfer resistance, the semicircle in each curve corresponds to the time constant. Increasing the Valeriana wallichii extract concentration increases the diameter of the capacitive loop from 100 to 500 mg L−1, which implies a promotion of the inhibition effect. The inhibition efficiency based on the impedance study can be calculated using the following formula:
| 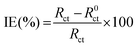 | (12) |
where
Rct and
R0ct are the charge transfer resistance with and without the inhibitor, respectively.
Table 5 EIS parameters for mild steel in 0.5 M H2SO4 without and with different concentrations of Valeriana wallichii extract at 298 K
Concentration of inhibitor (mg L−1) |
R
ct (Ω cm2) |
f
max (Hz) |
R
s (Ω cm2) |
CPE (μF cm−2) |
Efficiency (IE%) |
θ
|
0 |
15.71 |
37.60 |
1.22 |
269.48 |
— |
— |
100 |
89.10 |
5.48 |
0.70 |
325.40 |
82.36 |
0.8236 |
200 |
104.14 |
3.74 |
1.60 |
408.70 |
84.91 |
0.8491 |
300 |
123.09 |
4.54 |
1.30 |
284.83 |
87.23 |
0.8723 |
400 |
153.49 |
4.54 |
0.68 |
228.42 |
89.76 |
0.8976 |
500 |
221.48 |
4.54 |
1.99 |
158.31 |
92.90 |
0.9290 |
The inhibition efficiency is improved on increasing the concentration of the Valeriana wallichii extract, obtaining the highest value of 92.90% at 500 mg L−1. For the EIS curves of the blank solution, the inductive loops exist at low frequency, which results from the relaxation of the adsorbed intermediate products from the steel surface.42 For the rest of the concentrations, the disappearance of the inductive loop is usually regarded as a phenomenon of “degradation” for EIS.43 In the Bode plot, the variation trend of the impedance value with Valeriana wallichii extract concentration in the Bode modulus curves is consistent with that of the semicircle diameter in the Nyquist plot. All phase angle-frequency curves show a single wave, which confirms the one-time constant obtained from the Nyquist plot. According to the literature, if the phase angle equals 90° or 0°, the electrochemical behaviour at the steel solution interface is capacitive or resistive, respectively.44,45 It has been reported that the extract of Valeriana wallichii contains high levels of naphthoic acid, iridoid and an iridoid analogue.33,34 These molecules can be adsorbed onto the surface of the mild steel by donating π-electrons from the aromatic rings or lone-pairs of electrons from the heteroatoms to the vacant orbital of iron. These compounds can form a protective coating to block the mild steel surface from the corrosive medium by obstructing the transfer of charges and ions. The consequence is plainly that the Valeriana wallichii inhibitor inhibits the corrosion of mild steel at any concentration utilized and on increasing the inhibitor concentration, the inhibition effectiveness builds.29
3.7. Theoretical studies
3.7.1. Quantum chemical calculations.
As is already known, a plant extract is an intricate mixture of different phytochemical components. Valeriana wallichii contains naphthoic acid, iridoid and an iridoid analogue as the major chemical constituents. To examine the impact of electronic and molecular structure on the inhibition effectiveness of the inhibitor molecules, quantum chemical calculations have been performed. The optimized structures, lowest unoccupied molecular orbital (LUMO) and highest occupied molecular orbital (HOMO) of the major phytochemical compounds of Valeriana wallichii are shown in Fig. 7. The quantum chemical parameters, EHOMO, ELUMO and ΔE (energy gap between HOMO and LUMO) were computed. A lower value of ELUMO describes a propensity towards the acceptance of electrons from the metal surface, while a higher value of EHOMO shows a tendency of the molecule to give electrons to the metal with empty molecular orbitals. Moreover, a lower value of the energy gap ΔE (ELUMO − EHOMO) will result in good inhibition efficiencies, since the energy required to remove an electron from the most recently involved orbital will be minimized.46 A higher value of the number of transferred electrons (ΔN) demonstrates a higher capacity to give electrons to the metal surface. The electronegativity (χ) parameter can be used to illustrate the electron holding ability of the molecule. The higher the value of χ, the lower the electron transfer by the inhibitor molecule. Electron donation from the inhibitor to the metal increases with increasing softness (σ) and decreases with increasing hardness (η) of the inhibitor. A higher value of π demonstrates better performance for an inhibitor. All of the computed parameters were computed separately according to the following equations:9 | 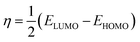 | (14) |
| 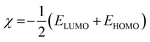 | (15) |
| 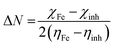 | (16) |
|  | (17) |
| 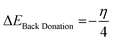 | (18) |
where χinh and χFe represent the electronegativity of the inhibitor molecule and iron, respectively. ηinh and ηFe represent the hardness of the inhibitor molecule and iron, respectively. A value of 7 eV mol−1 has been taken for χFe, and at the same time 0 eV mol−1 has been taken for ηFe, as per Pearson's electronegativity scale.38η is the softness value of the inhibitor molecule and σ is the chemical hardness of the inhibitor molecule. ΔEBack
Donation (eqn (18)) indicates that, at η > 0 and ΔEBack
Donation < 0, the charge transfer to a molecule, via back-donation starting with the molecule, is favoured energetically, and π is the chemical potential.47,48
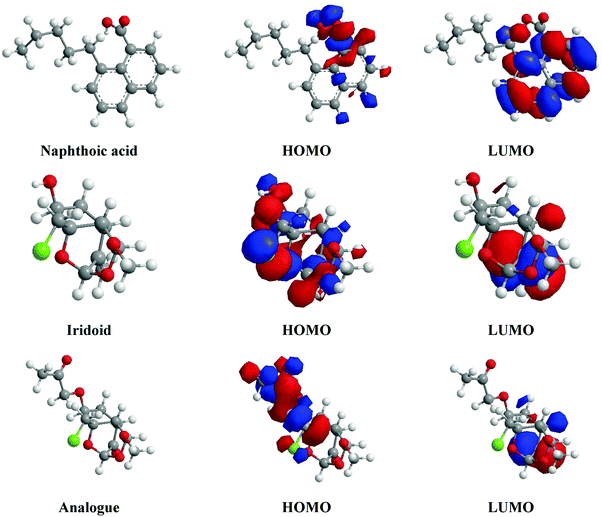 |
| Fig. 7 Optimized structures and frontier molecular orbital density distributions (HOMO & LUMO) obtained by the DFT/B3LYP/6-31G+(d,p) method of naphthoic acid, iridoid and the iridoid analogue. | |
Table 6 reveals the key parameters of the significant phytochemical components of Valeriana wallichii, which are thought to be important because of their direct impact on the electronic interaction between the inhibitor molecule and the surface of the mild steel. As per Table 6, the value of EHOMO of the studied molecules follows the order: naphthoic acid > iridoid > iridoid analogue, which indicates the order of donation of electrons to the vacant orbital of Fe. Naphthoic acid has the lowest ELUMO (−0.39 eV), which shows that it has the highest capability to accept electrons from the d-orbital of Fe, and also naphthoic acid has the lowest ΔE (0.79 eV), which shows the good stability of the [naphthoic acid–Fe] complex. The fact that it has the highest value of ΔN (7.86 e) shows that naphthoic acid has the greatest ability to donate electrons to the metal surface. The higher value of ΔEBack
Donation of naphthoic acid shows the higher stability of the [Fe–naphthoic acid] complex. The higher value of χ of naphthoic acid (0.78 eV) shows that it has the lowest tendency of electron donation to the vacant d-orbital of Fe. Furthermore, inspection of the obtained data shows that the value of χ decreases with increasing softness (σ) and decreasing hardness (η) of the inhibitor molecule.36 Taking every computed parameter and the molecular structures into account, the inhibition effect follows the order: naphthoic acid > iridoid > iridoid analogue. Naphthoic acid can be assumed to be the most essential component in the inhibition performance of the Valeriana wallichii extract. However, since the molecules change extensively under specific condition, any of them might exert a dominant impact; for example, if the examination conditions change, specific characteristics might in turn be upgraded or alternatively suppressed, which may result in a change in the inhibition performance of the Valeriana wallichii extract. In addition, it should be noted that all of the determined parameters were procured in the gas phase and the dissolution effects were neglected. In spite of the fact that a couple of analysts have shown that the registered parameters in the gas phase exhibit no critical difference compared to those in the vicinity of a solvent, it should be pointed out that this strategy can't be exact.18 As per the literature, specific isolated phytochemical constituents from plant extracts exhibit comparatively low inhibition efficiencies as compared to mixtures of various phytochemical constituents, which means that a mixture of naphthoic acid, iridoid and the iridoid analogue will show better results than each component separately.
Table 6 Calculated quantum chemical parameters of naphthoic acid, iridoid and the iridoid analogue obtained from the DFT/B3LYP/6-31G+(d,p) method in the gas phase
Molecule |
E
HOMO (eV) |
E
LUMO (eV) |
ΔE (eV) |
μ (D) |
ΔN (e) |
ΔEBack-Donation (eV) |
η (eV) |
σ (eV−1) |
χ (eV) |
π (eV) |
Naphthoic acid |
−1.18 |
−0.39 |
0.79 |
1.80 |
7.86 |
−0.09 |
0.39 |
2.53 |
0.78 |
−0.78 |
Iridoid |
−1.40 |
−0.11 |
1.29 |
1.20 |
4.84 |
−0.16 |
0.64 |
1.55 |
0.75 |
−0.75 |
Iridoid analogue |
−1.50 |
−0.37 |
1.13 |
1.30 |
5.36 |
−0.14 |
0.56 |
1.76 |
0.93 |
−0.93 |
3.8. SEM analysis
SEM micrographs of the mild steel surface following 24 h of immersion in 0.5 M H2SO4 at 298 K with (500 mg L−1) and without inhibitor are shown in Fig. 8. As is apparent from the figure, the smooth surface of the polished mild steel (Fig. 8(a)) is determinedly damaged in the absence of inhibitors (Fig. 8(b)) because of the intensive metal dissolution, resulting in an exceedingly porous surface with extensive and profound holes/cracks. In contrast, in the presence of inhibitor (Fig. 8(c)), the steel surface is significantly improved.
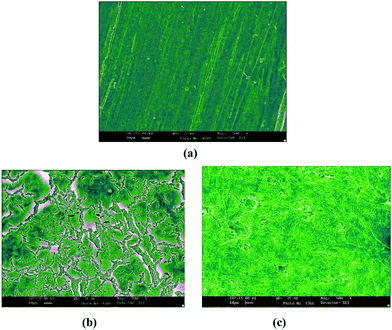 |
| Fig. 8 SEM micrographs of the mild steel surface after 24 h of immersion at 298 K in 0.5 M H2SO4: (a) abraded mild steel, (b) without inhibitor and (c) with the inhibitor (500 mg L−1). | |
3.9. AFM analysis
Three-dimensional AFM images of the polished mild steel, the uninhibited mild steel and the inhibited mild steel in 0.5 M H2SO4 with Valeriana wallichii extract are shown in Fig. 9(a)–(c). Fig. 9(a) shows that the average roughness value of the polished mild steel is 2.99 nm, with a maximum height of 60 nm. Fig. 9(b) shows that in the absence of the Valeriana wallichii extract, the surface of the mild steel is strongly damaged due to the dissolution of the metal in the acid solution. The average surface roughness in this case is 138.81 nm, with a maximum height of 2.1 μm (2100 nm). Meanwhile, Fig. 9(c) shows the appearance of the mild steel surface with the Valeriana wallichii extract, which has an average surface roughness value of 45.50 nm and a maximum height of 0.12 μm (120 nm). The decrease in height and average surface roughness of the mild steel specimen is due to the adsorption of the Valeriana wallichii inhibitor on the metal surface. It can be said that a protective layer is formed on the surface of the metal.
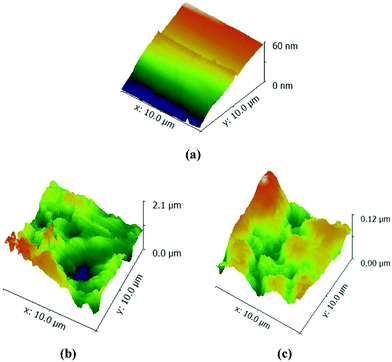 |
| Fig. 9 AFM images of the mild steel surface after 24 h of immersion at 298 K in 0.5 M H2SO4: (a) abraded mild steel, (b) without inhibitor and (c) with the inhibitor (500 mg L−1). | |
3.10. Proposed mechanism of action
The corrosion inhibition of mild steel in 0.5 M H2SO4 solution by the Valeriana wallichii root extract can be explained based on molecular adsorption. In most of the inhibition studies, the formation of donor–acceptor surface complexes between the lone pair/π-electrons of the inhibitor molecules and the empty d-orbitals of the metal was suggested. As mentioned above, the investigated inhibitor has major phytochemical constituents of naphthoic acid, iridoid and an iridoid analogue, which possess a number of heteroatoms and aromatic rings, and thus act as Lewis bases and form coordinate bonds with the empty d-orbitals of the metal, consequently being adsorbed on the metal surface with the creation of a protective layer on the metal surface, which protects the metal from corrosion. The inhibitor has a free energy of adsorption of −25.42 kJ mol−1 at 298 K, which demonstrates that the mechanism of adsorption of the inhibitor molecule on the mild steel surface is a mixture of both chemical and physical adsorption. The adsorption of the inhibitor molecule on the surface of the mild steel occurs through the displacement of adsorbed water molecules from the mild steel surface and the transfer of electrons between the heteroatoms and Fe. Fig. 10 shows a schematic representation of the adsorption behaviour of naphthoic acid on a mild steel surface.
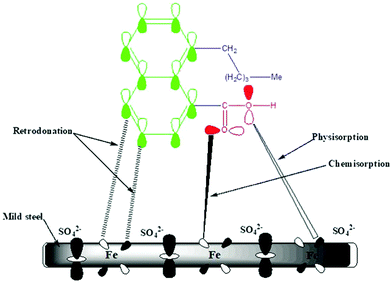 |
| Fig. 10 Schematic representation of the adsorption behaviour of the naphthoic acid molecule on a mild steel surface. | |
4. Conclusion
The inhibition behaviour of a root extract of Valeriana wallichii was scrutinized using weight loss, electrochemical studies, AFM and SEM surface investigation, UV-visible, FTIR and 1H NMR spectroscopy procedures, and quantum chemical calculations. As per the theoretical calculations, the inhibition efficiencies of the major phytochemical constituents of Valeriana wallichii towards the corrosion of mild steel in 0.5 M H2SO4 decreases in the order of naphthoic acid > iridoid > iridoid analogue. The potentiodynamic polarization estimations demonstrated that this natural product is a mixed-type inhibitor and acts as an adsorptive inhibitor, so by increasing the inhibitor concentration (up to 500 mg L−1), the inhibition effectiveness can be increased up to 93.47%. Electrochemical impedance spectroscopy indicated that the CPE of the electrical double layer decreased, and also the charge transfer resistance increased on increasing the inhibitor concentration. These conclusions additionally confirm that the Valeriana wallichii extract acts just via adsorption at the metal/solution interface. The experimental data were fit well by the Langmuir adsorption isotherm model, and ΔG0ads confirms that these inhibitor molecules are strongly adsorbed onto the mild steel surface via both physisorption and chemisorption. Quantum chemical calculations predict that the naphthoic acid is the best inhibitor for preventing corrosion.
The inhibition behaviour of the Valeriana wallichii extract was additionally confirmed by taking SEM micrographs and AFM pictures of the surfaces exposed to the inhibited solution, which was in extraordinary agreement with the electrochemical tests and weight loss estimations. The most extreme inhibition effectiveness of 93.47% was achieved utilizing an inhibitor concentration of 500 mg L−1 in 0.5 M H2SO4 at 298 K.
Conflicts of interest
There is no conflict of interest.
References
- M. K. Bagga, R. Gadi, O. S. Yadav, R. Kumar, R. Chopra and G. Singh, Investigation of phytochemical components and corrosion inhibition property of Ficus racemosa stem extract on mild steel in H2SO4 medium, J. Environ. Chem. Eng., 2016, 4, 4699–4707 CrossRef CAS.
- K. H. Hassan, A. A. Khadom and N. H. Kurshed,
Citrus aurantium roots extracts as a sustainable corrosion inhibitor of mild steel in sulfuric acid, S. Afr. J. Chem. Eng., 2016, 22, 1–5 Search PubMed.
- M. A. Amin, S. S. Abd El-Rehim, E. E. F. El-Sherbini and R. S. Bayoumi, The inhibition of low carbon steel corrosion in hydrochloric acid solutions by succinic acid. Part I. Weight loss, polarization, EIS, PZC, EDX and SEM studies, Electrochim. Acta, 2007, 52, 3588–3600 CrossRef CAS.
- S. Garai, S. Garai, P. Jaisankar, J. K. Singh and A. Elango, A comprehensive study on crude methanolic extract of Artemisia pallens (Asteraceae) and its active component as effective corrosion inhibitors of mild steel in acid solution, Corros. Sci., 2012, 60, 193–204 CrossRef CAS.
- M. S. Al-Otaibi,
et al., The effect of temperature on the corrosion inhibition of mild steel in 1 M HCL solution by Curcuma longa extract, Int. J. Electrochem. Sci., 2013, 5, 847–859 Search PubMed.
- V. G. Vasudha and K. Shanmuga Priya,
Polyalthia longifolia as a corrosion inhibitor for mild steel in HCl solution, Res. J. Chem. Sci., 2013, 3, 21–26 CAS.
- N. Patel, A. Rawat, S. Jauhari and G. Mehta, Inhibitive action on Bridelia restusa roots extract on corrosion of mild steel in acidic media, Eur. J. Chem., 2010, 1, 129–133 CrossRef CAS.
- T. Ibrahim, H. Alayan and Y. Al Mowaqet, The effect of Thyme roots extract on corrosion of mild steel in HCl, Prog. Org. Coat., 2012, 75, 456–462 CrossRef CAS.
- Z. Salarvand, M. Amirnasr, M. Talebian, K. Raeissi and S. Meghdadi, Enhanced corrosion resistance of mild steel in 1 M HCl solution by trace amount of 2-phenyl-benzothiazole derivatives: experimental, quantum chemical calculations and molecular dynamics (MD) simulation studies, Corros. Sci., 2017, 114, 133–145 CrossRef CAS.
- S. Shahabi, P. Norouzi and M. R. Ganjali, Electrochemical and theoretical study of the inhibition effect of two synthesized thiosemicarbazide derivatives on carbon steel corrosion in hydrochloric acid solution, RSC Adv., 2015, 5, 20838–20847 RSC.
- I. Ahamad, R. Prasad and M. A. Quraishi, Experimental and quantum chemical characterization of the adsorption of some Schiff base compounds of phthaloyl thiocarbohydrazide on the mild steel in acid solutions, Mater. Chem. Phys., 2010, 124, 1155–1165 CrossRef CAS.
- D. Daoud, T. Douadi, H. Hamani, S. Chafaa and M. Al-Noaimi, Corrosion inhibition of mild steel by two new S-heterocyclic compounds in 1 M HCl: experimental and computational study, Corros. Sci., 2015, 94, 21–37 CrossRef CAS.
- S. Hejazi,
et al., Electrochemical and quantum chemical study of thiazolo-pyrimidine derivatives as corrosion inhibitors on mild steel in 1 M H2SO4, J. Ind. Eng. Chem., 2015, 25, 112–121 CrossRef CAS.
- H. M. Abd El-Lateef, M. A. Abo-Riya and A. H. Tantawy, Empirical and quantum chemical studies on the corrosion inhibition performance of some novel synthesized cationic gemini surfactants on carbon steel pipelines in acid pickling processes, Corros. Sci., 2016, 108, 94–110 CrossRef CAS.
- G. Gece, The use of quantum chemical methods in corrosion inhibitor studies, Corros. Sci., 2008, 50, 2981–2992 CrossRef CAS.
- V. V. Torres,
et al., Study of thioureas derivatives synthesized from a green route as corrosion inhibitors for mild steel in HCl solution, Corros. Sci., 2014, 79, 108–118 CrossRef CAS.
- N. Soltani, N. Tavakkoli, M. Khayatkashani, M. R. Jalali and A. Mosavizade, Green approach to corrosion inhibition of 304 stainless steel in hydrochloric acid solution by the extract of Salvia officinalis roots, Corros. Sci., 2012, 62, 122–135 CrossRef CAS.
- L. Li, X. Zhang, J. Lei, J. He, S. Zhang and F. Pan, Adsorption and corrosion inhibition of Osmanthus fragran roots extract on carbon steel, Corros. Sci., 2012, 63, 82–90 CrossRef CAS.
- G. Ji, S. Anjum, S. Sundaram and R. Prakash,
Musa paradisica peel extract as green corrosion inhibitor for mild steel in HCl solution, Corros. Sci., 2015, 90, 107–117 CrossRef CAS.
- A. A. Rahim, E. Rocca, J. Steinmetz, M. J. Kassim, R. Adnan and M. Sani Ibrahim,
Mangrove tannins and their flavanoid monomers as alternative steel corrosion inhibitors in acidic medium, Corros. Sci., 2007, 49, 402–417 CrossRef CAS.
- S. Deng and X. Li, Inhibition by Jasminum nudiflorum Lindl. roots extract of the corrosion of aluminium in HCl solution, Corros. Sci., 2012, 64, 253–262 CrossRef CAS.
- A. Ostovari, S. M. Hoseinieh, M. Peikari, S. R. Shadizadeh and S. J. Hashemi, Corrosion inhibition of mild steel in 1 M HCl solution by henna extract: a comparative study of the inhibition by henna and its constituents (Lawsone, Gallic acid, alpha-D-Glucose and Tannic acid), Corros. Sci., 2009, 51, 1935–1949 CrossRef CAS.
- X. Li and S. Deng, Inhibition effect of Dendrocalamus brandisii roots extract on aluminum in HCl, H3PO4 solutions, Corros. Sci., 2012, 65, 299–308 CrossRef CAS.
- V. V. Torres,
et al., Inhibitory action of aqueous coffee ground extracts on the corrosion of carbon steel in HCl solution, Corros. Sci., 2011, 53, 2385–2392 CrossRef CAS.
- P. C. Okafor, M. E. Ikpi, I. E. Uwah, E. E. Ebenso, U. J. Ekpe and S. A. Umoren, Inhibitory action of Phyllanthus amarus extracts on the corrosion of mild steel in acidic media, Corros. Sci., 2008, 50, 2310–2317 CrossRef CAS.
- I. Radojčić, K. Berković, S. Kovač and J. Vorkapić-Furač, Natural honey and black radish juice as tin corrosion inhibitors, Corros. Sci., 2008, 50, 1498–1504 CrossRef.
- S. Deng and X. Li, Inhibition by Ginkgo roots extract of the corrosion of steel in HCl and H2SO4 solutions, Corros. Sci., 2012, 55, 407–415 CrossRef CAS.
- D. I. Njoku, I. Ukaga, O. B. Ikenna, E. E. Oguzie, K. L. Oguzie and N. Ibisi, Natural products for materials protection: corrosion protection of aluminium in hydrochloric acid by Kola nitida extract, J. Mol. Liq., 2016, 219, 417–424 CrossRef CAS.
- E. A. Noor, The impact of some factors on the inhibitory action of Radish fruits aqueous extract for mild steel corrosion in 1 M H2SO4 solution, Mater. Chem. Phys., 2011, 131, 160–169 CrossRef CAS.
- M. A. Quraishi, A. Singh, V. K. Singh, D. K. Yadav and A. K. Singh, Green approach to corrosion inhibition of mild steel in hydrochloric acid and sulphuric acid solutions by the extract of Murraya koenigii roots, Mater. Chem. Phys., 2010, 122, 114–122 CrossRef CAS.
- X. Li, S. Deng and H. Fu, Inhibition of the corrosion of steel in HCl, H2SO4 solutions by bamboo leaf extract, Corros. Sci., 2012, 62, 163–175 CrossRef CAS.
- A. Saxena, D. Prasad and R. Haldhar, Use of Butea monosperma extract as green corrosion inhibitor, Int. J. Electrochem. Sci., 2017, 12, 8793–8805 CrossRef CAS.
- A. Pande and Y. N. Shukla, Naphthoic and derivative from Valeriana willichi, Phytochemistry, 1993, 32, 1350–1351 CrossRef CAS.
- R.-J. Wang,
et al., Iridoids from the roots of Valeriana jatamansi jones, Phytochemistry, 2017, 141, 156–161 CrossRef CAS PubMed.
- S. Sahu,
et al., Valeriana wallichii root extract improves sleep quality and modulates brain monoamine level in rats, Phytomedicine, 2012, 924–929 CrossRef PubMed.
- E. Toolika, N. Bhat and S. Shetty, A comparative clinical study on the effect of Tagara (Valeriana wallichii DC.) and Jatamansi (Nardostachys jatamansi DC.) in the management of Anidra (primary insomnia), AYU, 2015, 36, 46 CrossRef CAS PubMed.
- R. Haldhar, D. Prasad and A. Saxena,
Myristica fragrans extract as an eco-friendly corrosion inhibitor for mild steel in 0.5 M H2SO4, J. Environ. Chem. Eng., 2018, 6, 2290–2301 CrossRef CAS.
- C. Verma, M. A. Quraishi, E. E. Ebenso, I. B. Obot and A. El Assyry, 3-Amino alkylated indoles as corrosion inhibitors for mild steel in 1 M HCl: experimental
and theoretical studies, J. Mol. Liq., 2016, 219, 647–660 CrossRef CAS.
- G. Sığırcık, D. Yildirim and T. Tüken, Synthesis and inhibitory effect of N,N′-bis(1-phenylethanol)ethylenediamine against steel corrosion in HCl Media, Corros. Sci., 2017, 120, 184–193 CrossRef.
- R. Kumar, O. S. Yadav and G. Singh, Electrochemical and surface characterization of a new eco-friendly corrosion inhibitor for mild steel in acidic media: a cumulative study, J. Mol. Liq., 2017, 237, 413–427 CrossRef CAS.
- K. Rose, B. S. Kim, K. Rajagopal, S. Arumugam and K. Devarayan, Surface protection of steel in acid medium by Tabernaemontana divaricata extract: physicochemical evidence for adsorption of inhibitor, J. Mol. Liq., 2016, 214, 111–116 CrossRef CAS.
- I. Ahamad and M. A. Quraishi, Mebendazole: new and efficient corrosion inhibitor for mild steel in acid medium, Corros. Sci., 2010, 52, 651–656 CrossRef CAS.
- H. Ashassi-Sorkhabi and E. Asghari, Effect of hydrodynamic conditions on the inhibition performance of l-methionine as a ‘green’ inhibitor, Electrochim. Acta, 2008, 54, 162–167 CrossRef CAS.
- X. Zheng, S. Zhang, W. Li, L. Yin, J. He and J. Wu, Investigation of 1-butyl-3-methyl-1H-benzimidazolium iodide as inhibitor for mild steel in sulfuric acid solution, Corros. Sci., 2014, 80, 383–392 CrossRef CAS.
- M. Mahdavian and M. M. Attar, Electrochemical behaviour of some transition metal acetylacetonate complexes as corrosion inhibitors for mild steel, Corros. Sci., 2009, 51, 409–414 CrossRef CAS.
- M. S. Morad, An electrochemical study on the inhibiting action of some organic phosphonium compounds on the corrosion of mild steel in aerated acid solutions, Corros. Sci., 2000, 42, 1307–1326 CrossRef CAS.
- E. E. Oguzie, M. A. Chidiebere, K. L. Oguzie, C. B. Adindu and H. Momoh-Yahaya, Biomass extracts for materials protection: corrosion inhibition of mild steel in acidic media by Terminalia chebula extracts, Chem. Eng. Commun., 2014, 201, 790–803 CrossRef CAS.
- K. F. Khaled, Monte Carlo simulations of corrosion inhibition of mild steel in 0.5 M sulphuric acid by some green corrosion inhibitors, J. Solid State Electrochem., 2009, 13, 1743–1756 CrossRef CAS.
Footnote |
† Electronic supplementary information (ESI) available. See DOI: 10.1039/c8qm00120k |
|
This journal is © the Partner Organisations 2018 |
Click here to see how this site uses Cookies. View our privacy policy here.