DOI:
10.1039/C7RA11710H
(Paper)
RSC Adv., 2018,
8, 284-290
Synthesis and characterization of poly(amide-imide)s derived from a new ortho-functional unsymmetrical dicarboxylic acid†
Received
23rd October 2017
, Accepted 12th December 2017
First published on 2nd January 2018
Abstract
A new diacid monomer containing an imide ring and a methoxy pendant group, 3-trimellitimido-4-methoxybenzoic acid TMBA, was synthesized and characterized. The diacid was used to synthesize six novel poly(amide-imide)s, (PAIs), through a polycondensation reaction with different diamines in the presence of triphenylphosphite, calcium chloride, and pyridine in N-methyl-2-pyrrolidinone. The chemical structures of the diacid monomer and poly(amide-imide)s were confirmed by FTIR and 1H NMR spectroscopy. All PAIs were obtained in high yield with inherent viscosities ranging from 0.32 to 0.54 dL g−1. They were soluble in aprotic solvents, and exhibited film forming ability. The PAI films displayed glass transition temperatures between 281 and 377 °C, as determined by dynamic mechanical analysis, and they show high thermal stability. Gas permeability results for TMBA-6FDA film show that this PAI could be an interesting material for polymeric membranes aimed to CO2 separation process.
1. Introduction
Poly(amide-imide)s (PAI)s combine the superior mechanical properties associated with the amine group, and the high thermal stability of the imide ring allowing a combination intended to attain high-performance polymers.1 These properties make them appropriate for different applications as substitutes for some high performance polyamides and polyimides. Generally, the synthesis of PAIs is carried out through two main routes: (a) polycondensation through amide-bond formation utilizing monomers containing a preformed imide-bond; and (b) polycondensation through imide-bond formation utilizing monomers containing a preformed amide-bond.2 Based on these two approaches, the PAIs have been prepared using trimellitic anhydride (TMA), as a strategy to synthesize symmetric monomers with either preformed amide- or imide-bond.3–7
The synthesis of novel PAIs with specific functionalities in the backbone may provide a way of controlling certain physical properties or special functions. Feng et al.8 have reported the preparation of biodegradable PAIs based on diacid monomers containing amino acids. On the other hand, Rafiee7 synthesized a triptycene-containing dicarboxylic acid monomer, which was polymerized to obtain organosoluble PAIs with high performance properties. Sarkar et al.,9 reported the synthesis of PAIs based on aromatic diamine monomers with preformed amine bonds and pendent n-alkyloxy side chains, these PAIs exhibited good properties to align liquid crystals (LC). Typically, PAIs are synthesized from symmetrical monomers.10,11 However, the incorporation of unsymmetrical monomers into the polymer backbone decreases packing density and crystallinity which allows obtaining soluble high performance polymers.12 Moreover, the incorporation of chemical functionalities into the PAIs backbone could lead to preparation of thermally rearranged polymers that may find use in polymer membrane technology.13–15
This study reports the synthesis and characterization of a new ortho-functional unsymmetrical dicarboxylic acid containing an imide ring and a methoxy pendant group, 3-trimellitimido-4-methoxybenzoic acid. This low cost monomer is easy to obtain from a condensation reaction between trimellitic anhydride and a non-conventional aminoacid. Furthermore, to the best of our knowledge, there are not reports in the literature of its synthesis and application for polymer preparation. Additionally, the synthesis, characterization and some physical properties of six novel PAIs derived from the new diacid monomer are described. Moreover, the effect of PAI structure on pure gas permeability coefficients for the six different PAIs films was also investigated and discussed.
2. Experimental
2.1 Materials
All reactants used for the nonconventional aminoacid monomer and PAIs synthesis were purchased from Sigma-Aldrich. The reactants 3-amino-4-methoxybenzoic acid (AMA), trimellitic anhydride (TMA), 2,4,6-trimethyl-1,3-phenylenediamine (3MPD), 2,3,5,6-tetramethyl-1,4-phenylenediamine (4MPD), 4,4′-hexafluoroisopropylidenedianiline (6FDA), 4,4′-(hexafluoroisopropylidene)bis(p-phenyloxy)dianiline (BAPHF), 4,4′-(9-fluorenylidene)diamine (FND), 4,4′-(1,4-phenylenediisopropylidene)bisaniline (PDBI), N-methyl-2-pyrrolidinone (NMP), triphenylphosphite (TPP), and pyridine (Py) were used as received. Calcium chloride (CaCl2) was dried under vacuum at 200 °C overnight prior to use. All other solvents and reagents were used as received without further purification.
2.2 Characterizations
FTIR spectra were recorded on with a Nicolet 8700 (ThermoScientific) FTIR spectrometer. The diacid monomer and poly(amide-imide)s were analysed using KBr pellets and films, respectively. The spectra were obtained in the transmittance mode on the range of 4000 to 400 cm−1, with 32 scans using a resolution of 4 cm−1. 1H NMR spectra were acquired on a 600 MHz Varian VNMRS spectrometer using deuterated dimethylsulfoxide (DMSO-d6) as solvent with tetramethylsilane as an internal reference. Solubility tests were carried out placing 5 mg of PAIs into 1 mL of solvent at room temperature followed by stirring during 24 h. Thermogravimetric analyses (TGA) were performed using a TGA-8000 (Perkin Elmer, Inc) thermogravimetric balance. 5 to 8 mg film samples were analysed between 50 and 800 °C, at a heating rate of 10 °C min−1 under nitrogen atmosphere. Dynamic mechanical analysis (DMA) of PAIs films was performed on a DMA-Q800 (TA Instruments) the measurements were carried out using rectangular films 20 mm long × 1 mm wide × 0.1 mm thick in the tension mode. Measurements were performed as a function of temperature between 50 and 400 °C at a heating rate of 5 °C min−1 using a static force of 110 mN and an 88 mN dynamic force at 1 Hz. A Shimadzu AGS-X universal testing machine was employed to determine the tensile properties of the films. Stress–strain curves were obtained with a 100 N load cell using a 1 mm min−1 strain rate according to ASTM-D882-02. Inherent viscosity (ηinh) was determined with an Ubbelohde viscometer, size 50, on 0.5 g dL−1 polymer solutions in dimethylacetamide (DMAc) at 30 °C. PAIs films density was determined by the density gradient column method (Techne Corp) using calcium nitrate solutions (1.22 to 1.47 g cm−3). Pure gas permeability coefficients were determined using a constant volume, variable pressure permeation cell, at 35 °C and 2 atm. The effective film area was 1.13 cm2. Before each permeation test, the film was degassed for 24 h. Gas permeability coefficients, P, were determined from the slope of the downstream pressure versus time plot under steady-state conditions (dp/dt)ss, from the following equation:
where V is the cell downstream volume (cm3), RN is the molar volume of a gas molecule at standard conditions (STP), l and A are the thickness (cm) and effective area (cm2) of the film exposed to the gas. R is the gas constant, T is the absolute temperature in K, and Δp is the pressure differential (cm Hg) between the feed gas and the downstream of the permeation cell.
2.3 Monomer synthesis
2.3.1 3-Trimellitimido-4-methoxybenzoic acid (TMBA). A mixture of 1.67 g (10 mmol) of 3-amino-4-methoxybenzoic acid (AMA), 1.92 g (10 mmol) of trimellitic anhydride (TMA), and 20 mL of dimethylformamide (DMF) was refluxed at 160 °C during 18 h. After cooling at room temperature, the reaction mixture was poured into 500 mL of cold water. The resulting precipitate was filtered off, and then washed thoroughly with water. The crude product obtained was recrystallized from isopropanol obtaining a creamy solid. (2.2 g, yield: 64.5%) Mp: 312 °C. FT-IR: (KBr: cm−1) 3400–3300 (O–H stretch, broad), 1780 (C
O asymm. stretch), 1700 (C
O symm. stretch), 1380 (C–N stretch), 740 (C–N bending), and 1090 (C–O–C stretch). 1H-NMR (600 MHz, DMSO-d6) δ 8.43 (d, J = 8.0 Hz, Ha, 1H), 8.32 (s, Hb, 1H), 8.09 (m, Hc, Hd, 2H), 8.01 (d, J = 8.0 Hz, He, 1H), 7.34 (d, J = 8.0 Hz, Hf, 1H), 3.84 (s, Hg, 3H). 13C NMR (600 MHz, DMSO-d6) δ 166.29 (Ca), 165.91 (C1), 165.90 (Cb), 165.74 (C3), 158.73 (C2′), 136.65 (C8), 135.60 (C6), 134.84 (C9), 132.42 (C4′), 132.04 (C1′), 131.64 (C6′), 123.95 (C5), 123.52 (C7), 123.20 (C4), 119.91 (C5′), 112.23 (C3′), 56.37 (Cc) (Fig. S1†).
2.4 Poly(amide-imide)s synthesis
PAIs were synthesized by direct polycondensation of TMBA with six aromatic diamines (3MPD, 4MPD, 6FDA, FND, PDBI, BAPFH) using Yamazaki's method.16 A typical synthesis procedure for trimellitimido-methoxy-carboxylic hexafluroisopropylidene poly(amide-imide), TMBA-6FDA, was carried out as follows: a mixture of 0.68 g (2 mmol) of TMBA, 0.67 g (2 mmol) of 6FDA, previously sublimated, 0.8 g CaCl2 previously dried at 200 °C overnight, 1.4 mL (17.31 mmol) of pyridine (Py), 1.4 mL (5.34 mmol) of triphenylphosphite (TPP), and 8.8 mL of NMP were charged into a three neck round bottom flask equipped with a nitrogen inlet/outlet, and a mechanical stirrer. The reaction mixture was stirred, and heated at 105 °C during 24 h. After cooling at room temperature, the resulting viscous solution was poured slowly into 700 mL of a water/ethanol mixture (1
:
3 v/v). The resulting fibrous precipitated was recuperated by filtration, washed thoroughly with methanol and hot water and dried at 100 °C under vacuum for 24 h.
2.5 PAIs films preparation
PAIs dense films were prepared using a solvent casting method. Briefly, 0.3 g of PAI were placed in 6 mL of DMF; next, they were stirred until a homogeneous solution was obtained. The polymeric solution was filtered and poured into an aluminium mould. The solvent was slowly evaporated at 80 °C during 24 h. After that, the dense films were peeled off and subsequently dried under vacuum at 150 °C for 24 h. Solvent elimination was verified by TGA in the interval of temperature between 50 and 250 °C. Dense films for all PAIs were obtained with thicknesses ranging from 65 to 80 μm.
3. Results and discussion
3.1 Monomer synthesis
Fig. 1 shows the synthetic route for the ortho-functional unsymmetrical dicarboxylic acid, TMBA. This strategy is a simple one-pot reaction where 3-amino-4-methoxybenzoic acid was reacted with trimellitic anhydride under dimethylformamide reflux to obtain a preformed imide ring.17,18 The FTIR spectrum of TMBA monomer (Fig. 2A) showed the characteristic absorption of carboxylic acid bands (3400–3300 cm−1, OH–stretch), and imide heterocyclic ring (1780 cm−1 C
O symm, 1700 cm−1 C
O asymm, and 1380 cm−1 C–N stretch). Other important signal was observed at 1090 cm−1 corresponding to the methoxy group ether bond (C–O–C stretch). The 1H NMR spectrum of TMBA was used to confirm the proposed chemical structure (Fig. 2B). First, no signal from amine groups was detected. Second, the proton signals of carboxylic acids were detected at 13.0 ppm. Finally, the signals in the range of 7.3–8.4 ppm were assigned to aromatic protons and the singlet at 3.84 ppm corresponds to methoxy group protons present in the ortho-position of AMA. On the basis of these spectroscopic data, the synthesis of a new monomer, TMBA, was successfully achieved; thus, the new monomer was employed for the synthesis of several novel PAIs.
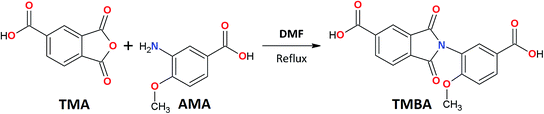 |
| Fig. 1 Scheme of TMBA monomer synthesis. | |
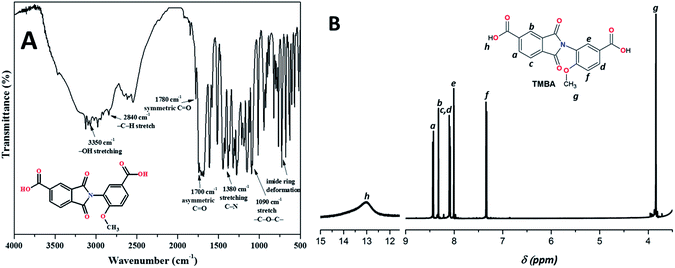 |
| Fig. 2 (A) FTIR and (B) 1H NMR TMBA spectra. | |
3.2 Poly(amide-imide)s synthesis
Traditionally, the phosphorylation polycondensation reaction is reported for condensation of diamines and diacids to obtain polyamides.16 Using this approach TMBA was reacted with six commercial diamines as shown in Fig. 3. 3MPD and 4MPD were considered due to the fact that they have a short and stiff structure. 6FDA and FND were selected because they present bulky pendant groups that hinder rotations of the polymer chain. On the other hand, PDBI and BAPHF were taken into account to understand the effect that long and flexible sections have on the PAIs. All polymerization reactions produce homogeneous and highly viscous solutions with up to 95% yield.
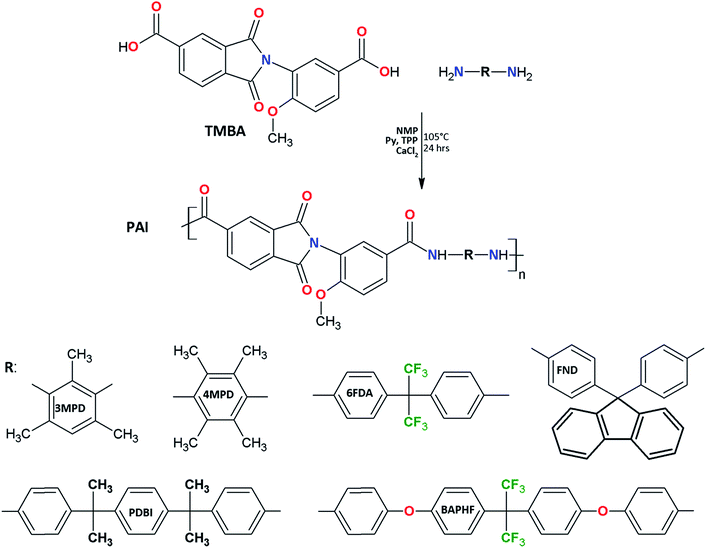 |
| Fig. 3 Synthesis of poly(amide-imide)s. | |
The main disadvantage of both polyamides and polyimides is their insolubility, which difficults their processing. The poly(amide-imide)s synthesized from the monomer TMBA were soluble in polar aprotic solvents at a concentration of 5 mg mL−1, and in some cases partially soluble in THF. They were insoluble in chlorinated solvents (Table 1). Based on these solubility results, their inherent viscosities were determined in DMAc. Inherent viscosity values range from 0.32 to 0.54 dL g−1 which is indicative of polymers formation with moderate molecular weights.7,19 These values were acceptable to elaborate flexible dense films by the solution casting method.
Table 1 PAIs solubility and inherent viscositya
PAI |
Solvents |
ηinh (dL g−1) |
NMP |
DMAc |
DMF |
DMSO |
THF |
DCM |
TCE |
CHCl3 |
Measured in DMAc (0.5 g dL−1) at 30 °C. Solubility (5 mg mL−1): +, soluble, −, insoluble, ±, partially soluble at room temperature. |
TMBA-3MPD |
+ |
+ |
+ |
+ |
− |
− |
− |
− |
0.47 |
TMBA-4MPD |
+ |
+ |
+ |
+ |
− |
− |
− |
− |
0.46 |
TMBA-6FDA |
+ |
+ |
+ |
+ |
± |
− |
− |
− |
0.54 |
TMBA-FND |
+ |
+ |
+ |
+ |
− |
− |
− |
− |
0.47 |
TMBA-PDBI |
+ |
+ |
+ |
+ |
± |
− |
− |
− |
0.32 |
TMBA-BAPHF |
+ |
+ |
+ |
+ |
± |
− |
− |
− |
0.34 |
All PAIs chemical structures were confirmed by FTIR and 1H NMR spectroscopy. FTIR spectra of all PAIs (Fig. S2†) show the formation of the amide bond that was easily identified due to the presence of characteristic bands at 3300 cm−1 (N–H stretching) and at 1660 cm−1 (C
O stretching). Moreover, the resulting PAIs also showed signals at 1780 cm−1 and 1720 cm−1 (C
O symmetrical and asymmetrical stretching), and at 1370 cm−1 (C–N stretching) corresponding to the imide ring, and at 2930 cm−1 (C–H stretching) of methyl groups present in TMBA. Fig. 4 shows, as an example, the FTIR spectrum of the poly(amide-imide) TMBA-6FDA. Furthermore, in the 1H NMR spectra of all PAIs (Fig. S3A and S3B†) there were observed two signals in the region between 10.2 and 10.9 ppm and 9.8 to 10.5 ppm, these signals were attributed to amide protons (–CO–NH–) formed by the condensation reaction between diacids and diamines; moreover, these results confirmed the unsymmetrical structure of TMBA, where the amide proton downfield corresponds to TMA, while the amide proton observed upfield corresponds to the AMA moiety. Fig. 5 shows, as an example, the assignments of protons for the repeating unit from poly(amide-imide) TMBA-6FDA.
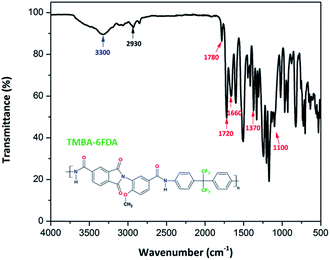 |
| Fig. 4 FTIR spectrum of PAI TMBA-6FDA. | |
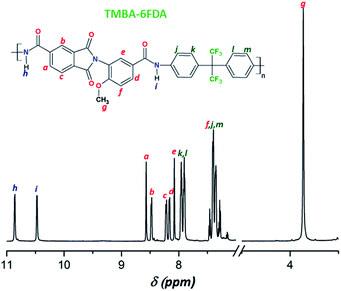 |
| Fig. 5 1H NMR spectrum of PAI TMBA-6FDA. | |
3.3 Thermal properties
Thermal behaviour of all PAIs was evaluated by DMA and TGA techniques under nitrogen atmosphere the results are summarized in Table 2. Glass transition temperature (Tg) was obtained from the peak in tan delta curves from DMA analysis (Fig. S4†).20 Tg's of poly(amide-imide)s were in the range of 281 to 377 °C. The Tg depended on the diamine moiety structure. When the diamine is rigid and bulky, such as FND, the poly(amide-imide) TMBA-FND displayed the highest Tg; whereas when the diamine has flexible linkages, as is the case of the poly(amide-imide) TMBA-BAPHF, Tg was the lowest due to a higher flexibility associated with the ether (C–O–C) groups present in this diamine moiety. On the other hand, all aromatic PAIs exhibited two thermal degradation steps (Table 2, Fig. 6, and Fig. S5†). The first weight loss was observed around 368 up to 426 °C and it was ascribed to the elimination of the methoxy pendant groups present in the TMBA, which could be converted to benzoxazole rings by thermal rearrangement;22–24 meanwhile the second weight loss in the range of 496 to 592 °C was ascribed to the polymer backbone degradation. Moreover, the char yield at 800 °C was found in the range of 53 to 56 wt%. The later data were employed to calculate the limiting oxygen index (LOI). Based on LOI values obtained on PAIs these polymers can be classified as auto-extinguishing materials.7,25,26
Table 2 Thermal properties of PAIs
PAIs |
Tg (°C), DMAa |
Tmax1b (°C) |
Tmax2 (°C) |
Char yieldc (%) |
LOId |
Measured by DMA at heating rate of 5 °C min−1 and 1 Hz. Tmax, onset of decomposition temperature of the two events observed. At 800 °C in N2 atmosphere. Limiting oxygen index (LOI) calculated from equation LOI = 17.5 + 0.4 (char yield).21 |
TMBA-3MPD |
353 |
373 |
516 |
59.7 |
41.3 |
TMBA-4MPD |
367 |
368 |
496 |
59.7 |
41.3 |
TMBA-6FDA |
347 |
423 |
578 |
53.5 |
38.9 |
TMBA-FND |
377 |
426 |
592 |
66.3 |
44.0 |
TMBA-PDBI |
286 |
419 |
541 |
55.0 |
39.4 |
TMBA-BAPHF |
281 |
419 |
575 |
56.6 |
40.1 |
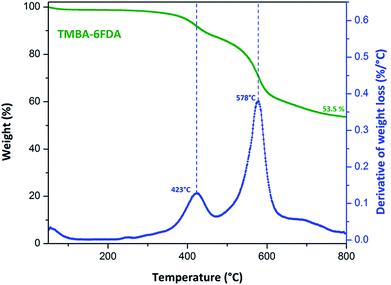 |
| Fig. 6 TGA and DTGA curves of PAI TMBA-6FDA. | |
3.4 Mechanical properties
Mechanical properties of PAIs films, obtained by the solvent casting method, were characterized by the tensile test and the results are summarized in Table 3. Tensile properties of the PAI TMBA-3MPD were not measured because the film was highly brittle. The other PAIs films present tensile strengths ranging from 52 to 71 MPa. Tensile modulus was found to be between 1.3 and 1.8 GPa while elongation at break percent lies in between 3.5 and 9.0%. These results are consistent with data previously reported in the literature for aromatic PAIs and suggest that the PAIs dense films based on TMBA present high mechanical properties.6,7,10,17
Table 3 PAIs mechanical properties under tension
PAI |
Tensile modulus (GPa) |
Tensile strength (MPa) |
Elongation at break (%) |
TMBA-3MPD |
— |
— |
— |
TMBA-4MPD |
1.3 |
64.1 |
6.4 |
TMBA-6FDA |
1.6 |
63.9 |
6.4 |
TMBA-FND |
1.8 |
55.9 |
3.5 |
TMBA-PDBI |
1.4 |
52.0 |
4.5 |
TMBA-BAPHF |
1.4 |
71.9 |
9.0 |
3.5 Gas permeability coefficient
Polymeric gas separation membranes have great potential for industrial gas separation process because of their cost-effectiveness and lower energy requirements in applications such as natural gas sweeting.27–29 The potential application of different poly(amide-imide)s as gas separation membranes has been previously reported.30–32 In here, pure gas permeability coefficients of the novel PAIs films prepared were tested using a constant-volume, variable pressure gas permeation cell at 35 °C and 2 atm. Results of pure gas permeability coefficients for He, O2, CO2, CH4 and N2 for the six PAIs and a commercial PAI (Torlon) for comparison are given in Table 4. Gas permeability coefficients for the TMBA-based PAIs decrease in the order PHe > PCO2 > PO2 > PN2 > PCH4. This behaviour agrees with the kinetic diameter order of the gases evaluated: He (2.6 Å) > CO2 (3.3 Å) > O2 (3.46 Å) > N2 (3.64 Å) > CH4 (3.8 Å).
Table 4 PAIs pure gas permeability coefficients
PAI |
Permeabilitya, P, (Barrer) |
αb |
He |
CO2 |
O2 |
N2 |
CH4 |
CO2/CH4 |
1 Barrer = 10−10 cm3 (STP) cm s−1 cm−2 cmHg−1, evaluated at 35 °C and 2 atm. Ideal separation factor. |
TMBA-3MPD |
14.5 |
2.4 |
0.73 |
0.38 |
0.37 |
6.5 |
TMBA-4MPD |
27.3 |
5.8 |
1.35 |
0.56 |
0.52 |
11.1 |
TMBA-6FDA |
46.5 |
12.3 |
3.10 |
0.73 |
0.64 |
19.2 |
TMBA-FND |
29.6 |
9.1 |
2.43 |
1.12 |
1.11 |
8.2 |
TMBA-PBDI |
19.0 |
7.4 |
1.63 |
0.48 |
0.48 |
15.4 |
TMBA-BAPHF |
28.3 |
10.9 |
2.41 |
0.64 |
0.58 |
18.8 |
Torlon33 |
3.73 |
0.54 |
0.13 |
0.018 |
0.013 |
41.5 |
PAIs films showed significant changes on pure gas permeability coefficient depending on the kind of diamine used for its preparation. For example, CO2 permeability coefficients, PCO2 decrease in the order TMBA-6FDA > TMBA-BAPHF > TMBA-FND > TMBA-PDBI > TMBA-4MPD > TMBA-3MPD. Interestingly, TMBA-6FDA film also shows the highest ideal selectivity for the gas pair CO2/CH4. However, its selectivity was lower than the one reported for Torlon.33 Gas permeability in a dense polymeric film is related with structural parameters which are responsible of molecular packing such as fractional free volume.
The concept of fractional free volume (FFV) has been helpful to explain the gas permeability behaviour in polymeric membranes. FFV is defined as:
in which,
V is the specific volume of the polymer, calculated as:
where
ρ is the measured density given in
Table 5, and
Vo is the polymer chain occupied volume that was calculated from:
where,
VW is the van der Waals volume of the polymer chain repeating unit, that was calculated by Bondi's group contribution method.
34,35
Table 5 PAIs density and fractional free volume
PAI |
ρ (g cm−3) |
V (cm3 mol−1) |
Vwd (cm3 mol−1) |
FFV |
TMBA-3MPD |
1.279 |
356.14 |
240.58 |
0.122 |
TMBA-4MPD |
1.278 |
367.37 |
254.25 |
0.100 |
TMBA-6FDA |
1.420 |
450.35 |
288.77 |
0.166 |
TMBA-FND |
1.288 |
507.53 |
332.77 |
0.148 |
TMBA-PDBI |
1.248 |
520.59 |
347.57 |
0.132 |
TMBA-BAPHF |
1.391 |
592.16 |
386.37 |
0.152 |
In the present work, PAIs gas permeability coefficients show a good correlation with FFV. In particular, TMBA-6FDA and TMBA-BAPHF, that contain the bulky –CF3 group, presented higher FFV which is associated to higher pure gas permeability. They also show the best CO2/CH4 ideal selectivity which is linked to the presence of –CF3 groups.
4. Conclusions
In summary, a new ortho-functional unsymmetrical dicarboxylic acid, 3-trimellitimido-4-methoxybenzoic acid (TMBA) was successfully synthesized and its chemical structure confirmed. Moreover, novel poly(amide-imide)s were prepared from TMBA with six aromatic diamines by a direct polycondensation reaction using triphenyl phosphite and pyridine. The PAIs were soluble in polar aprotic solvents. They were able to form dense films with good mechanical properties and they present high thermal stability and LOI. They have pure gas permeability coefficient one order of magnitude larger than those of commercial poly(amide-imide) Torlon. TMBA-6FDA films show both higher pure gas permeability coefficients and higher ideal selectivity for the gas pair CO2/CH4 in the evaluated membranes; thus, this particular poly(amide-imide), TMBA-6FDA, shows a potential for application as a membrane for gas separation processes.
Conflicts of interest
There are no conflicts to declare.
Acknowledgements
The authors thankfully acknowledge the financial support from CONACYT-México grant number 206998. R. J. Canto-Acosta thanks CONACYT-México for the fellowship 576647. The authors thank Dr Patricia Quintana for the access to LANNBIO-CINVESTAV to carry out NMR measurements, and M. Sc. Rosalba Gamboa Magaña for her technical support in the FTIR analysis.
References
- J. M. Dodda and P. Bêlský, Eur. Polym. J., 2016, 84, 514 CrossRef CAS.
- E. Ravve, Principles of polymer chemistry, Springer, New York, 3rd edn, 2012 Search PubMed.
- P. S. Patil, R. R. Pal, M. M. Salunkhe, N. N. Maldar and P. P. Wadgaonkar, Eur. Polym. J., 2007, 43, 5047 CrossRef CAS.
- I. Bacosca, E. Hamciuc, M. Bruma and M. Ignat, React. Funct. Polym., 2011, 71, 905 CrossRef CAS.
- H. Behniafar and S. Mohammadparast-delshaad, Polym. Degrad. Stabil., 2012, 97, 228 CrossRef CAS.
- S.-H. Hsiao, W. Guo, T.-H. Tsai and Y.-T. Chiu, J. Polym. Res., 2014, 21, 391 CrossRef.
- Z. Rafiee, J. Macromol. Sci., Part B: Phys., 2015, 54, 749 CrossRef CAS.
- J. Feng, F. He, Z. Yang and J. Yao, Polym. Degrad. Stabil., 2016, 129, 231 CrossRef CAS.
- A. Sarkar, A. S. More, P. P. Wadgaonkar, G. J. Shin and J. C. Jung, J. Appl. Polym. Sci., 2007, 105, 1793 CrossRef CAS.
- C.-P. Yang, S.-H. Hsiao and H.-C. Hsiao, J. Polym. Sci., Part A: Polym. Chem., 1999, 37, 69 CrossRef CAS.
- S.-H. Hsiao, C.-Y. Teng and Y.-R. Kung, RSC Adv., 2015, 5, 93591 RSC.
- L. Cheng and X. G. Jian, J. Appl. Polym. Sci., 2004, 92, 1516 CrossRef CAS.
- H. B. Park, S. H. Han, C. H. Jung, Y. M. Lee and A. J. Hill, J. Membr. Sci., 2010, 359, 11 CrossRef CAS.
- S. H. Han, N. Misdan, S. Kim, C. M. Doherty, A. J. Hill and Y. M. Lee, Macromolecules, 2010, 43, 7657 CrossRef CAS.
- R. Guo, D. F. Sanders, Z. P. Smith, B. D. Freeman, D. R. Paul and J. E. McGrath, J. Mater. Chem., 2013, 1, 6063 RSC.
- N. Yamazaki, F. Higashi and J. Kawabata, J. Polym. Sci., Polym. Chem. Ed., 1974, 12, 2149 CrossRef CAS.
- S. H. Hsiao, C.-P. Yang, C.-W. Chen and G. S. Liou, J. Polym. Res., 2005, 12, 289 CrossRef CAS.
- L. H. Tagle, C. A. Terraza, A. Tundidor-Camba and F. A. Lara, Polym. Bull., 2014, 71, 1031 CrossRef CAS.
- C. Hamciuc, E. Hamciuc, M. Homocianu, A. Nicolescu and I.-D. Carja, Dyes Pigm., 2015, 114, 110 CrossRef CAS.
- P. K. Gutch, L. K. Pandey and C. Saxena, J. Appl. Polym. Sci., 2008, 110, 203 CrossRef CAS.
- D. W. van Krevelen and K. Ten Nijenhius, Properties polymer, completely rev., Amsterdam, 4th edn, 2009 Search PubMed.
- K. H. Park, M.-A. Kakimoto and Y. Imai, J. Polym. Sci., Part A: Polym. Chem., 1998, 36, 1987 CrossRef CAS.
- J.-H. Chang, K. M. Park, S.-O. Lee and J. B. Oh, J. Polym. Sci., Part A: Polym. Chem., 2000, 38, 2537 CrossRef CAS.
- B. Jarzabek, M. Wójtowicz and A. Wolinska-Grabczyck, Macromol. Chem. Phys., 2015, 216, 2377 CrossRef CAS.
- R. Balasubramanian, K. Kumutha and M. Sarojadevi, Polym. Bull., 2016, 73, 309 CrossRef CAS.
- S. Mondal and N. Das, RSC Adv., 2014, 4, 61383 RSC.
- R. N. Baker, Ind. Eng. Chem. Res., 2002, 41, 139 CrossRef.
- D. F. Sanders, Z. P. Smith, R. Guo, L. M. Robeson, J. M. McGrath, D. R. Paul and B. D. Freeman, Polymer, 2013, 54, 4729 CrossRef CAS.
- J. K. Adewole and A. L. Ahmad, J. Polym. Res., 2017, 24, 70 CrossRef.
- D. Fritsch and K.-V. Peinemann, J. Membr. Sci., 1995, 99, 29 CrossRef CAS.
- Z.-K. Xu, M. Böhning and J. Springer, Polymer, 1997, 38, 581 CrossRef CAS.
- S.-H. Huang, C.-C. Hu, K.-R. Lee, D.-J. Liaw and J.-Y. Lai, Eur. Polym. J., 2006, 42, 140 CrossRef CAS.
- W. F. Yong, F. Y. Li, T. S. Chung and Y. W. Tong, J. Membr. Sci., 2014, 462, 119 CrossRef CAS.
- A. Bondi, J. Phys. Chem., 1964, 68, 441 CrossRef CAS.
- J. Y. Park and D. R. Paul, J. Membr. Sci., 1997, 125, 23 CrossRef CAS.
Footnote |
† Electronic supplementary information (ESI) available. See DOI: 10.1039/c7ra11710h |
|
This journal is © The Royal Society of Chemistry 2018 |
Click here to see how this site uses Cookies. View our privacy policy here.