DOI:
10.1039/C7RA13074K
(Paper)
RSC Adv., 2018,
8, 5106-5113
An efficient mercapto-functionalized organic–inorganic hybrid sorbent for selective separation and preconcentration of antimony(III) in water samples
Received
6th December 2017
, Accepted 22nd January 2018
First published on 29th January 2018
Abstract
This work reported on the application of mercapto-functionalized silica-supported organic–inorganic hybrid sorbent as a solid phase extraction (SPE) extractant for effective separation and preconcentration of Sb(III) species in real water samples. The influences of pH, sorbent amounts, flow rates and the concentration of eluent on the adsorption and desorption of Sb(III) species had been evaluated. The recovery of Sb(III) species at pH 5 with 100 mg mercapto-functionalized hybrid sorbent at the flow rate of 5.0 mL min−1 was greater than 95% without interference from all of metal ions tested. The trapped Sb(III) species by extractant was then eluted with 5% HCl solution at the flow rate of 5.0 mL min−1. The proposed procedure permitted large enrichment factors of about 200 and higher for 10 μg L−1 of Sb(III) species. The merits of analytical figures for the determination of Sb(III) species were as follows: detection limit (3σ, n = 11), 2 ng L−1; precision, 1.6% (n = 11) for 10 μg L−1 of Sb(III) species; the linear calibration curve presented in the concentration range of 1.0–200.0 μg L−1. The validity of the proposed procedure was checked by the analysis of standard reference materials. Excellent agreement between the analytical results and the certified values (t-test at 95% confidence level) was found. The mercapto-functionalized hybrid sorbent as a SPE extractant was applied to the determination of Sb(III) species in various water samples with satisfactory results.
1. Introduction
Antimony (Sb) is increasingly considered to be a toxic heavy metal with possible carcinogenic effects on human health.1 Antimony contamination is receiving concerns with increasing knowledge on its environmental fates and toxicological characteristics.2 Antimony exists primarily as Sb(V) in oxic systems and Sb(III) in anoxic systems.3 Sb(III) compounds have ten times higher acute toxicity than Sb(V) species.4 To understand the bioavailability and toxicity of Sb, there is a need for reliable methods that enable the measurements of specific speciation of Sb, especially for Sb(III) species.
Atomic fluorescence spectrometry is a simple and well available technique for the determination of Sb in real samples.5 However, its main problem is the low selectivity for the speciation of Sb. Separation and preconcentration procedures can be applied to overcome this limitation. Solid-phase extraction (SPE) is one of widely used and fast emerging techniques for the separation and preconcentration of heavy metals due to its high enrichment factors, minimal costs, environmental friendliness, no need for emulsion, simplicity, flexibility, safety and ease of automation.6 Up to now, several kinds of SPE extractants, such as silica-based octyl sorbent,7 multi-walled carbon nanotubes,8 octyl-immobilized silica-coated magnetic nanoparticles,9 nano γ-alumina,10 single-walled carbon11 and functionalized mesoporous silica,12 have been used to selectively retain the Sb(III) complex with ammonium pyrrolidine dithiocarbamate. Li et al.9 reported that Sb(III) forms a hydrophobic complex with ammonium pyrrolidine dithiocarbamate at pH 5.0 and is retained on SPE extractant, whereas Sb(V) remain as the free species in aqueous solution. However, a few SPE extractants based on the direct retention of Sb(III) species have been developed. Zih-Perényi et al.13 used imino diacetic acid–ethyl cellulose for the selective separation and preconcentration of Sb(III). SiO2 nanoparticles functionalised with 1-(2-pyridylazo)-2-naphthol was used as SPE extractant for the preconcentration of trace amounts of Sb(III) in different water samples by Kaur and Gupta.14 Silica gel functionalized with 1,5-bis(di-2-pyridyl) methylene thiocarbonohydrazide was developed for the simultaneous separation, preconcentration and determination of antimony.15 Silica gel is used as a solid support for chelating groups because it is an inorganic material, stable under acidic conditions, nonswelling, high mass exchange characteristics and thermal resistance,16 and relatively easy to be grafted with organosilanes containing functional groups by the co-condensation or self-condensation reactions.17 In our previous work, the immobilization of mercapto groups onto the surface of silica gel could be used for the selective removal of Sb(III) from aqueous solution due to the well-known phenomenon of hard-soft acid-bases theory.18 However, the simultaneous separation, preconcentration and determination of Sb(III) species in water samples using mercapto-functionalized hybrid sorbents as the SPE extractant has not been reported.
The purpose of this work is to establish a new SPE method using mercapto-functionalized hybrid sorbents as the extractant for the separation, preconcentration and determination of Sb(III) in water samples prior to atomic fluorescence spectrometry measurement. The influences of experimental conditions (such as pH, the flow rates of sample and eluent, and the concentration and volume of eluent solution) on the quantitative recoveries of Sb(III), have been investigated to establish an optimized SPE procedure. The validity of the proposed procedure has been evaluated by the analysis of certified reference materials. The separation, preconcentration and determination of Sb(III) species from real samples were also tested.
2. Experimental
2.1 Chemicals
The stock solutions of Sb(III) (1000 mg L−1) and Sb(V) (1000 mg L−1) were prepared by potassium antimonyl tartrate and potassium hexahydroxyantimonate (Sigma-Aldrich), respectively. The working solutions were prepared by series dilution of the stock solutions immediately prior to their use. The other chemicals were obtained from Sinopharm Chemical Reagent Co., Ltd., Shanghai, China. All chemicals used in this work were of analytical grade and used without further purification. All the metal stock solutions (1000 mg L−1) were obtained from the National Research Center for Standard Materials (NRCSM, Beijing, China). The solution of sodium tetrahydroborate 0.7% was prepared by dissolving 7 g of NaBH4 powder and 4 g of NaOH in 1000 mL of water. A stock solution of potassium iodide 50% (w/v) containing L-ascorbic acid 10% (w/v) was prepared by dissolving 25 g of KI and 5 g of L-ascorbic acid in a 50 mL of water. 8-Hydroxyquinoline stock solution 1% (w/v) was prepared by a dissolving 1 g of 8-hydroxyquinoline was in a 10 mL of methanol, and then diluted with water to make 100 mL. All experimental and reagent solutions were prepared with deionized water. The solutions were prepared weekly and stored in a refrigerator. In order to prevent metal contamination, all the glassware was kept overnight in a 10% (v/v) HNO3 solution with posterior cleaning with deionized water prior to use.
2.2 Apparatus
Fourier transmission infrared (FT-IR) spectra were recorded on a Spectrum One spectrometer (Perkin Elmer) with KBr pellets and a resolution of 1 cm−1. The samples were characterized by a scanning electron microscope (SEM) coupled to an energy dispersive spectrometer (EDS) with gold coating at the desired magnification (Shimadzu SSX-550). A commercial two channel hydride generation nondispersive atomic fluorescence spectrometer (HG-AFS, Model AFS-2202E, Beijing Haiguang Instrument Co., Beijing, China) equipped with a quartz argon–hydrogen flame atomizer, a quartz gas–liquid separator, and coded high intensity hollow cathode lamps of Sb were used for the measurement of Sb(III) and total Sb concentrations in solution according to the steps described by Deng et al.19
2.3 Synthesis of the sorbent
Mercapto-functionalized hybrid sorbent was prepared by a two-step acid–base catalyzed sol–gel process as previously reported in the literature.20 Mercapto-functionalized hybrid sorbent was prepared by the following process: tetraethoxysilane (TEOS) was mixed with an acidic aqueous solution by using TEOS and water at the volume ratio of 2
:
1 at pH 2 with the addition of 6 mol L−1 HCl solution. The mixture was stirred for 20 min at room temperature to initiate the hydrolysis reaction. Next, 30 wt% of ammonium hydroxide was poured into the mixture to induce the condensation reaction. The transparent solution became turbid, indicating that the colloidal particles were formed. The obtained sorbent was left for a night and rinsed with 60/40 (v/v) of ethanol/water for 12 h. The precipitate was filtered through a membrane filter and washed with deionized water. Thus, formed mercapto-functionalized hybrid sorbent was obtained after drying under vacuum at 60 °C for 16 h. The mercapto-functionalized hybrid sorbent was sieved to obtain the particle size between 100 and 200 meshes. The textural properties of mercapto-functionalized hybrid sorbent were characterized by FT-IR spectra and SEM-EDS.
2.4 Samples
100 mg of stream sediment certified reference material (GBW07309) was accurately weighed into a beaker and a mixture of concentrated hydrochloric acid (24 mL) and nitric acid (8.0 mL) was added. The beaker covered with a watch glass was evaporated on a hot plate almost to dryness. Then, 8.0 mL of mixed acid solution was added to the residue. After cooling, the mixture was filtered through a glass filter. The sample and 5 mL of 10% vitamin C solution was mixed, and then the mixture solution was diluted to 25 mL with deionized water. The pH of the appropriate aliquots of the solution was adjusted to 5.0. The surface river water and lake water samples were collected from Hun River and Nan Lake, Shenyang, China in May 2016. Samples were filtrated immediately through nitrocellulose membrane (pore size: 0.45 μm, Sartorius, Germany) and were acidified with HCl to pH 2 for conservation. All the samples were stored in polyethylene bottles, kept in the refrigerator at 4 °C before use and filtered prior to analytical procedure. Tap water samples were taken from our research laboratory without pretreatment. Before SPE procedure, the samples were adjusted to pH 5.0 according to optimized experiment conditions.
2.5 Preconcentration procedures
The separation and preconcentration studies were carried out in a packed-bed system. The stopcock of the glass column (100 mm in length and 10 mm in diameter) was covered with a fritted glass disc. A total of 100 mg of extractant was slurred in water, and then poured into the column. A small amount of glass wool was placed on the disc to prevent loss of the sorbents during sample loading. The column height (adsorption bed) was about 15 mm. It was treated successively with 2 mol L−1 HCl and water. The column was preconditioned by passing a blank solution and then a solution containing 2.5 μg of Sb(III) in a volume of 250 mL was passed through the column at a flow rate of 5.0 mL min−1 (controlled by a peristaltic pump) after adjusting pH 5.0. The column was washed with 5 mL water and then eluted with 5 mL of 5% HCl. The desorbed Sb(III) in eluent was measured by HG-AFS.
3. Results and discussion
3.1 Sorbent characterization
The mercapto-functionalized hybrid sorbent was characterized by FT-IR spectrum as shown in Fig. 1a. The FT-IR spectrum exhibits the stretching vibrational absorption bands for the corresponding organosilane functionalities such as S–H stretching vibrations from thiol groups at 2561 cm−1 and C–H symmetric stretching vibrations, asymmetric stretching vibrations and bending vibration from mercaptopropyl groups at 2933, 2866 and 1410 cm−1, which are the characteristic absorption peaks of mercaptopropyl groups.21 The peaks at 3448 and 1632 cm−1 reflect the stretching vibration and bending vibration of O–H bond of hydroxy remaindered in sorbent. The peaks at 1086, 804 and 467 cm−1 come from the stretching vibrations of Si–O–Si, Si–O–Si and Si–O groups, respectively.22 It could be ascertained that the mercaptopropyl group was successfully grafted onto the surface of silica gel.
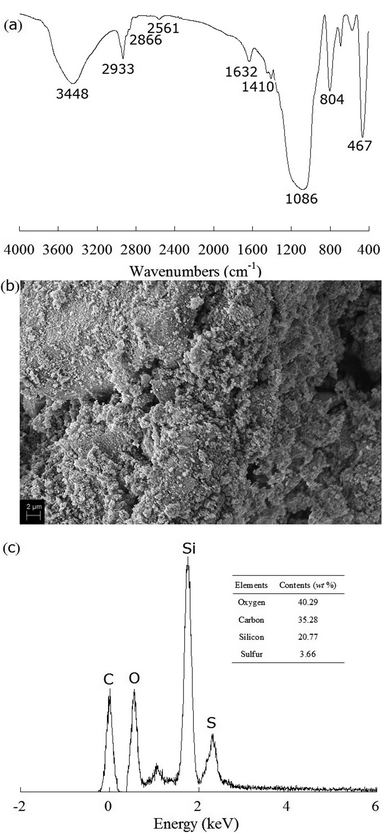 |
| Fig. 1 (a) IR spectrum, (b) SEM image and (c) EDS spectra of mercapto-functionalized hybrid sorbent. | |
It can be seen in Fig. 1b that SEM micrographs for mercapto-functionalized hybrid sorbent indicate loose aggregate morphology consisting of irregularly shaped particles with a broad size distribution varying from 100 to 1000 nm, which tend to form larger accumulations. EDS was used in order to evaluate the elemental composition of the prepared mercapto-functionalized hybrid sorbent. Fig. 1c indicates the presence of the S-peak, which is characteristic for the mercapto group (–SH). Based on the EDS results, it could be concluded that the mercaptopropyl groups were successfully attached to the surfaces of the silica.
3.2 Effect of pH
The pH of the aqueous solution plays an important role in the preconcentration of the analytes by SPE due to the enrichment of heavy metal ions based on chelation. The concentration of hydrogen ion significantly affects the formation of metal–ligand complex. The effects of pH on the preconcentration of 10 μg each of Sb(III) and Sb(V) present in 1 litre of solution were investigated at the pH range 3–9, meanwhile keeping the other parameters constant. The deposition percentage of analytes depends on the sample pH as shown in Fig. 2. The high deposition percentage of Sb(III) was found to be constant in the pH range of 3–9. Low enrichment efficiency of Sb(V) was achieved at a whole pH range investigated. The high deposition percentage of Sb(III) (>99%) and low enrichment efficiency of Sb(V) were due to the strong affinity of mercapto group on the mercapto-functionalized hybrid sorbent with Sb(III) species and the weak affinity with Sb(V) species.23 The mercapto groups as “soft acid” owns a high affinity for “soft” Sb(III) species and a low affinity for “hard” Sb(V) species according to the hard-soft acid-bases theory.24 The relatively high enrichment efficiencies of Sb(V) species at strongly acidic conditions were observed for a comparison with the weak acidic and alkaline conditions. To achieve high efficiency and good selectivity, a pH of 5.0 was selected for the subsequent work.
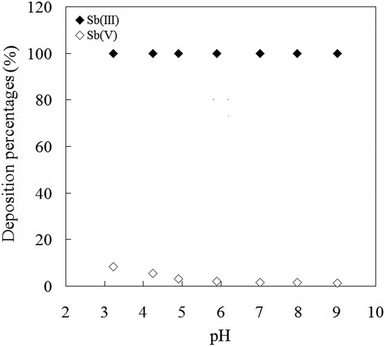 |
| Fig. 2 Effect of pH of the sample solution on the deposition percentage of Sb(III) ions onto mercapto-functionalized hybrid sorbent. | |
3.3 Effect of the amount of sorbent
The amount of the sorbent is another important factor on the column studies for the quantitative recovery of Sb(III) species. Effect of the different amounts of mercapto-functionalized hybrid sorbent as SPE extractant on the deposition percentage of Sb(III) species was investigated in the range of 25–200 mg. Aliquots of 5.0 mL of 10 μg L−1 Sb(III) species solutions were separately introduced into a series of packed columns containing different amounts of mercapto-functionalized hybrid sorbent. After passing solutions through the column, the deposition percentages of Sb(III) species on the mercapto-functionalized hybrid sorbent were calculated. The results are depicted in Fig. 3. The high deposition percentages of Sb(III) species (>99%) were achieved in the range of 100–200 mg of the mercapto-functionalized hybrid sorbent. After that, deposition percentage of Sb(III) species onto mercapto-functionalized hybrid sorbent was found to be constant. Therefore, 100 mg of mercapto-functionalized hybrid sorbent as SPE extractant was used in all the subsequent experiments.
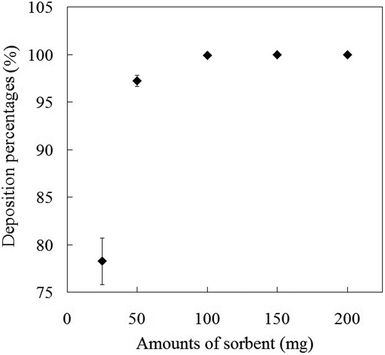 |
| Fig. 3 Effect of the amount of extractant on the deposition percentage of Sb(III) ions onto mercapto-functionalized hybrid sorbent. | |
3.4 Effect of sample flow rate
One of the parameters affecting the recovery and preconcentration is the sample flow rate, which is a decisive factor of the contact time between the sample solution and the sorbent. The influences of the flow rate of the sample solution for the deposition percentages of analytes were investigated by pumping aliquots of 20.0 mL of 10.0 μg L−1 Sb(III) species solutions at pH of 5.0 into a packed column containing 100 mg of mercapto-functionalized hybrid sorbent at different flow rates ranges of 1–20 mL min−1. The flow rate was adjusted simply by connecting the adsorbing column to a flask, which was under controllable vacuum. The results indicated that the sample flow rate variations in the range of 2–10 mL min−1 had no significant effect on the deposition percentages of Sb(III) species onto mercapto-functionalized hybrid sorbent (as shown in Fig. 4). It can be seen from Fig. 4 that the adsorption of the Sb(III) species onto mercapto-functionalized hybrid sorbent is a relatively rapid kinetic process. In order to decrease in analysis time and achieve a good precision, all the further studies were performed at the sample flow rate of 5.0 mL min−1.
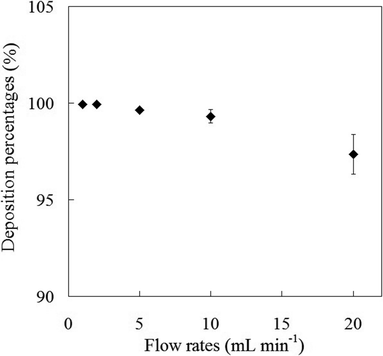 |
| Fig. 4 Effect of sample flow rate on the deposition percentage of Sb(III) ions onto mercapto-functionalized hybrid sorbent. | |
3.5 Optimization of elution conditions
3.5.1 Type and concentration of eluent. Hydrochloric and nitric acid were used as the eluents for the desorption of Sb(III) species from mercapto-functionalized hybrid sorbent in SPE column. In the wide pH range of 2–11, the Sb(OH)3 is the principal species. Sb(OH)4− dominates only above pH 11.8, whereas the cationic form Sb(OH)2+ is predominant at pH < 1.4.25 The desorption percentage of the eluent with different concentration was investigated by taking volumes of 5.0 mL at 5 mL min−1 flow rate of the each eluent. The results for this experiment are summarized in Table 1. The results showed that the desorption percentages for Sb(III) species using 5% and 10% of acids as eluent were higher than with 1% of acids. The desorption percentages of Sb(III) species were more than 95% with 5% and 10% HCl solution or HNO3 solution. There were no notable difference of desorption percentages between 5% and 10% of acids. The desorption of Sb(III) species from mercapto-functionalized hybrid sorbent was due to the protonation of mercapto groups and the competition between H+ and Sb(OH)2+ ions.18 Compared with HNO3, HCl is cheaper. Therefore, 5% HCl solution was used as the effective eluent for the quantitative recovery of Sb(III) species.
Table 1 Effect of eluents on the desorption of Sb(III) ions
Eluents |
Desorption percentages (%) |
1% of HNO3 solution |
85.8 ± 3.3 |
5% of HNO3 solution |
96.2 ± 2.2 |
10% of HNO3 solution |
97.4 ± 2.0 |
1% of HCl solution |
84.9 ± 2.9 |
5% of HCl solution |
96.4 ± 2.1 |
10% of HCl solution |
97.1 ± 1.9 |
3.5.2 Volume of eluent. To investigate the effect of the volume of eluent, various volumes (1–20 mL) of 5% HCl solution were studied for desorption of the retained analytes from extractant. Effect of eluent volume on desorption percentages is shown in Fig. 5. It was found that Sb(III) species could be quantitatively recovered from extractant with the high desorption percentages (>95%) when the volume range of eluent was more than 5.0 mL. In the eluent volume lower than 5.0 mL, the desorption percentages of the Sb(III) species were poor because of insufficient eluent volume. For achieving the higher enrichment factor, 5.0 mL of eluent was used in the following experiments.
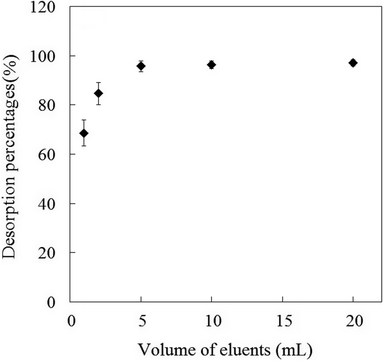 |
| Fig. 5 Effect of eluent volume on the desorption percentage of Sb(III) ions from mercapto-functionalized hybrid sorbent. | |
3.5.3 Flow rate of eluent. Flow rate of eluent solution is one of important factors for the quantitative recoveries and desorption of Sb(III) species from mercapto-functionalized hybrid sorbent. The influence of the flow rate of eluent solution for the recoveries of Sb(III) species was investigated in the flow rate ranges of 1.0–20.0 mL min−1 (as presented in Fig. 6). The desorption percentages for Sb(III) species were more than 95% high in the flow rates range from 1.0 mL min−1 to 5.0 mL min−1. In posterior experiments, the flow rate of eluent solution was kept constant at 5.0 mL min−1.
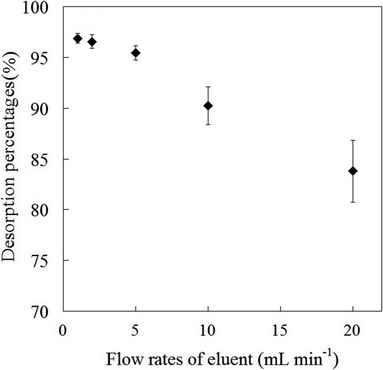 |
| Fig. 6 Effect of eluent flow rate on the desorption percentage of Sb(III) ions from mercapto-functionalized hybrid sorbent. | |
3.6 Breakthrough volume
Sb(III) species were quantitatively retained from the different sample volumes of 50–1000 mL containing the same amounts of metal ions (2.5 mg). The deposition percentage for Sb(III) species was very efficient (>95%) for the sample volume up to 1000 mL. Consequently, by considering the final elution volume of 5.0 mL of 5% HCl solution and the break through volume of 1000 mL, a preconcentration factor of 200 could be obtained easily. However, in order to decrease the analysis time, sample volumes of 250 mL was used for the preparation of calibration curves with a preconcentration factor of 50.
3.7 Interference studies
In order to examine the effect of coexisting ions on the determination of Sb(III) species in the optimum conditions, the interferences of coexisting ions in binary mixtures containing Sb(III) species and one of the foreign ions were investigated on percent recovery of Sb(III) species. After introducing the binary solution into the column, the adsorbed Sb(III) species were eluted by 5.0 mL of 5% HCl solution at the flow rate of 5.0 mL min−1. Then the content of Sb(III) species in the effluents was determined using HG-AFS and the recoveries were calculated. Any deviation of ±5% or more from the absorbance value of the standard solution was considered as interference. The results are presented in Table 2. The results indicated that most of the tested ions except Fe(III) ion had no notable influence on the determination of Sb(III) species. The following tolerance amount ratios were obtained: 5000 for Mg(II) and Ca(II), 1000 for Zn(II), Cu(II), Co(II), Ni(II), Pb(II), and Cd(II), and 500 for Cr(III) and As(III). The interfering effect of Fe(III) ions can be improved by the addition of excess amounts of F(I−) ions (1 × 10−2 mol L−1) to the sample solution prior to its introduction into the column. The tolerance ratio of Fe(III) ions can be improved to 500. The results showed that the interferences of most foreign ions for the preconcentration of Sb(III) species in real samples were negligible.
Table 2 Effects of the foreign ions on the recovery of 10.0 μg L−1 of Sb(III) ions
Foreign ions |
Tolerance ratios (Cforeign ions/CSbIII) |
Recovery (%) |
The concentration of F(I−) ion is 0.01 mol L−1 and F(I−) ion in solution is excess. |
Ca(II) |
5000 |
97.1 ± 0.8 |
Mg(II) |
5000 |
97.9 ± 0.4 |
Cd(II) |
1000 |
98.1 ± 0.4 |
Zn(II) |
1000 |
98.6 ± 1.6 |
Cu(II) |
1000 |
98.6 ± 0.7 |
Pb(II) |
1000 |
99.1 ± 0.4 |
Co(II) |
1000 |
98.4 ± 0.6 |
Ni(II) |
1000 |
97.8 ± 0.6 |
As(III) |
500 |
95.7 ± 1.7 |
Cr(III) |
500 |
97.8 ± 1.6 |
Fe(III)a |
500 |
95.8 ± 2.6 |
3.8 Analytical performance
The calibration curve was obtained by using 250 mL of 0.010–10.0 μg L−1 Sb(III) species solutions at the optimum condition. The contents of Sb(III) species in eluting solutions were determined by HG-AFS. The linear calibration curve was observed in the concentration range of 1.0–200.0 μg L−1 for Sb(III) species. The regression coefficient of calibration curve was 0.9905. The relative standard deviations (RSD) for eleven replicate measurements at a concentration level of 10 μg L−1 of Sb(III) species was 1.6%. The values of the detection limits (LOD), defined as the concentration of analyte giving signals equivalent to three times, the standard deviation of the blank signal plus the net blank intensity were found to be 2 ng L−1 when 250 mL of solution was preconcentrated to a final volume of 5.0 mL. The instrumental LOD for Sb(III) species was also determined by using blank solutions without preconcentration and found to be 0.1 μg L−1 for comparison.
3.9 Analysis of standard reference materials
The accuracy of the proposed method was investigated by examining the standard reference materials (SLRS-5 trace elements in river water, GBW(E) 080696 antimony component analysis solution and GBW07309 stream sediment) following the experimental procedure. The total concentrations of Sb in standard reference materials are known, whereas the speciation of Sb in the standard reference materials is unknown. In order to test the accuracy of the proposed method, the standard reference materials were diluted appropriately with 5% HCl solution and 5 mL of 10% vitamin C solution. The vitamin C was excess by calculation. The excess vitamin C can reduce Sb(V) to Sb(III) and can ensure the existence of antimony as Sb(III) species in the standard reference materials aqueous solution. The results were found to be in good agreement with the certified values (as seen in Table 3). These values revealed that there was no significant difference between the certified values and the measured values by performing t-test at 95% confidence limit, thus confirming the accuracy of the proposed procedure.
Table 3 The results for the determination of Sb in the certified reference materials
CRMs |
Certified value |
Found value |
Indicative value. |
SLRS-5 |
0.23 mg L−1a |
0.21 ± 0.03 mg L−1 |
GBW(E) 080696 |
9.87 mg L−1a |
9.9 ± 0.1 mg L−1 |
GBW07309 |
0.81 ± 0.23 mg kg−1 |
0.90 ± 0.2 mg kg−1 |
3.10 Applications
In order to assess the applicability of the proposed procedure to the analysis of real samples including pure water, tap water, river water (from the Hun River, Shenyang, China), mineral wastewater and sea water, were analyzed according to the general procedure. The correctness of results was verified by analyzing the concentration after the addition of known amounts of Sb(III) species into water samples. Different amounts of the investigated Sb(III) species were spiked by adding 1.250 and 2.50 μg of Sb(III) species in a water sample and diluting to 250 mL and the resulting solutions were submitted to the preconcentration procedure. The results (deducted the reagents blank) are shown in Table 4. The recovery values for Sb(III) species were found to be in the range of 95–102% for spiked Sb(III) species. Good agreement was achieved between the added and found concentrations of analytes using the proposed procedure. A comparison of the analytical performance obtained by the proposed method with other extractants reported in the literature for Sb(III) species is shown in Table 5.7–12,26 As can be seen, the relative low LOD of the proposed method were obtained with other SPE extractants reported in the literature for Sb(III) species for comparison. The proposed method had the lowest precision except the results reported in the ref. 12, and had also the narrowest range of recovery. The results indicated that mercapto-functionalized hybrid sorbent was suitable for the selective separation and preconcentration of Sb(III) species at the μg L−1 levels in real water samples.
Table 4 Analytical application
Samples |
Sb(III) added (μg L−1) |
Sb(III) founda (μg L−1) |
Recovery (%) |
Mean ± SD for three replicate measurements of three individual preparations. Below detection limit. |
Pure water |
0.00 |
LODb |
— |
5.0 |
4.9 ± 0.2 |
98 ± 3 |
10.0 |
10.2 ± 0.3 |
102 ± 3 |
Tap water |
0.00 |
LOD |
— |
5.0 |
5.1 ± 0.2 |
102 ± 4 |
10.0 |
9.9 ± 0.3 |
99 ± 3 |
Hun River |
0.00 |
LOD |
— |
5.0 |
4.9 ± 0.2 |
98 ± 3 |
10.0 |
9.8 ± 0.3 |
98 ± 3 |
Mineral wastewater |
0.00 |
33.5 ± 1.3 |
— |
5.0 |
38.3 ± 0.9 |
96 ± 3 |
10.0 |
43.7 ± 1.5 |
102 ± 4 |
Sea water |
0.00 |
LOD |
— |
5.0 |
4.8 ± 0.3 |
96 ± 6 |
10.0 |
9.5 ± 0.5 |
95 ± 5 |
Table 5 Comparison of analytical performance with other SPE extractants reported in the literature for Sb(III) species
SPE extractants |
LOD (ng L−1) |
Precision (%) |
Recovery (%) |
Ref. |
Silica-based octyl sorbent |
1 |
— |
90.2–114 |
7 |
Multi-walled carbon nanotubes |
3.5 |
4.8 |
96–104.1 |
8 |
C8–Fe3O4@SiO2 nanoparticles |
1 |
3.4 |
91–105 |
9 |
Nano γ-alumina |
6 |
2.8 |
93–107 |
10 |
Single-walled carbon |
2.1 |
4.8 |
92–106.5 |
11 |
Functionalized mesoporous silica |
1 |
0.9 |
91.3–109.9 |
12 |
TiO2 |
140 |
6.8 |
103–120 |
26a |
C16-bonded silica gel |
7 |
3.8 |
— |
26b |
Dowex 1×8 – 200 |
2000 |
3.1 |
92.8–102.5 |
26c |
Yeast immobilized polyurethane foam |
150 |
— |
81–113 |
26d |
Mercapto-functionalized hybrid sorbent |
2.5 |
1.6 |
95–102 |
This work |
4. Conclusion
By using mercapto-functionalized hybrid sorbents as SPE extractant, a simple, rapid, powerful, advanced, precise, accurate and reliable method was developed for the separation and preconcentration of Sb(III) species prior to the determination by HG-AFS analysis in real samples. The value of LOD was found to be 2 ng L−1 when a sample volume of 1000 mL was preconcentrated to a final volume of 5.0 mL. The obtained optimum pH range was found to be at a range of 3–9. Most of the cations were free of interference for the preconcentration of Sb(III) species within a wide range of concentration. The results obtained by analyzing standard reference materials proved that the accuracy and precision of the proposed procedure were very good. The proposed procedure showed very good recoveries (95–102%) of several water samples. Thus, mercapto-functionalized hybrid sorbent as the SPE extractant had been demonstrated to be promising for the routine laboratories in the determination of Sb(III) species even at very low concentration.
Conflicts of interest
There are no conflicts to declare.
Acknowledgements
Sponsored by the National Natural Science Foundation of China (grant no. 21477082 and 21107076) and by Liaoning Natural Science Foundation (grant no. 201602402).
References
-
(a) USEPA, Antimony: An Environmental and Health Effects Assessment, US Environmental Protection Agency, Office of Drinking Water, Washington, DC, 1984 Search PubMed;
(b) M. M. López Guerrero, E. Vereda Alonso, J. M. Cano Pavón, M. T. Siles Cordero and A. J. García de Torres, J. Anal. At. Spectrom., 2016, 31, 975–984 RSC.
- M. Filella, N. Belzile and Y.-W. Chen, Earth-Sci. Rev., 2002, 59, 265–285 CrossRef CAS.
- M. Filella, N. Belzile and Y.-W. Chen, Earth-Sci. Rev., 2002, 57, 125–176 CrossRef CAS.
- N. Gurnani, A. Sharma and G. Tulukder, Nucleus, 1994, 37, 71–96 CAS.
-
(a) S. C. Apte, J. Anal. At. Spectrom., 1986, 1, 38–47 Search PubMed;
(b) Z. Li and Y. Guo, Talanta, 2005, 65, 1318–1325 CrossRef CAS PubMed;
(c) R. Wietecha, P. Kościelniak, T. Lech and T. Kielar, Microchim. Acta, 2005, 149, 137–144 CrossRef CAS.
-
(a) V. Camel, Spectrochim. Acta, Part B, 2003, 58, 1177–1233 CrossRef;
(b) Y. Zhai, Y. Liu, X. Chang, S. Chen and X. Huang, Anal. Chim. Acta, 2007, 593, 123–128 CrossRef CAS PubMed;
(c) E. M. Thurman and M. S. Mills, Solid-Phase Extraction, Principles and Practice, Wiley, New York, 1998 Search PubMed;
(d) M. M. López Guerrero, M. T. Siles Cordero, E. Vereda Alonso, J. M. Cano Pavón and A. García de Torres, J. Anal. At. Spectrom., 2015, 30, 1169–1178 RSC.
- C. Yu, Q. Cai, Z. X. Guo, Z. Yang and S. B. Khoo, Analyst, 2002, 127, 1380–1385 RSC.
- H. Fazelirad and M. A. Taher, Microchim. Acta, 2014, 181, 655–662 CrossRef CAS.
- P. Li, Y. Chen, X. Hu and H. Lian, Talanta, 2015, 134, 292–297 CrossRef CAS PubMed.
- J. Tian, T. Huang and J. Lu, Food Anal. Methods, 2013, 6, 1080–1089 CrossRef.
- H. Wu, X. Wang, B. Liu, Y. Liu, S. Li, J. Lu, J. Tian, W. Zhao and Z. Yang, Spectrochim. Acta, Part B, 2011, 66, 74–80 CrossRef.
- M. M. López Guerrero, M. T. Siles Cordero, E. Vereda Alonso, A. J. García de Torres and J. M. Cano Pavón, Talanta, 2014, 129, 1–8 CrossRef PubMed.
- K. Zih-Perényi, P. Jankovics, E. Sugár and A. Lásztity, Spectrochim. Acta, Part B, 2008, 63, 445–449 CrossRef.
- A. Kaur and U. Gupta, J. AOAC Int., 2010, 93, 1302–1307 CAS.
- A. Calvo Fornieles, A. García de Torres, E. Vereda Alonso and J. M. Cano Pavón, J. Anal. At. Spectrom., 2013, 28, 364–372 RSC.
- G.-Z. Fang, J. Tan and X.-P. Yan, Anal. Chem., 2005, 77, 1734–1739 CrossRef CAS PubMed.
-
(a) J. B. Wu, S. Y. Zang and Y. L. Yi, J. Sol-Gel Sci. Technol., 2013, 66, 434–442 CrossRef CAS;
(b) L. He, B. B. Wang, D. D. Liu, K. S. Qian and H. B. Xu, Korean J. Chem. Eng., 2014, 31, 343–349 CrossRef CAS;
(c) D. P. Sui, H. X. Chen and D. W. Li, J. Sol-Gel Sci. Technol., 2016, 80, 504–513 CrossRef CAS.
- D. Zhang, Y. Zhao and H.-B. Xu, Sep. Sci. Technol., 2017, 52, 1938–1945 CrossRef CAS.
- T.-L. Deng, Y.-W. Chen and N. Belzile, Anal. Chim. Acta, 2001, 432, 293–302 CrossRef.
-
(a) Buhani, Narsito, Nuryono and E. S. Kunarti, Desalination, 2010, 251, 83–89 CrossRef CAS;
(b) C. A. Quirarte-Escalante, V. Soto, W. Cruz, G. R. Porras, R. Manríquez and S. Gomez-Salazar, Chem. Mater., 2009, 21, 1439–1450 CrossRef CAS.
-
(a) B. Viltužnik, A. Košak, Y. L. Zub and A. Lobnik, J. Sol-Gel Sci. Technol., 2013, 68, 365–373 CrossRef;
(b) J. Wu, J. Xie, L. Ling, G. Ma and B. Wang, J. Coat. Technol. Res., 2013, 10, 849–857 CrossRef.
- L. He, B.-B. Wang, D.-D. Liu, K.-S. Qian and H.-B. Xu, Korean J. Chem. Eng., 2014, 31, 343–349 CrossRef.
- M. Matsui, K. Matsumoto and K. Terada, Anal. Chim. Acta, 1987, 193, 381–385 CrossRef CAS.
- H. Sun, S. C. Yan and W. S. Cheng, Eur. J. Biochem., 2000, 267, 5450–5457 CrossRef CAS PubMed.
- F. Wu, F. Sun, S. Wu, Y. Yan and B. Xing, Chem. Eng. J., 2012, 183, 172–179 CrossRef.
-
(a) L. Zhang, Y. Morita, A. Sakuragawa and A. Isozaki, Talanta, 2007, 72, 723–729 CrossRef CAS PubMed;
(b) S. Garbos, M. Rzepecka, E. Bulska and A. Hulanicki, Spectrochim. Acta, Part B, 1999, 54, 873–881 CrossRef;
(c) F. S. Rojas, C. B. Ojeda and J. M. C. Pavón, Talanta, 2007, 72, 951–956 CrossRef CAS PubMed;
(d) A. A. Menegário, A. J. Silva, E. Pozzi, S. F. Durrant and C. H. Abreu, Spectrochim. Acta, Part B, 2006, 61, 1074–1079 CrossRef.
Footnote |
† The first two authors contributed equally to this paper. |
|
This journal is © The Royal Society of Chemistry 2018 |
Click here to see how this site uses Cookies. View our privacy policy here.