DOI:
10.1039/C7RA11695K
(Paper)
RSC Adv., 2018,
8, 4125-4129
A fast-responsive two-photon fluorescent probe for in vivo imaging superoxide radical anion with a large stokes shift†
Received
23rd October 2017
, Accepted 14th January 2018
First published on 23rd January 2018
Abstract
A novel and simple two-photon fluorescent probe NS-O for the detection of superoxide radical anion (O2˙−) with a large turn-on fluorescence signal is presented. The probe NS-O exhibited favorable properties including fast response, high selectivity, large stokes shift, low cytotoxicity and almost 100-fold fluorescence enhancement in solution. The two-photon probe could be applied to monitor endogenous superoxide radical anions in living cells and tissues. Notably, the two-photon fluorescent probe NS-O could be used to image superoxide radical anions in zebrafish.
Introduction
Reactive oxygen species (ROSs) including superoxide radical anion (O2˙−), hydrogenperoxide (H2O2), hydroxyl radical (˙OH), and peroxynitrite (ONOO−), a broad range of small signaling molecules with a highly reactive unpaired valence or an odd number of electrons, are important in the immune process and defense of organisms against bacteria, viruses, and parasites.1 Although ROSs are crucial to maintain some internal environmental balances of organisms, their imbalances could affect the homeostasis of the biosystem,2 and lead to a range of related diseases including arthritis, cancer, oxidative stress arteriosclerosis and so on.3 It is worth noting that the superoxide radical anion (O2˙−) is linked to many inflammatory diseases.4 The superoxide radical anion (O2˙−) with strong oxidation, which is related to the generation of most of the reactive oxygen and nitrogen species, could be obtained from disproportionation of hydrogen peroxide (H2O2).5 Therefore, it is very important to develop useful methods with high sensitivity and good selectivity for imaging the superoxide radical anion (O2˙−) in biosamples.
Some analytical methods had been developed for the detection of superoxide radical anion exploited in the previous reports including mass spectrometry (MS), high performance liquid chromatography (HPLC), and electron paramagnetic resonance spectroscopy (EPR).6 However, these methods are only applied to extracellular detection. In the past few years, organic fluorescent probes, which have been proved to be the most useful monitoring tools, have become an important method used in biological studies with good merits including high sensitivity, good selectivity and real-time detection.7
Fluorescence imaging has been developed as a powerful method for monitoring various signaling molecules in the past few decades. In recent years, a number of fluorescent probes for the detection of the superoxide radical anion had been reported,8 some of which were developed by deprotection of the leaving group by the superoxide radical anion. Also, other reports were constructed by means of oxydehydrogenation. Therefore, it is important to develop a fast-responsive fluorescent probe with a large turn-on signal and stokes shifts for imaging the superoxide radical anion (O2˙−). Importantly, with the development of two-photon fluorescent probe, the merits including long excitation wavelengths, three-dimensional detection of living tissues, depressed the photodamage to biological samples, increased penetration ability of tissue and reduced fluorescent interference of background have been attracted more and more groups. Thus, it is necessary to develop a two-photon fluorescent probe for specifical detection of the superoxide radical anion (O2˙−) in living cells, tissues and zebrafish.
In this report, a fast-responsive two-photon fluorescent probe NS-O has been developed for monitoring endogenous superoxide radical anion with a large turn-on signal (Scheme 1). This novel two-photon probe NS-O exhibits good selectivity over other analytes. Besides, the cell imaging, tissue imaging and the zebrafish imaging have proved to be that the probe NS-O could be suitable to image the level of superoxide radical anion in living biosamples. Therefore, the two-photon probe NS-O is expected to be applied to monitor superoxide radical anion in biosamples.
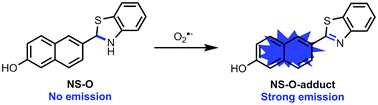 |
| Scheme 1 Proposed sensing mechanism of the probe NS-O with KO2. | |
Results and discussion
1. Probe design and synthesis
We choose the fluorescent compound of 6-hydroxy-2-naphthaldehyde with good properties as the fluorophore. By the way of simple modification of aldehyde group, the two-photon fluorescent probe NS-O was designed and synthesized in Scheme 2 with one step. The structure of the probe NS-O was totally characterized by 1H NMR, 13C NMR and HRMS (Fig. S5–S7†).
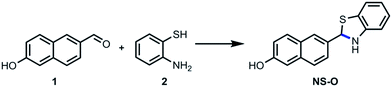 |
| Scheme 2 Synthesis of the probe NS-O. | |
2. The sensing property of NS-O probe for the superoxide radical anion (O2˙−)
The fast-responsive two-photon probe NS-O was used to examine optical properties in presence of the superoxide radical anion (O2˙−). The solubility of probe NS-O was measured in Fig. S1a† and the absorption spectroscopy was also listed without or with the addition of the superoxide radical anion (O2˙−) (Fig. S1b†). The probe NS-O showed no fluorescence with excitation at 400 nm without the addition of the superoxide radical anion (O2˙−) (Fig. 1). In contrast, the strong fluorescence emission were exhibited at 531 nm in the presence of the superoxide radical anion (O2˙−) with a large stokes shift. With the increasing addition of the superoxide radical anion (O2˙−), the fluorescence enhancement of probe NS-O increased notably (Fig. 1). And the detection limit for the superoxide radical anion was about 1.71 μM (Fig. S2†). Therefore, the two-photon probe NS-O was sensitive to the superoxide radical anion (O2˙−), which could be applied to monitor the superoxide radical anion (O2˙−) as a useful tool.
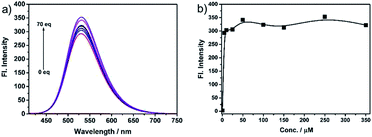 |
| Fig. 1 (a) Fluorescence spectra of NS-O (5 μM) in pH 7.4 PBS/DMSO (v/v = 1/1) in the absence or presence of KO2 (5 equiv.). (b) Fluorescence intensity at 531 nm at different concentrations. | |
In order to investigate the mechanism, the research of probe NS-O was measured when added with the superoxide radical anion (O2˙−) and the process was measured by mass spectrometry. A peak at m/z 278.0632 was showed in the ESI-MS spectrum in good agreement with the predicted NS-O-adduct (Fig. S3†). The result was in accordance with the proposed sensing mechanism depicted in Scheme 1.
We further investigated the time courses of probe NS-O (5 μM) in presence of the superoxide radical anion (O2˙−, 5 equiv.) (Fig. 2). Notably, the fluorescence intensity was increased to maximum instantly with the addition of the superoxide radical anion (O2˙−) (about 2 min) which shows that the probe NS-O exhibits fast response. As to the pH value of PBS buffer, we treated the probe NS-O with different pH of PBS buffer in Fig. 3. And the fluorescence intensity was almost no change without the addition of the superoxide radical anion (O2˙−), while the pH of PBS buffer changed from 4.0 to 10.0. That is to say, the probe NS-O was stable in the wide pH range. Obviously the fluorescence intensity increased gradually from 6.0 to 10.0 in the presence of the superoxide radical anion (O2˙−). The data show that the probe NS-O can image the superoxide radical anion (O2˙−) at physiological pH (7.4).
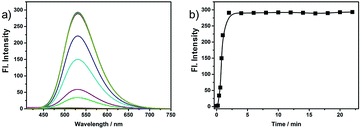 |
| Fig. 2 (a) Fluorescence spectra of NS-O (5 μM) in pH 7.4 PBS/DMSO (v/v = 1/1) in the absence or presence of KO2. (b) Fluorescence intensity at 531 nm as a function of time. | |
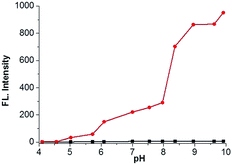 |
| Fig. 3 pH effects of fluorescence spectra of NS-O (5 μM) in different pH PBS buffer (containing 50% DMSO) in the absence ( ) or presence ( ) of KO2 (5 equiv.). | |
The selectivity research, another important and relevant property of probe NS-O, was also measured. When we treated the probe NS-O with other analytes, such as H2O2 (100 μM), NaClO (100 μM), ˙OH (100 μM), DTBP (100 μM), TBHP (100 μM), NO (100 μM), NaNO2 (2.5 mM), NaNO3 (2.5 mM), GSH (5 mM), Hcy (5 mM), Cys (5 mM), FeSO4 (2.5 mM), KI (2.5 mM), Na2S (2.5 mM), CaCl2 (2.5 mM), MgCl2 (2.5 mM), CuCl2 (2.5 mM), ZnCl2 (2.5 mM), there are no fluorescence intensity and opposite phenomena was obtained in presence of the superoxide radical anion (O2˙−), which was shown in Fig. 4. The research on selectivity had been proved to be that the probe NS-O is suitable for bioimaging in the complicated biosystem. Therefore, the two-photon probe NS-O could be applied for monitoring the superoxide radical anion (O2˙−) with high selectivity of other interfering molecules.
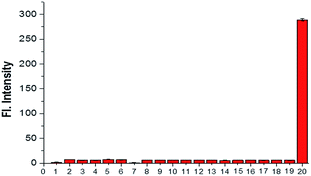 |
| Fig. 4 The fluorescence intensity of probe NS-O (10 μM) in the presence of various analytes in PBS buffer (pH 7.4 PBS/DMSO (v/v = 1/1)). (1) Free probe; (2) H2O2 (100 μM); (3) NaClO (100 μM); (4) ˙OH (100 μM); (5) DTBP (100 μM); (6) TBHP (100 μM); (7) NO (100 μM); (8) NaNO2 (2.5 mM); (9) NaNO3 (2.5 mM); (10) GSH (5 mM); (11) Hcy (5 mM); (12) Cys (5 mM); (13) FeSO4 (2.5 mM); (14) KI (2.5 mM); (15) Na2S (2.5 mM); (16) CaCl2 (2.5 mM); (17) MgCl2 (2.5 mM); (18) CuCl2 (2.5 mM); (19) ZnCl2 (2.5 mM); (20) KO2 (50 μM). | |
3. Cytotoxicity and imaging
The above results indicate that the probe NS-O has good properties including fast response, a very large turn-on signal and stokes shifts, good selectivity. Thus, the probe NS-O might be used for monitoring the superoxide radical anion (O2˙−) in real biosamples. We evaluated imaging assays of probe NS-O in living cells, and fluorescence imaging was completed in HeLa cells by the way of confocal laser scanning microscopy.
The cytotoxicity of probe NS-O was measured in living HeLa cells by a MTT assay (Fig. S4†). The results indicate that the probe NS-O exhibits no marked cytotoxicity to HeLa cells after a long period (>90% HeLa cells survived after 24 h with NS-O (30.0 μM) incubation). Thus, the probe NS-O could be applied to image the superoxide radical anion (O2˙−) in living cells with low cytotoxicity.
Due to the good properties and low cytotoxicity of probe NS-O, we want to know whether the probe NS-O could be responsive in living HeLa cells. The utility of NS-O for cell imaging of the superoxide radical anion (O2˙−) was investigated in HeLa cells (Fig. 4). When HeLa cells were incubated with incubated with a superoxide scavenger Tiron (200 μM) for 20 min, NS-O probe (10.0 μM) for 30 min and KO2 (10 equiv.) for another 20 min, clearly detectable fluorescence was observed. For control experiment, when the cells were pre-treated with a superoxide scavenger Tiron (200 μM) for 20 min, and then incubated with probe (10 μM) for another 30 min, no marked fluorescence was shown in the blue channel (Fig. 5e) at the same test conditions. However, when we treated the HeLa cells with Tiron (200 μM) for 20 min and stimulated with 2-ME (100 μM) for 30 min and then incubated with probe (10 μM) for 30 min, strong fluorescence enhancement was exhibited in the blue channel in Fig. 5h, confirming that the probe could be fit for in vivo imaging.
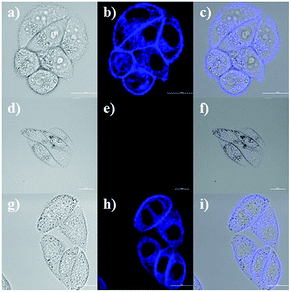 |
| Fig. 5 Fluorescence response of the NS-O probe in living HeLa cells. (a) Bright-field image of live HeLa cells incubated with a superoxide scavenger Tiron (200 μM) for 20 min, NS-O probe (10.0 μM) for 30 min and KO2 (10 equiv.) for another 20 min; (b) fluorescence image of (a); (c) overlay of brightfield and blue channel. (d) Bright-field image of live HeLa cells pretreated with a superoxide scavenger Tiron (200 μM) for 20 min, and then incubated with probe (10 μM) for another 30 min; (e) fluorescence image of (d); (f) overlay of brightfield and blue channel; (g) bright-field image of live HeLa cells pretreated with Tiron (200 μM) for 20 min and stimulated with 2-ME (100 μM) for 30 min and then incubated with probe (10 μM) for 30 min. (h) Fluorescence image of (g); (i) overlay of brighfield and blue channel. Excitation at 405 nm. Scale bar = 20 μm. | |
Encouraged with in vivo cell imaging of probe NS-O, tissue imaging was operated by the way of two-photon fluorescence microscopy. We prepared the living tissues slices of rat liver with thickness at 400 μm. The living tissue slices incubated with a superoxide scavenger Tiron (200 μM) for 30 min and NS-O probe (20.0 μM) for 30 min in PBS buffer exhibit no fluorescence at the emission window of 0–40 nm (Fig. 6a). However, when we treated tissue slices with a superoxide scavenger Tiron (200 μM) for 30 min, NS-O (20 μM) and KO2 (10 equiv.) for another 30 min, significant fluorescence was shown in Fig. 6e at the depth of 40 μm in living tissues by two-photon fluorescence microscopy.
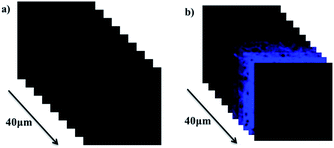 |
| Fig. 6 (a) Two-photon fluorescence images of a fresh mouse liver slice incubated with a superoxide scavenger Tiron (200 μM) for 30 min and NS-O probe (20.0 μM) for 30 min in PBS buffer exhibit no fluorescence at the emission window of 0–40 nm. (b) Two-photon fluorescence images of a fresh mouse liver slice pretreated with a superoxide scavenger Tiron (200 μM) for 30 min, NS-O (20 μM) and KO2 (10 equiv.) in PBS buffer at the depths of approximately 0–40 μm. Excitation at 800 nm with fs pulse. | |
Due to the advantages of fast response and large stokes shifts, the two-photon probe NS-O was further applied to image the superoxide radical anion (O2˙−) in living zebrafish. As listed in Fig. 7b, zebrafish exhibited nearly no fluorescence in blue channel when the probe NS-O was pretreated with a superoxide scavenger Tiron (200 μM) for 20 min and NS-O (20.0 μM) for 30 min. In contrast, when we the treated the living zebrafish with Tiron (200 μM), NS-O (20.0 μM) and KO2 (10 equiv.) for 30 min, a clear fluorescence enhancement was imaged in two zygomorphic areas around its yolk extension and eyes in the blue channel with excitation at 405 nm in Fig. 6e. The above results indicated that the two-photon probe NS-O could be capable of monitoring the superoxide radical anion (O2˙−) in living cells, tissues and zebrafish by means of fluorescence microscopy.
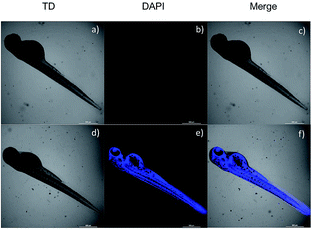 |
| Fig. 7 Zebrafish imaging in stained with the probe NS-O (a) brightfield image of zebrafish costained with Tiron (200 μM) for 30 min and NS-O (20.0 μM) for 30 min; (b) fluorescence images of (a) from blue channel; (c) overlay of (a) and (b); (d) brightfield image of zebrafish incubated with Tiron (200 μM) for 30 min, NS-O (20.0 μM) and KO2 (10 equiv.) for 30 min; (e) fluorescence images of (d) from blue channel; (f) overlay of the brightfield image (d) and blue channels (e). | |
Experimental
1. Materials and instruments
Unless otherwise noted, all reagents and materials were purchased from commercial company and used without further purification. Twice-distilled water was applied to all experiments. High-resolution electronspray (ESI-HRMS) mass spectra were examined from Bruker APEX IV-FTMS 7.0T mass spectrometer; NMR spectra were obtained from AVANCE III 400 MHz Digital NMR Spectrometer with TMS as an internal standard; electronic absorption spectra were recorded on a LabTech UV Power spectrometer; photoluminescent spectra were obtained with a HITACHI F4600 fluorescence spectrophotometer; the fluorescence images were collected with Nikon A1MP confocal microscopy with a CCD camera; the pH measurements were implemented on a Mettler-Toledo Delta 320 pH meter; analysis was exhibited on silica gel plates and column chromatography was carried out over silica gel (mesh 200–300). Both TLC and were purchased from the Qingdao Ocean Chemicals.
2. Synthesis of the two-photon probe NS-O
A mixture of 6-hydroxy-2-naphthaldehyde (0.5 mmol, 86.0 mg, 1.0 equiv.) and 2-aminobenzenethiol (0.55 mmol, 68.8 mg, 1.1 equiv.) was added in EtOH (5.0 mL) under N2. The solution was refluxed for 5 h until TLC indicated the end of reaction. After cooling to room temperature, the mixture was concentrated under vacuum, and the product was obtained by silica column chromatography to give the probe NS-O in the yield of 63%; 1H NMR (100 MHz, DMSO-d6): δ 10.12 (s, 1H), 8.56 (s, 1H), 8.17–8.01 (m, 4H), 7.86 (d, J = 8.8 Hz, 1H), 7.58–7.45 (m, 2H), 7.22–7.18 (m, 2H). 13C NMR (100 MHz, DMSO-d6): δ 168.2, 157.6, 154.2, 136.6, 134.8, 131.2, 127.8, 127.8, 127.7, 127.6, 127.1, 125.8, 124.7, 123.1, 122.8, 120.3, 109.4. HRMS: m/z [M + H]+ calcd for [C17H14NOS]+: 280.0708, found 280.0705.
3. Preparation of solutions of probe NS-O and analytes
Without other noted, all the tests were operated according to the following procedure. A stock solution (1.0 mM) of NS-O was prepared in DMSO. In a 10 mL tube the test solution of compounds NS-O was prepared by placing 0.09 mL of stock solution, 4.5 mL of DMSO, 4.5 mL of 0.1 M PBS buffer and an appropriate volume of KO2 sample solution. After adjusting the final volume to 10 mL with 0.1 M PBS buffer, standing at room temperature, 3 mL portion of it was transferred to a 1 cm quartz cell to measure absorbance or fluorescence. All fluorescence measurements were conducted at room temperature on a Hitachi F4600 Fluorescence spectrophotometer. The slight pH variations of the solutions were achieved by adding the minimum volumes of NaOH (0.1 M) or HCl (0.2 M).
4. Cytotoxicity assays
The living cells line were treated in DMEM (Dulbecco's Modified Eagle Medium) supplied with fetal bovine serum (10%, FBS), penicillin (100 U mL−1) and streptomycin (100 μg mL−1) under the atmosphere of CO2 (5%) and air (95%) at 37 °C. The HeLa cells were then seeded into 96-well plates, and 0, 1, 5, 10, 20, 30 μM (final concentration) of the probe NS-O (99.9% DMEM and 0.1% DMSO) were added respectively. Subsequently, the cells were cultured at 37 °C in an atmosphere of CO2 (5%) and air (95%) for 24 hours. Then the HeLa cells were washed with PBS buffer, and DMEM medium (100 μL) was added. Next, MTT (10 μL, 5 mg mL−1) was injected to every well and incubated for 4 h. Violet formazan was treated with sodium dodecyl sulfate solution (100 μL) in the H2O–DMF mixture. Absorbance of the solution was measured at 570 nm by the way of a microplate reader. The cell viability was determined by assuming 100% cell viability for cells without NS-O.
5. Cell imaging
HeLa cells were grown in modified Eagle's medium (MEM) replenished with 10% FBS with the atmosphere of 5% CO2 and 95% air at 37 °C for 24 h. The HeLa cells were washed with PBS when used. As to the in vivo imaging of endogenous O2˙−, the living HeLa cells were incubated with various concentration of a superoxide scavenger Tiron and 2-methoxyestradiol (2-ME) and then treated with NS-O (10 μM) for another 30 min at 37 °C. For control imaging, HeLa cells treated with a superoxide scavenger Tiron for 20 min and NS-O (10.0 μM) for 30 min. The ideal fluorescence images were acquired with a Nikon A1MP confocal microscopy with the equipment of a CCD camera.
6. Tissue imaging
The Kunming mice were purchased from Shandong University Laboratory Animal Center (Jinan, China). All procedures for this study were approved by the Animal Ethical Experimentation Committee of Shandong University according to the requirements of the National Act on the use of experimental animals (China). The fresh mouse liver slices were obtained from the liver of 14 day-old mouse. The living liver slices were gained with 400 micron thickness using a vibrating-blade microtome in 25 mM PBS (pH 7.4). The living liver slices were pre-treated with a superoxide scavenger Tiron (200 μM) for 30 min and NS-O (20 μM) for 30 min. The slices were washed three times by PBS buffer and imaged. To obtain the two-photon fluorescence images of the tissues, the slices were pre-treated with a superoxide scavenger Tiron (200 μM) for 30 min and NS-O (20 μM) for 30 min before the KO2 was added. Following this incubation for another 30 min at 37 °C, the slices were washed three times by PBS buffer and imaged. The two-photon fluorescence emission was collected upon excitation at 800 nm with a femtosecond laser.
7. Zebrafish imaging
We incubated the 5 day-old zebrafish with NS-O (20 μM) in embryo media for 20 minutes at 37 °C. After washing with PBS three times, we further incubated zebrafish with the superoxide radical anion (O2˙−, 10 equiv.) for another 20 min at 37 °C. Then the zebrafish was washed with PBS three times. For control group, the zebrafish was incubated with probe NS-O (20 μM) for 20 min at 37 °C and washed with PBS buffer three times. The zebrafish imaging was obtained by Nikon A1MP confocal microscopy inverted fluorescence microscopy equipped with a cooled CCD camera.
Conclusions
In conclusion, a large turn-on signal fluorescent probe NS-O was developed for imaging endogenous superoxide radical anion (O2˙−) with two-photon excitation. Desirable properties, such as fast response, high selectivity, large stokes shifts and low cytotoxicity, were exhibited. Notably, the probe NS-O could be applied to monitor endogenous superoxide radical anion in the living cells. Besides, the tissue and zebra fish imaging proved to be that the novel probe NS-O could be helpful for investigation and detection of superoxide radical anion in living organisms.
Conflicts of interest
There are no conflicts to declare.
Acknowledgements
This work was financially supported by NSFC (21704047), Natural Science Foundation of Shandong Province (ZR2017BB078).
Notes and references
-
(a) L. A. Sena and N. S. Chandel, Mol. Cell, 2012, 48, 158–167 CrossRef CAS PubMed;
(b) W. M. Nauseef, Semin. Immunopathol., 2008, 30, 195–208 CrossRef CAS PubMed;
(c) M. Inoue, E. F. Sato, M. Nishikawa, A.-M. Park, Y. Kira, I. Imada and K. Utsumi, Curr. Med. Chem., 2003, 10, 2495–2505 CrossRef CAS PubMed.
-
(a) B. M. Babior, Curr. Opin. Immunol., 2004, 16, 42–47 CrossRef CAS PubMed;
(b) G. Groeger, C. Quiney and T. G. Cotter, Antioxid. Redox Signaling, 2009, 11, 2655–2671 CrossRef CAS PubMed;
(c) M. Valko, D. Leibfritz, J. Moncol, M. T. Cronin, M. Mazur and J. Telser, Int. J. Biochem. Cell Biol., 2007, 39, 44–84 CrossRef CAS PubMed.
-
(a) C. Nathan, J. Clin. Invest., 2003, 111, 769–778 CrossRef CAS PubMed;
(b) B. Halliwell, J. Gutteridge and C. E. Cross, J. Lab. Clin. Med., 1992, 119, 598–620 CAS;
(c) A. P. Wickens, Respir. Physiol., 2001, 128, 379–391 CrossRef CAS PubMed;
(d) D. C. Wallace, Science, 1999, 283, 1482–1488 CrossRef CAS PubMed.
- K. Kundu, S. F. Knight, N. Willett, S. Lee, W. R. Taylor and N. Murthy, Angew. Chem., Int. Ed., 2009, 48, 299–303 CrossRef CAS PubMed.
-
(a) B. C. Dickinson and C. J. Chang, Nat. Chem. Biol., 2011, 7, 504–511 CrossRef CAS PubMed;
(b) A. W. Linnane, M. Kios and L. Vitetta, Mitochondrion, 2007, 7, 1–5 CrossRef CAS PubMed;
(c) C. C. Winterbourn, Free Radical Biol. Med., 1993, 14, 85–90 CrossRef CAS;
(d) I. A. Abreu and D. E. Cabelli, Biochim. Biophys. Acta, Proteins Proteomics, 2010, 1804, 263–274 CrossRef CAS PubMed.
-
(a) M. Yamato, T. Egashira and H. Utsumi, Free Radical Biol. Med., 2003, 35, 1619–1631 CrossRef CAS;
(b) W. M. Armstead, Brain Res., 2001, 910, 19–28 CrossRef CAS PubMed;
(c) Y. Saito, Y. Yoshida, T. Akazawa, K. Takahashi and E. Niki, J. Biol. Chem., 2003, 278, 39428–39434 CrossRef CAS PubMed;
(d) G. M. Rosen and B. A. Freeman, Proc. Natl. Acad. Sci. U. S. A., 1984, 81, 7269–7273 CrossRef CAS PubMed;
(e) H. Yasui and H. Sakurai, Biochem. Biophys. Res. Commun., 2000, 269, 131–136 CrossRef CAS PubMed.
-
(a) J. R. Lakowicz, Principles of Fluorescence Spectroscopy, Springer, New York, 2006 Search PubMed;
(b) Y. Yang, Q. Zhao, W. Feng and F. Li, Chem. Rev., 2013, 113, 192–270 CrossRef CAS PubMed.
-
(a) J. Niu, J. Fan, X. Wang, Y. Xiao, X. Xie, X. Jiao, C.-Z. Sun and B. Tang, Anal. Chem., 2017, 89, 7210–7215 CrossRef CAS PubMed;
(b) R.-Q. Li, Z.-Q. Mao, L. Rong, N. Wu, Q. Lei, J.-Y. Zhu, L. Zhuang, X.-Z. Zhang and Z.-H. Liu, Biosens. Bioelectron., 2017, 87, 73–80 CrossRef CAS PubMed;
(c) J. Zhang, C. Li, R. Zhang, F. Zhang, W. Liu, X. Liu, S. M.-Y. Leeb and H. Zhang, Chem. Commun., 2016, 52, 2679–2682 RSC;
(d) F. Si, Y. Liu, K. Yan and W. Zhong, Chem. Commun., 2015, 51, 7931–7934 RSC;
(e) K. Xu, X. Liu and B. Tang, ChemBioChem, 2007, 8, 453–458 CrossRef CAS PubMed;
(f) H. Maeda, K. Yamamoto, Y. Nomura, I. Kohno, L. Hafsi, N. Ueda, S. Yoshida, M. Fukuda, Y. Fukuyasu, Y. Yamauchi and N. Itoh, J. Am. Chem. Soc., 2005, 127, 68–69 CrossRef CAS PubMed;
(g) J. J. Gao, K. H. Xu, B. Tang, L. L. Yin, G. W. Yang and L. G. An, FEBS J., 2007, 274, 1725–1733 CrossRef CAS PubMed.
Footnote |
† Electronic supplementary information (ESI) available. See DOI: 10.1039/c7ra11695k |
|
This journal is © The Royal Society of Chemistry 2018 |
Click here to see how this site uses Cookies. View our privacy policy here.