DOI:
10.1039/C7RA12412K
(Paper)
RSC Adv., 2018,
8, 74-79
A new and facile approach to 1,2-dihydroisoquinolin-3(4H)-imines by the Cu(I)-catalyzed reaction of 2-ethynylbenzyl methanesulfonates, sulfonyl azides and amines†
Received
14th November 2017
, Accepted 11th December 2017
First published on 19th December 2017
Abstract
A new, step-economical and operationally simple access to unsubstituted 1,2-dihydroisoquinolin-3(4H)-imines by Cu-catalyzed MCRs under mild conditions is described. In addition, selective hydrolysis of imines to the corresponding 1,2-dihydroisoquinolin-3(4H)-ones under refluxed conc. HCl has also been investigated.
Introduction
Heterocycle skeletons are popular scaffolds in various pharmaceuticals, biologically active compounds and functional materials.1 Tetrahydroisoquinoline (THIQ), an important nitrogen-containing heterocycle skeleton, is also found frequently in naturally existing alkaloids which have been shown to have a wide range of interesting biological activities such as antimuor, antimicrobial activities, and other pharmacological properties.2 Due to these advantages, great efforts have been made to develop various methodologies toward the synthesis of the THIQ skeleton and its derivatives. In general, traditional synthetic methods for the construction of this class of compounds are the Bischler–Napieralski cyclization/reduction,3 the Pictet–Spengler reaction,3a,4 the noble-transition metal-catalyzed asymmetric direct hydrogenation of isoquinoline or dihydroisoquinoline derivatives5 and some other elegant reactions.6 In recent years, in order to further expand the desirable molecular library of the THIQ alkaloids for the discovery of pharmaceutically active compounds, many excellent approaches by directly using THIQ precursors to their derivatives have also been developed. For example, currently, the most popular strategies to construct the THIQ skeleton-containing derivatives are the nucleophilic addition to the preformed C
N+ bond of cyclic precursors offered from the substrate of tetrahydroisoquinoline via the oxidative cross-dehydrogenative coupling (CDC) reaction7 or the redox-neutral reaction8 and those associated with free radical intermediates.9 Among the above-mentioned synthetic strategies, although all approaches can provide access to the THIQ skeleton and their derivatives, the synthetic pathways towards 1,2-dihydroisoquinolin-3(4H)-imines, which are a class of important members of THIQ family and have been wildly applied in material sciences and biological research,10 are rarely reported in the literature. A more careful literature survey indicates that only Soufiaoui et al. in 1992 described their synthesis via the 1,3-dipolar cycloaddition of arylazides with 1,2-dihydroisoquinoline derivatives followed by splitting decomposition11 under heating (Scheme 1A) and Chang's group in 2006 reported a catalytic one-pot approach to the title product using aminoalkyne and azide as substrate under mild condition12 (Scheme 1B). However, these methods to synthesize 1,2-dihydroisoquinolin-3(4H)-imine suffered from some disadvantages including multiple reaction steps,11 harsh reaction conditions,11 few scope of substrate (one example only!)12 and the use of noble metal.12 Therefore, the search for a step-economical, more convenient and efficient catalytic system with attractive features such as easily accessible starting materials, mild reaction conditions, and nontoxic side products to generate structural diversity of 1,2-dihydroisoquinolin-3(4H)-imine is of great value.
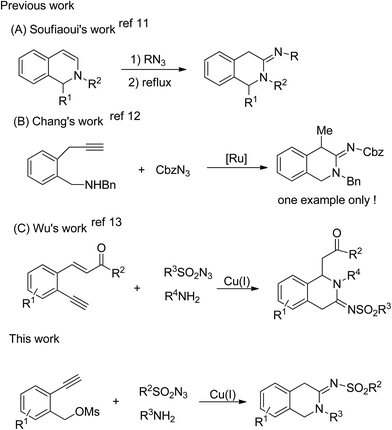 |
| Scheme 1 Different strategies for the construction of 1,2-dihydroisoquinolin-3(4H)-imine. | |
As an ideal synthetic tool, multicomponent reactions (MCRs) involving a tandem reaction process with three or more reagents in a single process under mild conditions have emerged as a powerful and attractive strategy for the synthesis of structurally diverse organic molecules. Recently, the methodology is also applied in the synthesis of 1,2-dihydroisoquinolin-3(4H)-imines. For instance, Wu's group in 2011 presented one type of 1,2-dihydroisoquinolin-3(4H)-imines via MCRs using 2-ethynylphenyl-chalcones, sulfonyl azides and amines as substrates under Cu(I) catalyst (Scheme 1C),13 in which the strategy may experience the reaction process with the active ketenimine intermediate investigated widely by the pioneers.14 It is well known that the pharmacological and biological activity of molecule is shown to be dependent on the nature of substituents. However, most MCRs follow a single reaction route to afford only one substituted type of molecular skeleton and their derivatives. Therefore, development of novel MCRs for the selective formation of diverse substituted molecules containing the same molecular skeleton is a worthy and challenging goal. As a continuation of our interest in ketenimine chemistry and THIQ skeleton, we herein report a convenient and efficient synthetic strategy for the synthesis of 1,2-dihydroisoquinolin-3(4H)-imines via novel MCRs from 2-ethynylbenzyl methanesulfonates, sulfonyl azides and amines (Scheme 1, this work).
Results and discussion
Our studies started with the synthesis of variously substituted 2-ethynylbenzyl methanesulfonates 4 from substituted 2-bromobenzaldehyde 1 according to the literature procedures15 as indicated in Scheme 2.
 |
| Scheme 2 Facile synthesis of 2-ethynylbenzyl methanesulfonates 4. | |
And then, under N2 and sealed tube, we carried out a model MCRs of 2-ethynylbenzyl methanesulfonate 4a with TsN3 5a and phenylamine 6a in dichloromethane at room temperature in the presence of CuI and Et3N for 12 h (Table 1). To our delight, the desired product (entry 1), 2-dihydroisoquinolin-3(4H)-imine of 7a was isolated in 40% yield, and its structure was further conformed by X-ray crystallographic analysis (showed in Table 1). Inspired by the result of reaction, we continue to optimize the reaction condition of model reaction to improve the yield of 7a to the highest. The results for various optimization experiments are summarized in Table 1. Subsequently, other copper salt were then screened under the reaction condition just mentioned. The results indicated that, in comparison to the potential copper catalyst, only CuBr was proven to be more effective one (54%), whereas Cu2O even can not lead to the formation of 7a (entries 1–4). When the reaction temperature under CuBr as catalyst was gradually increased from rt to 60 °C, the yield of 7a increases to 77%. However, it was found that further elevating the reaction temperature, in contrast, made the yield decrease (entries 5–8). We next examined the effect of solvent in the MCRs. The results revealed that dichloromethane (77%) was the most suitable solvent for the reaction (entry 7, entries 9–13). As showed in Table 1, different base also affected the final desired outcome. The reaction was carried out efficiently when triethylamine acted as the base, furnishing the desired product in 77% yield, and the corresponding product was not almost afforded in the presence of K2CO3 or DBU (entry 7, entries 14–17). Additionally, it should be noted that the reaction time also played the important role in improving the yield of target product. The experimental results indicated the shorter reaction time would be beneficial to the formation of 7a (entry 7, entries 18–19). Hence, after screening the reaction condition of MCRs, the optimized conditions to selectively obtain the desired product 7a, under N2 and sealed tube, are identified as the employment of Et3N as base and CuBr as catalyst in dichloromethane for 0.5 h at 60 °C.
Table 1 Optimization of the reaction conditionsa

|
Entry |
Catalyst |
Base |
Solvent |
Temp. (°C) |
Yieldb (%) |
Reaction conditions: toluenesulfonyl azide (0.36 mmol), 2-ethynylbenzyl methanesulfonate (0.3 mmol), phenylamine (0.36 mmol), base (0.45 mmol), catalyst (0.03 mmol),solvent (1 mL). Isolated yields. nd = not detected. Reaction carried out for 12 h. Reaction carried out for 0.5 h. Reaction carried out for 1 h. |
1c |
CuI |
Et3N |
DCM |
rt |
40 |
2 |
Cu2O |
Et3N |
DCM |
rt |
nd |
3 |
CuCl |
Et3N |
DCM |
rt |
47 |
4 |
CuBr |
Et3N |
DCM |
rt |
54 |
5 |
CuBr |
Et3N |
DCM |
40 |
57 |
6 |
CuBr |
Et3N |
DCM |
50 |
60 |
7 |
CuBr |
Et3N |
DCM |
60 |
77 |
8 |
CuBr |
Et3N |
DCM |
70 |
50 |
9 |
CuBr |
Et3N |
CHCl3 |
60 |
64 |
10 |
CuBr |
Et3N |
MeCN |
60 |
35 |
11 |
CuBr |
Et3N |
THF |
60 |
67 |
12 |
CuBr |
Et3N |
Dioxane |
60 |
50 |
13 |
CuBr |
Et3N |
DMF |
60 |
35 |
14 |
CuBr |
K2CO3 |
DCM |
60 |
Trace |
15 |
CuBr |
(iPr2)NEt |
DCM |
60 |
70 |
16 |
CuBr |
DBU |
DCM |
60 |
nd |
17 |
CuBr |
DABCO |
DCM |
60 |
37 |
18d |
CuBr |
Et3N |
DCM |
60 |
82 |
19e |
CuBr |
Et3N |
DCM |
60 |
80 |
With the optimized reaction conditions secured, we subsequently applied the optimized conditions for the formation of 7a to explore the scope and generality of the MCRs. The results in this section, as summarized in Table 2, demonstrated that the present method is generally applicable in the formation of target products depending on the employment of the corresponding starting materials. It was found that all aromatic substrates bearing various substutution such as electron donating and withdrawing group underwent smoothly this transformation generating the corresponding products 7 with moderate to excellent yields. However, we also found that in addition to the substrates with withdrawing group such as F at the para position of phenyl ring giving the lower yields (51% in both) (entries 16, 31), the property of other substituents on phenyl ring showed no obvious impact on the yield of corresponding products. Intriguingly, when the substrates are aliphatic amines, the methodology only furnish either the low corresponding yield (entry 9, 35%) or no desired product (entry 10, 0%). The result may be caused by the fact that in contrast to aromatic amines, aliphatic amines with stronger nucleophilic have higher activity and lower selectivity. Therefore, when aliphatic amines reacted with other substrates, the product in a low or no yield will be achieved.
Table 2 Substrate scope of MCRs for the formation of 7a

|
Entry |
R1 |
R2 |
R3 |
Product |
Yieldb [%] |
Optimal reaction conditions: 4 (0.2 mmol), CuBr (0.02 mmol), Et3N (0.3 mmol) in DCM (1 mL), sealed and then heated at 60 °C for 0.5 h under N2. Isolated yields are given. |
1 |
H(4a) |
4-CH3C6H4(5a) |
C6H5(6a) |
7a |
82 |
2 |
4a |
5a |
4-CH3C6H4(6b) |
7b |
78 |
3 |
4a |
5a |
4-FC6H4(6c) |
7c |
84 |
4 |
4a |
5a |
4-ClC6H4(6d) |
7d |
77 |
5 |
4a |
5a |
2-CH3OC6H4(6e) |
7e |
68 |
6 |
4a |
5a |
2-FC6H4(6f) |
7f |
76 |
7 |
4a |
5a |
2,6-EtC6H3(6g) |
7g |
65 |
8 |
4a |
5a |
2,4,6-CH3C6H2(6h) |
7h |
74 |
9 |
4a |
5a |
Bn(6i) |
7i |
35 |
10 |
4a |
5a |
tBu(6j) |
7j |
0 |
11 |
4a |
C6H5(5b) |
6a |
7k |
80 |
12 |
4a |
5b |
6e |
7l |
78 |
13 |
4a |
5b |
6h |
7m |
74 |
14 |
4a |
2,4,6-CH3C6H2(5c) |
6a |
7n |
85 |
15 |
4a |
5c |
6c |
7o |
84 |
16 |
4-F(4b) |
5a |
6a |
7p |
51 |
17 |
4b |
5a |
6c |
7q |
83 |
18 |
5-F(4c) |
5a |
6a |
7r |
89 |
19 |
4c |
5a |
6b |
7s |
77 |
20 |
4c |
5a |
6c |
7t |
84 |
21 |
4c |
5b |
6c |
7u |
81 |
22 |
4c |
5c |
6a |
7v |
80 |
23 |
6-F(4d) |
5a |
6a |
7w |
75 |
24 |
4d |
5a |
6c |
7x |
88 |
25 |
4d |
5b |
6a |
7y |
71 |
26 |
4-Cl(4e) |
5a |
6a |
7z |
77 |
27 |
4e |
5a |
6c |
7aa |
87 |
28 |
4e |
5b |
6c |
7ab |
72 |
29 |
4-CH3(4f) |
5a |
6a |
7ac |
77 |
30 |
4f |
5a |
6b |
7ad |
73 |
31 |
4f |
5a |
6c |
7ae |
51 |
Based on the above results and the chemistry related to ketenimine, a plausible mechanism of this MCRs is proposed and shown in Scheme 3. Taking the formation of 7a as an example, first, the ketenimine intermediate A is formed via the reaction of 2-ethynylbenzyl methanesulfonate (4a) with TsN3 under the optimized conditions. Subsequently, when the anilines added into the reaction, the double nucleophilic addition would happen to afford the intermediate B. And then, intermediate B will tautomerize into the intermediate C. Finally, the intermediate C, which undergo sequential proton transfer, will transform into the desired product 7a.
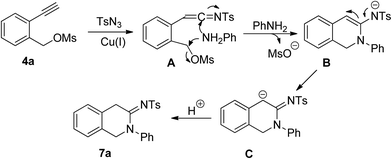 |
| Scheme 3 Possible reaction mechanism for the formation of 7a. | |
Subsequently, to further take advantage of the synthetic application of this methodology, we then explored the synthesis of 1,2-dihydroisoquinolin-3(4H)-ones 8 by hydrolysis of the resulting products 7. To the best of our knowledge, 8 were considered as important building block in organic synthesis16 and have good physiological activities in medicinal chemistry. For example, more recently, the researchers found that the compounds containing the skeleton of 1,2-dihydroisoquinolin-3(4H)-one were acetylcholinesterase and β-secretase dual inhibitors in treatment with alzheimer's disease.17 Therefore, we proceeded to treat the dihydroisoquinolin-3(4H)-imines 7 with concentrated HCl under reflux for 12 h. To our delight, the corresponding title products 8 were achieved in good yields (Table 3). The results indicate that 7 can be considered as a class of important precursor of 1,2-dihydroisoquinolin-3(4H)-ones 8.
Table 3 Hydrolysis of imines 7 to 1,2-dihydroisoquinolin-3(4H)-ones 8a
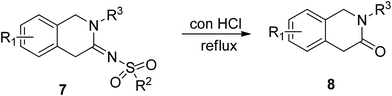
|
Entry |
R1 |
R2 |
R3 |
Product |
Yieldb [%] |
Reaction conditions: 7 (0.5 mmol), conc HCl (10 mL), reactions refluxed for 12 h. Isolated yields. |
1 |
H(a) |
4-CH3C6H4(b) |
C6H5 |
8a |
66 |
2 |
a |
b |
4-CH3C6H4 |
8b |
74 |
3 |
a |
b |
4-FC6H4 |
8c |
59 |
4 |
a |
b |
4-ClC6H4 |
8d |
69 |
In summary, a new and facile MCRs for the formation of unsubstituted 1,2-dihydroisoquinolin-3(4H)-imines in good yields has been developed under mild condition. The experimental results indicated that various aromatic substrates could be well tolerated in this reaction. Meanwhile, selective hydrolysis of imines to the corresponding 1,2-dihydroisoquinolin-3(4H)-ones has also been investigated under refluxed conc. HCl. In addition, some 2-ethynylbenzyl methanesulfonates that had not previously been reported were also synthesized and characterized. The methodology provides a step-economical and operationally simple access to functionalized desired product and can further enrich the compound library of 1,2-dihydroisoquinolin-3(4H)-imine.
Conflicts of interest
There are no conflicts to declare.
Acknowledgements
We are grateful for financial support by the Science Foundation of Shanghai Institute of Technology (grant number YJ2013-61).
Notes and references
-
(a) L. F. Tietze and A. Modi, Med. Res. Rev., 2000, 20, 304–322 CrossRef CAS PubMed
;
(b) B. B. Touré and D. G. Hall, Chem. Rev., 2009, 109, 4439–4486 CrossRef PubMed
;
(c) S. Yu and S. Ma, Angew. Chem., Int. Ed., 2012, 51, 3074–3112 CrossRef CAS PubMed
;
(d) K. S. Gudmundsson and B. A. Johns, Org. Lett., 2003, 5, 1369–1372 CrossRef CAS PubMed
;
(e) C. Hamdouchi, B. Zhong, J. Mendoza, E. Collins, C. Jaramillo, J. Diego, D. Robertson, C. D. Spencer, B. D. Anderson, S. A. Watkins, F. Zhang and H. B. Brooks, Bioorg. Med. Chem. Lett., 2005, 15, 1943–1947 CrossRef CAS PubMed
. - For selected examples:
(a) K. W. Bentley, The Isoquinoline Alkaloids, Hardwood Academic, Amsterdam, 1998, vol. 1 Search PubMed
;
(b) J. D. Phillipson, M. F. Roberts and M. H. Zenk, The Chemistry and Biology of Isoquinoline Alkaloids, Springer Verlag, Berlin, 1985 Search PubMed
;
(c) M. D. Rozwadowska, Heterocycles, 1994, 39, 903–931 CrossRef CAS
;
(d) D. Jack and R. Williams, Chem. Rev., 2002, 102, 1669–1730 CrossRef
;
(e) M. Chrzanowska and M. D. Rozwadowska, Chem. Rev., 2004, 104, 3341–3370 CrossRef CAS PubMed
;
(f) P. Siengalewicz, U. Rinner and J. Mulzer, Chem. Soc. Rev., 2008, 37, 2676–2696 RSC
;
(g) B. Zhou, J. Guo and S. Danishefsky, Org. Lett., 2002, 4, 43–46 CrossRef CAS PubMed
;
(h) A. Endo, A. Yanagisawa, M. Abe, S. Tohma, T. Kan and T. Fukuyama, J. Am. Chem. Soc., 2002, 124, 6552–6554 CrossRef CAS PubMed
;
(i) K. W. Bentley, Nat. Prod. Rep., 2006, 23, 444–463 RSC
;
(j) X. Fang, Y. Yin, Y. Chen, L. Yao, B. Wang, M. Caneron, L. Lin, S. Khan, P. LoGrasso and Y. Feng, J. Med. Chem., 2010, 53, 5727–5737 CrossRef CAS PubMed
;
(k) C. Razafindrabe, S. Aubry, B. Bourdon, M. Andriantsiferana, S. Pellet-Rostaing and M. Lemaire, Tetrahedron, 2010, 66, 9061–9066 CrossRef CAS
. -
(a) M. Chrzanowska and M. D. Rozwadowska, Chem. Rev., 2004, 104, 3341–3370 CrossRef CAS PubMed
;
(b) B. S. Thyagarajan, Chem. Rev., 1954, 54, 1019–1064 CrossRef CAS
;
(c) J. Gal, R. J. Wienkam and N. Castagnoli, J. Org. Chem., 1974, 39, 418–421 CrossRef CAS
. - J. Stökigt, A. P. Antonchick, F. Wu and H. Waldmann, Angew. Chem., 2011, 123, 8692–8696 (Angew. Chem., Int. Ed., 2011, 50, 8538–8564) CrossRef
. - For selected examples of the asymmetric hydrogenation of isoquinolines, see:
(a) Y. G. Zhou, Acc. Chem. Res., 2007, 40, 1357 CrossRef CAS PubMed
;
(b) D. S. Wang, Q. A. Chen, S. M. Lu and Y. G. Zhou, Chem. Rev., 2012, 112, 2557–2590 CrossRef CAS PubMed
;
(c) R. Noyori, M. Ohta, Y. Hsiao, M. Kitamura, T. Ohta and H. Takaya, J. Am. Chem. Soc., 1986, 108, 7117–7119 CrossRef CAS
;
(d) N. Uematsu, A. Fujii, S. Hashiguchi, T. Ikariya and R. Noyori, J. Am. Chem. Soc., 1996, 118, 4916–4917 CrossRef CAS
;
(e) S. M. Lu, Y. Q. Wang, X. W. Han and Y. G. Zhou, Angew. Chem., 2006, 118, 2318–2321 (Angew. Chem., Int. Ed., 2006, 45, 2260–2263) CrossRef
;
(f) C. Q. Li and J. L. Xiao, J. Am. Chem. Soc., 2008, 130, 13208–13209 CrossRef CAS PubMed
;
(g) P. C. Yan, J. H. Xie, G. H. Hou, L. X. Wang and Q. L. Zhou, Adv. Synth. Catal., 2009, 351, 3243–3250 CrossRef CAS
;
(h) L. E. Vanno, J. Ormala and P. M. Pihko, Chem.–Eur. J., 2009, 15, 12963–12967 CrossRef PubMed
;
(i) M. X. Chang, W. Li and X. M. Zhang, Angew. Chem., 2011, 123, 10867–10870 (Angew.Chem., Int. Ed., 2011, 50, 10679–10681) CrossRef
;
(j) F. Berhal, Z. Wu, Z. G. Zhang, T. Ayad and V. Ratovelomanana-Vidal, Org. Lett., 2012, 14, 3308–3383 CrossRef CAS PubMed
;
(k) A. Iimuro, K. Yamaji, S. Kandula, T. Nagano, Y. Kita and K. Mashima, Angew. Chem., 2013, 125, 2100–2104 (Angew. Chem., Int. Ed., 2013, 52, 2046–2050) CrossRef
;
(l) Z. Wu, M. Perez, M. Scalone, T. Ayad and V. Ratovelomanana-Vidal, Angew. Chem., 2013, 125, 5025–5028 (Angew. Chem., Int. Ed., 2013, 52, 4925–4928) CrossRef
. - For other selected examples for the synthesis of THIQ skeleton and their derivatives:
(a) A. Padwa, M. D. Danca, K. Hardcastle and M. McClure, J. Org. Chem., 2003, 68, 929–941 CrossRef CAS PubMed
;
(b) A. Padwa and M. D. Danca, Org. Lett., 2002, 4, 715–717 CrossRef CAS PubMed
;
(c) E. Garcia, E. Lete and N. Sotomayor, J. Org. Chem., 2006, 71, 6776–6784 CrossRef CAS PubMed
;
(d) K. Shimizu, M. Takimoto and M. Mori, Org. Lett., 2003, 5, 2323–2325 CrossRef CAS PubMed
;
(e) L. Evanno, J. Ormala and P. Pihko, Chem.–Eur. J., 2009, 15, 12963–12967 CrossRef CAS PubMed
. - For selected examples of oxidative cross-dehydrogenative coupling:
(a) X. H. Liu, J. L. Zhang, S. X. Ma, Y. X. Ma and R. Wang, Chem. Commun., 2014, 50, 15714–15717 RSC
;
(b) S. Murarka, I. Deb, C. Zhang and D. Seidel, J. Am. Chem. Soc., 2009, 131, 13226–13227 CrossRef CAS PubMed
;
(c) J. Xie, H. M. Li, J. C. Zhou, Y. X. Cheng and C. Zhu, J. Angew. Chem., 2012, 124, 1278–1281 (Angew. Chem., Int. Ed., 2012, 51, 1252–1255) CrossRef
;
(d) J. M. Zhang, B. Tiwari, C. Xing, X. K. Chen and Y. R. Chi, Angew. Chem., 2012, 124, 3709–3712 (Angew. Chem., Int. Ed., 2012, 51, 3649–3652) CrossRef
;
(e) G. Zhang, Y. X. Ma, S. L. Wang, Y. H. Zhang and R. Wang, J. Am. Chem. Soc., 2012, 134, 12334–12337 CrossRef CAS PubMed
;
(f) A. J. Neel, J. P. Hehn, P. F. Tripet and F. D. Toste, J. Am. Chem. Soc., 2013, 135, 14044–14047 CrossRef CAS PubMed
;
(g) H. Ueda, K. Yoshida and H. Tokuyama, Org. Lett., 2014, 16, 4194–4197 CrossRef CAS PubMed
;
(h) G. Zhang, Y. X. Ma, G. B. Cheng, D. B. Liu and R. Wang, Org. Lett., 2014, 16, 656–659 CrossRef CAS PubMed
;
(i) H. M. Huang, Y. J. Li, Q. Ye, W. B. Yu, L. Han, J. H. Jia and J. R. Gao, J. Org. Chem., 2014, 79, 1084–1092 CrossRef CAS PubMed
. - For selected examples of redox-neutral reaction:
(a) W. L. Lin, T. Cao, W. Fan, Y. L. Han, J. Q. Kuang, H. W. Luo, B. K. Y. Miao, X. J. Tang, Q. Yu, W. M. Yuan, J. S. Zhang, C. Zhu and S. M. Ma, Angew. Chem., Int. Ed., 2014, 53, 277–281 CrossRef CAS PubMed
;
(b) Q. H. Zheng, W. Meng, G. J. Jiang and Z. X. Yu, Org. Lett., 2013, 15, 5928–5931 CrossRef CAS PubMed
;
(c) M. T. Richers, M. Breugst, A. Y. Platonova, A. Ullrich, A. Dieckmann, K. N. Houk and D. Seidel, J. Am. Chem. Soc., 2014, 136, 6123–6135 CrossRef CAS PubMed
;
(d) C. L. Jarvis, M. T. Richers, M. Breugst, K. N. Houk and D. Seidel, Org. Lett., 2014, 16, 3556–3559 CrossRef CAS PubMed
;
(e) Z. B. Zhu and D. Seidel, Org. Lett., 2017, 19, 2841–2844 CrossRef CAS PubMed
;
(f) K. L. Zheng, M. Q. You, W. M. Shu, Y. D. Wu and A. X. Wu, Org. Lett., 2017, 19, 2262–2265 CrossRef CAS PubMed
. -
(a) W. T. Wei, X. J. Dong, S. Z. Nie, Y. Y. Chen, X. J. Zhang and M. Yan, Org. Lett., 2013, 15, 6018–6021 CrossRef CAS PubMed
;
(b) Y. Y. Chen, X. J. Zhang, H. M. Yuan, W. T. Wei and M. Yan, Chem. Commun., 2013, 49, 10974–10976 RSC
;
(c) J. Xuan, T. T. Zeng, J. Feng, Q. H. Deng, J. R. Chen, L. Q. Lu, W. J. Xiao and H. Alper, Angew. Chem., Int. Ed., 2015, 54, 1625–1628 CrossRef CAS PubMed
. - For selected examples:
(a) H. Li, J. L. Petersen and K. K. Wang, J. Org. Chem., 2003, 68, 5512–5518 CrossRef CAS PubMed
;
(b) J. Qian, X. Qian and Y. Xu, Chem.–Eur. J., 2009, 15, 319–323 CrossRef CAS PubMed
;
(c) M. Jaggi, C. Blum, N. Dupont, J. Grilj, S. X. Liu, J. Hauser, A. Hauser and S. Decurtins, Org. Lett., 2009, 11, 3096–3099 CrossRef CAS PubMed
;
(d) H. Langhals and T. Pust, Eur. J. Org. Chem., 2010, 3140–3145 CrossRef CAS
. - S. Kitane, L. Chraibi and M. Soufiaoui, Tetrahedron, 1992, 48, 8935–8942 CrossRef CAS
. - S. Chang, M. J. Lee, D. Y. Jung, E. J. Yoo, S. H. Cho and S. K. Han, J. Am. Chem. Soc., 2006, 128, 12366–12367 CrossRef CAS PubMed
. - Z. Y. Chen, C. Ye, L. Gao and J. Wu, Chem. Commun., 2011, 47, 5623–5625 RSC
. - For selected examples for ketenimine chemistry:
(a) E. J. Yoo, I. Bae, S. H. Cho, H. Han and S. Chang, Org. Lett., 2006, 8, 1347–1350 CrossRef CAS PubMed
;
(b) S. H. Cho and S. Chang, Angew. Chem., Int. Ed., 2007, 46, 1897–1900 CrossRef CAS PubMed
;
(c) S. H. Cho and S. Chang, Angew. Chem., Int. Ed., 2008, 47, 2836–2839 CrossRef CAS PubMed
;
(d) E. J. Yoo, S. H. Park, S. H. Lee and S. Chang, Org. Lett., 2009, 11, 1155–1158 CrossRef CAS PubMed
;
(e) I. Bae, H. Han and S. Chang, J. Am. Chem. Soc., 2005, 127, 2038–2039 CrossRef CAS PubMed
;
(f) Y. Shen, S. Cui, J. Wang, X. Chen, P. Lu and Y. G. Wang, Adv. Synth. Catal., 2010, 352, 1139–1144 CrossRef CAS
;
(g) W. Yao, L. Pan, Y. Zhang, G. Wang, X. Wang and C. Ma, Angew. Chem., Int. Ed., 2010, 49, 9210–9214 CrossRef CAS PubMed
;
(h) Y. Shang, K. Ju, X. He, J. Hu, S. Yu, M. Zhang, K. Liao, L. Wang and P. Zhang, J. Org. Chem., 2010, 75, 5743–5745 CrossRef CAS PubMed
;
(i) I. Cano, E. Alvarez, M. C. Nicasio and P. J. Pérez, J. Am. Chem. Soc., 2011, 133, 191–193 CrossRef CAS PubMed
;
(j) Y. Shang, X. He, J. Hu, J. Wu, M. Zhang, S. Yu and Q. Zhang, Adv. Synth. Catal., 2009, 351, 2709–2713 CrossRef CAS
;
(k) Z. Chen, D. Zheng and J. Wu, Org. Lett., 2011, 13, 848–851 CrossRef CAS PubMed
. -
(a) P. C. Too and S. Chiba, Chem. Commun., 2012, 48, 7634–7636 RSC
;
(b) P. M. Poladura, E. Rubio and J. M. Gonzlez, Angew. Chem., Int. Ed., 2015, 54, 3052–3055 CrossRef PubMed
;
(c) H. J. Lu, C. Q. Li, H. L. Jiang, C. L. Lizardi and X. P. Zhang, Angew. Chem., Int. Ed., 2014, 53, 7028–7032 CrossRef CAS PubMed
. -
(a) M. Hu, P. R. Guzzo, C. X. Zha, K. Nacro, Y. L. Yang, C. Hassler and S. Liu, Tetrahedron Lett., 2012, 53, 846–848 CrossRef CAS
;
(b) R. A. J. Mook, K. Lackey and C. Bennett, Tetrahedron Lett., 1995, 36, 3969–3972 CrossRef CAS
;
(c) A. Kamimura, Y. Ishihara, M. So and T. Hayashi, Tetrahedron Lett., 2009, 50, 1727–1730 CrossRef CAS
. - Y. C. Deng, Y. R. Jiang, X. J. Zhao and J. L. Wang, J. Biomed. Sci., 2016, 4, 112–113 Search PubMed
.
Footnote |
† Electronic supplementary information (ESI) available. CCDC 1574819. For ESI and crystallographic data in CIF or other electronic format see DOI: 10.1039/c7ra12412k |
|
This journal is © The Royal Society of Chemistry 2018 |
Click here to see how this site uses Cookies. View our privacy policy here.