DOI:
10.1039/C7RA13267K
(Paper)
RSC Adv., 2018,
8, 5280-5285
Simultaneous voltammetric detection of dopamine, ascorbic acid and uric acid using a poly(2-(N-morpholine)ethane sulfonic acid)/RGO modified electrode
Received
12th December 2017
, Accepted 23rd January 2018
First published on 30th January 2018
Abstract
A poly(2-(N-morpholine) ethane sulfonic acid)/reduced graphene oxide (RGO) modified glassy carbon electrode (GCE) was prepared using an electropolymerization method, and was characterized by scanning electron microscopy (SEM) and electrochemical impedance spectroscopy (EIS). The electrochemical behaviors and simultaneous detection of ascorbic acid (AA), dopamine (DA) and uric acid (UA) at this electrode were studied by cyclic voltammetry (CV) and differential pulse voltammetry (DPV). Tests showed that this electrode exhibited excellent electrocatalytic activity towards the oxidation of AA, DA and UA. The oxidation peak currents of AA, DA and UA were proportional with their concentrations in the ranges 1.0 μM–30 μM (30 μM–100 μM), 0.05 μM–100 μM and 0.1 μM–100 μM, with detection limits of 0.43 μM, 0.0062 μM and 0.056 μM, respectively. In addition, this electrode exhibited an excellent selectivity, reproducibility and stability, and has been successfully used to determine real samples with satisfactory results.
1 Introduction
Ascorbic acid (AA), dopamine (DA) and uric acid (UA) are considered as important molecules for physiological processes in human metabolism. DA is one of the crucial catecholamine neurotransmitter molecules widely distributed in the mammalian central nervous system.1,2 It plays an important role in the function of the central nervous, renal, hormonal and cardiovascular systems.3,4 The dysfunction of the dopaminergic system in the central nervous system can result in some diseases or neurological disorders such as schizophrenia, Parkinson's disease and HIV infection.5,6 AA, presenting in both the animal and plant kingdoms, is an essential vitamin for humans, and has been used for the prevention and treatment of the common cold, mental illness, infertility, cancer and AIDS.7 UA is the primary end product of purine metabolism. The extreme abnormalities of UA levels in the body are symptoms of several diseases including gout, hyperuricemia, and Lesch–Nyan disease.8 Therefore, real time monitoring of AA, DA and UA in biological samples and pharmaceutical preparations is of great significance, shows an important issue in diseases diagnosis.
AA, DA and UA are of electrochemical active, therefore, electrochemical techniques have been considered as potential approaches to detect AA, DA and UA. However, it is a nontrivial task to simultaneously determine AA, DA and UA directly at ordinary (carbon and metal) electrodes, because these substances coexist in the extracellular fluid of the central nervous system and serum.9–11 Thus, the development of novel modified electrodes to distinguish AA, DA and UA in mixtures is a very active research area. Up to now, various modified electrodes, including nano-material,12–17 self-assemble monolayer,18–20 layer by layer self-assemble21–23 and polymer films24–27 have been successfully constructed for individually and simultaneously detecting these substances. Among these modified electrodes, polymer film modified electrode has attracted researcher's attention due to its advantages and wide applications in the chemical fields.28–31 Lin et al.24 used DNA/poly (p-aminobenzensulfonic acid) composite bi-layer modified electrode for determination of DA and UA under coexistence of AA; Zhang et al.25 used polyaniline nano-networks/p-aminobenzene sulfonic acid functionalized electrode for the simultaneous determination of AA and UA; Hu et al.26 used poly(acid chrome blue K) modified electrode for simultaneous determination of DA, AA and UA; Chen et al.32 used poly (4 – amino – 1 – 1′ – azobenzene-3,4′-disulfonic acid) modified electrode for selective detection of DA; Ohsaka et al.33 used poly (N,N-dimethylaniline)-modified electrode for simultaneous electroanalysis of DA and AA; Huang et al.34 fabricated a poly(p-toluene sulfonic acid) modified electrode for simultaneous detection of DA and AA. Recently, nanomaterial-based electrochemical sensors have been pay wide attention to due to its excellent performances,35 various nanomaterials modified electrodes have been employed for AA, DA and UA analysis. RGO-based hybrids bring new opportunities for improving sensor performances due to these hybrids affording significant physicochemical properties.36 Among these RGO-based hybrids, combining RGO with conducting polymers show important potential application in sensor field.37 2-(N-morpholine)ethane sulfonic acid (MES) with a morpholine ring, is often used as a buffering agent in biology and biochemistry. Previous study indicated that MES can be electropolymerized onto the electrode surface for silver electrodeposition providing good interface.38
Herein, a poly(2-(N-morpholine)ethane sulfonic acid) (PMES)/RGO modified electrode was constructed and used for simultaneously detecting AA, DA and UA (Scheme 1). To the best of our knowledge, it was for the first time report its application for determination of AA, DA and UA. Based on experiment results, a sensitive method for simultaneous determination of AA, DA and UA was established for routine analysis.
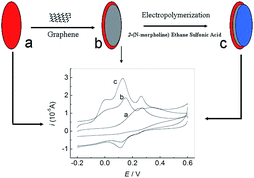 |
| Scheme 1 Schematic representation of the preparation process of PMES/RGO/GCE and its electrocatalytic activity towards the oxidation of AA, DA and UA. | |
2 Results and discussion
2.1 SEM characterization of PMES/RGO film
Fig. 1 showed the morphologies of RGO (a), PMES/RGO (b) characterized by SEM. Compared with RGO, a thin film layer was covered on its surface (b), because MES molecules were electropolymerized onto the RGO/GCE surface, indicated that PMES/RGO film could form on GCE surface.
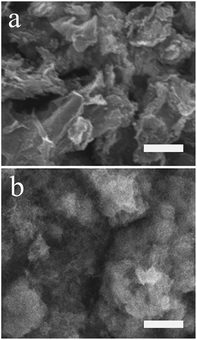 |
| Fig. 1 SEM images of RGO (a), PMES/RGO (b). The scale bar was 4 μm. | |
2.2 Electrochemical impedance characterization of PMES/RGO film
The EIS can be used to characterize the electrode surface modification process based on the electron-transfer resistance change (Ret) which is the semicircle diameter on EIS curve. We utilized the Ret to observe the change of electronic transfer resistance. Fig. 2 exhibited EIS curves of different electrodes. It can be observed that a lower Ret for RGO/GCE, compared with bare GCE, indicated RGO has better conductivity. The modification of PMES on the RGO/GCE surface resulted in a larger Ret, the reason may be that they have a charge repulsion role because of PMES film and redox probe are negatively charge. The above results indicated that PMES/RGO/GCE was successfully prepared.
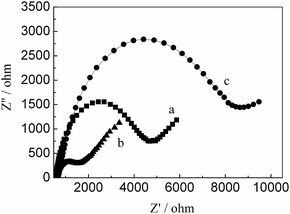 |
| Fig. 2 The Nyquist plots of bare GCE (a), RGO/GCE (b), and PMES/RGO/GCE (c) in the presence of 10 mM Fe(CN)63−/4− (1 : 1) as a redox probe (bias potential was 0.2 V and the frequency range was from 0.1 to 105 Hz, the amplitude of the alternate voltage was 5 mV). | |
2.3 Separation of the electrochemical responses of AA, DA and UA
Fig. 3 showed CVs of the mixture solution of AA, DA and UA at different electrodes in 0.1 M PBS (pH 7.0). A broad oxidation peak was observed (a), suggesting the peak potentials for AA, DA and UA are indistinguishable at the bare GCE. However, for PMES/RGO/GCE (c), three well-separated oxidation peaks corresponding to the electrooxidation of AA, DA and UA can be observed, which was enough to simultaneously detect them in mixture solution.
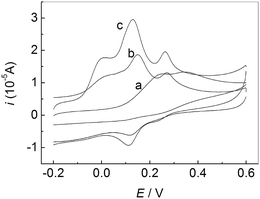 |
| Fig. 3 CVs of the mixture solution of AA, DA and UA at different electrodes in 0.1 M PBS (pH 7.0): bare GCE (a), PMES/GCE (b), and PMES/RGO/GCE (c). Scan rate: 100 mV s−1. | |
2.4 Single oxidation of AA, DA and UA
Fig. 4 showed CVs of AA (A), DA (B) and UA (C) at bare GCE and PMES/RGO/GCE in 0.1 M PBS (pH 7.0). Curves (a) and (b) correspond to bare GCE and PMES/RGO/GCE in the presence of AA, DA and UA, respectively. Compared with bare GCE, PMES/RGO/GCE can considerably enhance the oxidation peak currents of AA, DA and UA, and with more negative oxidation peak potentials, indicating that PMES/RGO/GCE had excellent electrocatalytic activities towards the oxidations of AA, DA and UA.
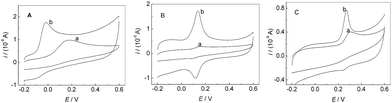 |
| Fig. 4 CVs of 0.05 mM AA (A), 0.5 μM DA (B) and 0.5 mM UA (C) at bare GCE (a) and PMES/RGO/GCE (b) in 0.1 M PBS (pH 7.0). Scan rate: 100 mV s−1. | |
2.5 Effect of pH
The effect of the solution pH on the response of AA, DA and UA were investigated in the range of 4.0–9.0. Fig. 5 showed the relationship of the oxidation peak currents of AA, DA and UA with pH, respectively. The oxidation peak current of AA decreased slightly with increasing pH until it reached 6.0, then it increased until pH reached 8.0. Further increasing pH, the oxidation peak current slightly decreased. For DA, the oxidation peak current increased with increasing pH until pH reached 7.0, and then it decreased when pH exceeded 7.0. For UA, the oxidation peak current decreased with increasing pH. In addition, all the oxidation peak potentials for AA, DA and UA shifted towards negative direction with increasing pH, showing that protons have taken part in their electrode processes. PBS (pH 7.0), much closer to physical conditions, was chosen for the following experiments.
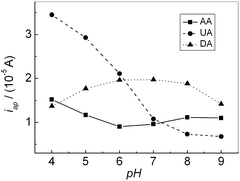 |
| Fig. 5 Effect of pH on the oxidation peak current of AA, DA and UA, respectively. | |
2.6 Effect of scan rate
The dependence of oxidation peak current of AA, DA and UA on scan rate was investigated as shown in Fig. 6. For DA and UA, The oxidation peak current increased linearly with the increase of scan rate, and the peak current (Ip) was proportional to scan rate (v) from 20 to 200 mV s−1, respectively. The linear regression equation was Ipa = 0.02 + 0.08v (r = 0.9967) and Ipa = 2.50 + 0.09v (r = 0.9983), respectively, suggesting an adsorption controlled process. For AA, the oxidation peak current was proportional to the square root of scan rate over the range of 20–200 mV s−1, the linear regression equation was Ipa = 1.67 + 0.74v1/2 (r = 0.9912), suggesting a diffusion controlled process.
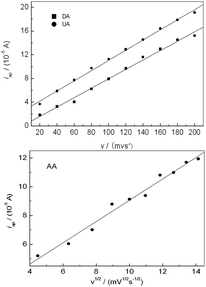 |
| Fig. 6 Effect of scan rate on the oxidation peak current of AA, DA and UA, respectively. | |
2.7 Simultaneous determination of AA, DA and UA
Fig. 7A showed the peak current of AA increased with its concentration increasing, when the concentration of DA and UA were kept constant. In addition, the change of AA concentration did not have significant influence on the peak currents and peak potentials of the other two compounds. Similarly, as shown in Fig. 7B and C, the oxidation peak current of DA or UA increased with the increase of the concentration of DA or UA by keeping the concentration of other two compounds constant. The above results confirmed that it was possible to simultaneously determine AA, DA and UA in mixture samples based on PMES/RGO/GCE.
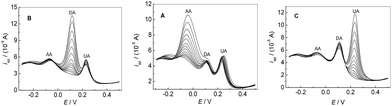 |
| Fig. 7 DPVs for the mixture solution containing different concentrations AA, DA and UA in 0.1 M PBS (pH 7.0) at PMES/RGO/GCE under keeping the concentrations of other two compounds constant. | |
If the concentrations of AA, DA and UA increased synchronously, the oxidation peak currents at the modified electrode increased accordingly as shown in Fig. 8. It can be seen that the oxidation peak currents of three analytes increase linearly with their concentrations, respectively. The relationship between the oxidation peak current and the concentration of AA, DA and UA was shown in Fig. 9, respectively. The linear ranges for AA, DA and UA were 1.0 μM–30 μM (30 μM–100 μM), 0.05 μM–100 μM, and 0.1 μM–100 μM, respectively. The regression equations were iDA (10 μA) = 0.43 + 0.051cDA (cDA: μM) (r = 0.9989) with a detection limit of 0.0062 μM (S/N = 3) and iUA (10 μA) = 0.35 + 0.046cUA (cUA: μM) (r = 0.9976) with a detection limit of 0.056 μM (S/N = 3), respectively. For AA, the regression equations were iAA (10 μA) = 0.49 + 0.038cAA (cAA: 1.0 μM–30 μM) (r = 0.988) with a detection limit of 0.43 μM (S/N = 3); iAA (10 μA) = 1.3 + 0.01cAA (cAA: 30 μM–100 μM) (r = 0.998). Compared with some reported methods,15,37,39,40 this method had excellent analytical performances for detecting the AA, DA and UA (Table 1).
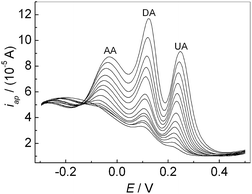 |
| Fig. 8 DPVs of the mixture solution containing AA, DA and UA at the PMES/RGO/GCE in 0.1 M PBS (pH 7.0). | |
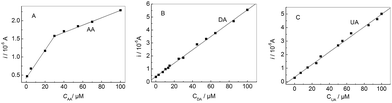 |
| Fig. 9 The oxidation peak current vs. the concentration of AA (A), DA (B) and UA (C), respectively. | |
Table 1 Comparison of linear range and detection limit with some reported methods
Modified electrode |
Linear range (μM) |
Detection limit (μM) |
References |
AA |
DA |
UA |
AA |
DA |
UA |
Zinc oxide/redox mediator/GCE |
15–240 |
6–960 |
50–800 |
1.4 |
0.7 |
4.5 |
15 |
poly(amido-amine), multi-walled carbon nanotubes and Au nanoparticles functionalized reduced graphene oxide modified electrode |
20–1800 |
10–320 |
1–114 |
6.7 |
0.3 |
0.33 |
37 |
Methylene blue/phosphorylated zirconia–silica composite electrode |
10–1600 |
6–100 |
22–350 |
8.3 ± 0.1 |
1.7 ± 0.1 |
3.7 ± 0.2 |
39 |
Reduced graphene oxide/GCE |
40–1000 |
0.1–100 |
0.8–800 |
4.2 |
0.008 |
0.6 |
40 |
PMES/RGO/GCE |
30–100, 1–30 |
0.05–100 |
0.1–100 |
0.43 |
0.0062 |
0.056 |
This work |
2.8 The reproducibility and stability
The reproducibility and stability of the modified electrode were investigated by CV response of 10 μM DA in 0.1 M PBS (pH 7.0). The fabrication of six modified electrodes, made independently, showed a well reproducibility with a relative standard deviation (RSD) of 3.4%. The stability of the modified electrode was studied by scanning for 30 continuous cycles at the potential between −0.2 and +0.6 V (vs. SCE) with a scan rate of 100 mV s−1, the peak heights of CV showed a negligible change. Therefore, the modified electrode exhibited an excellent reproducibility and stability.
2.9 Interference study and analytical application
The influence of various foreign species on the determination of 0.4 mM AA, 50 μM DA and 0.3 mM UA were investigated. The results indicated that the mutual interference from AA, DA and UA can be neglected. Other influences from common coexisting substances were also investigated. The experiment results indicated that no significant interference for the detection of AA, DA and UA for these compounds: L-lysine (20), L-cystine (20), L-tytrosine (20), glucose (20), where the data in the brackets were the concentration ratios. The PMES/RGO/GCE was applied for analysis of AA, dopamine hydrochloride injection and human urine in a mixed sample with 0.1 M PBS (pH 7.0) by DPV. In order to detect the concentration of UA in human urine from a healthy individual, the urine samples were diluted with 0.1 M PBS (pH 7.0) without any pretreatment process to fit into the linear range of UA. After that, the concentration of UA could be calculated by the regression equation of UA, and diluted to 20 μM, which was then used for testing recovery by the standard addition method. The experiment results were listed in Table 2. The recovery values were reasonable, showing that the proposed method could be efficiently used for the real sample analysis. To further verify the reliability of this method and its potential in clinical diagnosis, the fresh serum samples from a healthy female individual were treated by centrifuging and filtering before the experiments. The concentration of UA in serum was calculated with the level of approximate 171 μM by this method, which was close to the hospital's assay result (186 μM). Which indicated that the proposed method had the good reliability and potential in clinical diagnosis.
Table 2 Detection of AA, dopamine hydrochloride injection and human urine in mixture
Sample |
Original (μM) |
Added (μM) |
Found (μM) |
Recovery (%) |
AA |
10.0 |
10.0 |
19.78 |
98.90 |
Dopamine hydrochloride injection |
5.0 |
10.0 |
14.92 |
99.47 |
Human urine |
20.0 |
20.0 |
41.34 |
103.35 |
3 Experimental
3.1 Reagents and apparatus
AA, DA, UA and 2-(N-morpholine) ethane sulfonic acid were purchased from Sigma (USA), L-lysine, L-cystine, L-tytrosine, glucose and other reagents were purchased from Nanjing Chemical Reagent (Nanjing, China). 0.1 M phosphate buffer solutions (PBS) with different pH values were prepared by mixing the stock standard solution of Na2HPO4 and NaH2PO4 and pH was adjusted with H3PO4 or NaOH solution. All chemicals were of analytical reagent grade and used without any further purification. All solutions were prepared with doubly distilled water.
CV was performed on a CHI660A electrochemical workstation (Shanghai Chenhua Instruments, China). The three-electrode system was used in the experiment with bare GCE or modified electrode as working electrode, a saturated calomel electrode (SCE) as reference electrode, and a platinum wire as counter electrode. All electrochemical measurements were carried out in a 10 mL electrochemical cell, where oxygen was removed with high-purity nitrogen for 20 min and a blanket of nitrogen was maintained over the solution during the measurements. All potentials given in this paper were referred to SCE. All experiments were performed in compliance with the ethical principles of human experimentation, and approved by the ethics committee at suzhou university. Informed consents were obtained from human participants of this study.
3.2 Preparation of the PMES/RGO/GCE
Prior to modification, bare GCE was orderly polished to a mirror-like surface with 1.0, 0.3 and 0.05 μm a-Al2O3. This electrode was then successively ultrasonically washed with anhydrous ethanol and doubly distilled water, each for 1 min. RGO (1.0 mg) were dispersed in 10 mL N,N-dimethylformamide by ultrasonic agitation for about 60 min to get a 0.1 mg mL−1 black suspension. 10 μL RGO suspensions was dropped onto the fresh GCE surface and dried naturally at room temperature to form RGO film, it was then immersed into doubly distilled water for 5 min to remove loosely adsorbed RGO. The electrode was then immersed in 0.1 M PBS (pH 7.0) containing 2.0 × 10−4 M MES and the potential of working electrode was cycled between +0.2 and +2.0 V (vs. SCE) at 100 mV s−1 for 20 scans to form the PMES/RGO/GCE.38
4 Conclusions
The PMES/RGO/GCE was fabricated and used to detect AA, DA and UA and their mixture by DPV, exhibited a highly electrocatalytic activity for the oxidation of AA, DA and UA, and a large peak separations between AA, DA and UA. The modified electrode can individually or simultaneously detect AA, DA and UA with good reproducibility, stability, sensitivity and selectivity. In addition, this electrode can be applied to detect real samples with satisfactory results. The excellent performances of this method indicated a strong potential for application in the routine analysis of AA, DA and UA in clinical tests.
Conflicts of interest
There are no conflicts of interest to declare.
Acknowledgements
This project was supported by the Natural Science Research Key Project of Education Department of Anhui Province (KJ2017A434, KJ2016A888); the Opening Project of Anhui Key Laboratory of Spin Electron and Nanomaterials (2015ykf16); the Funded Project of Suzhou University Cultivate Outstanding Talent (SZXYQNL2017001); Research Team of Anhui Provincial Education Department (2016SCXPTTD); Key Discipline of Material Science and Engineering of Suzhou University (2017XJZDXK3).
Notes and references
- H. R. Zare, N. Rajabzadeh, N. Nasirizadeh and M. M. Ardakani, J. Electroanal. Chem., 2006, 589, 60–69 CrossRef CAS.
- J. Li and X. Q. Lin, Sens. Actuators, B, 2007, 124, 486–493 CrossRef CAS.
- P. Shakkthivel and S. M. Chen, Biosens. Bioelectron., 2007, 22, 1680–1687 CrossRef CAS PubMed.
- J. Kima, M. Jeona, K. J. Paengb and I. R. Paenga, Anal. Chim. Acta, 2008, 619, 87–93 CrossRef PubMed.
- R. M. Wightman, L. J. May and A. C. Michael, Anal. Chem., 1988, 60, 769A–779A CrossRef CAS PubMed.
- J. W. Mo and B. Ogorevc, Anal. Chem., 2001, 73, 1196–1202 CrossRef CAS PubMed.
- O. Arrigoni and M. C. Tullio, Biochim. Biophys. Acta, 2002, 1569, 1–9 CrossRef CAS.
- V. S. E. Dutt and H. A. Mottola, Anal. Chem., 1974, 46, 1777–1781 CrossRef CAS PubMed.
- H. R. Zare, N. Nasirizadeh and M. M. Ardakani, J. Electroanal. Chem., 2005, 577, 25–33 CrossRef CAS.
- S. S. Kumar, J. Mathiyarasu and K. L. Phani, J. Electroanal. Chem., 2005, 578, 95–103 CrossRef CAS.
- J. Premkumar and S. B. Khoo, J. Electroanal. Chem., 2005, 576, 105–112 CrossRef CAS.
- P. Kannan and S. Abraham John, Anal. Biochem., 2009, 386, 65–72 CrossRef CAS PubMed.
- S. Thiagarajan and S. M. Chen, Talanta, 2007, 74, 212–222 CrossRef CAS PubMed.
- J. X. Huang, Y. Liu, H. Q. Hou and T. Y. You, Biosens. Bioelectron., 2008, 24, 632–637 CrossRef CAS PubMed.
- C. F. Tang, S. A. Kumar and S. M. Chen, Anal. Biochem., 2008, 380, 174–183 CrossRef CAS PubMed.
- D. Wen, S. J. Guo, S. J. Dong and E. K. Wang, Biosens. Bioelectron., 2010, 26, 1056–1061 CrossRef CAS PubMed.
- G. Z. Hu, Y. G. Ma, Y. Guo and S. J. Shao, Electrochim. Acta, 2008, 53, 6610–6615 CrossRef CAS.
- Y. Chen, L. R. Guo, W. Chen, X. J. Yang, B. Jin, L. M. Zheng and X. H. Xia, Bioelectrochemistry, 2009, 75, 26–31 CrossRef CAS PubMed.
- C. R. Raj and T. Ohsaka, J. Electroanal. Chem., 2001, 496, 44–49 CrossRef CAS.
- G. Z. Hu, Y. Guo and S. J. Shao, Electroanalysis, 2009, 21, 1200–1206 CrossRef CAS.
- L. Qian, Q. Gao, Y. H. Song, Z. Li and X. R. Yang, Sens. Actuators, B, 2005, 107, 303–310 CrossRef CAS.
- Y. Z. Zhang, Y. Pan, S. Su, L. P. Zhang, S. P. Li and M. W. Shao, Electroanalysis, 2007, 19, 1695–1701 CrossRef CAS.
- G. R. Xu, Y. P. Zhang, J. Z. Tao, S. Y. Kim and Z. U. Bae, Electroanalysis, 2007, 19, 1085–1092 CrossRef CAS.
- X. Q. Lin, G. F. Kang and L. P. Lu, Bioelectrochemistry, 2007, 70, 235–244 CrossRef CAS PubMed.
- L. Zhang, C. H. Zhang and J. Y. Lian, Biosens. Bioelectron., 2008, 24, 690–695 CrossRef CAS PubMed.
- R. Zhang, G. D. Jin, D. Chen and X. Y. Hu, Sens. Actuators, B, 2008, 138, 174–181 CrossRef.
- H. Tang, G. Z. Hu, S. X. Jiang and X. Liu, J. Appl. Electrochem., 2009, 39, 2323–2328 CrossRef CAS.
- K. M. Manesh, P. Santhosh, A. Gopalan and K. P. Lee, Talanta, 2008, 75, 1307–1314 CrossRef CAS PubMed.
- W. Y. Su and S. H. Cheng, Electrochem. Commun., 2008, 10, 899–902 CrossRef CAS.
- W. Zheng, J. Li and Y. F. Zheng, Biosens. Bioelectron., 2008, 23, 1562–1566 CrossRef CAS PubMed.
- A. L. Liu, S. B. Zhang, W. Chen, X. H. Lin and X. H. Xia, Biosens. Bioelectron., 2008, 23, 1488–1495 CrossRef CAS PubMed.
- S. A. Kumar, C. F. Tang and S. M. Chen, Talanta, 2008, 74, 860–866 CrossRef CAS PubMed.
- P. R. Roy, T. Okajima and T. Ohsaka, Bioelectrochemistry, 2003, 59, 11–19 CrossRef CAS PubMed.
- P. F. Huang, L. Wang, J. Y. Bai, H. J. Wang, Y. Q. Zhao and S. D. Fan, Microchim. Acta, 2007, 157, 41–47 CrossRef CAS.
- M. Reza Hormozi Nezhad, J. Tashkhourian, J. Khodaveisi and M. Reza Khoshi, Anal. Methods, 2010, 2, 1263–1269 RSC.
- J. Li, J. Yang, Z. J. Yang, Y. F. Li, S. H. Yu, Q. Xu and X. Y. Hu, Anal. Methods, 2012, 4, 1725–1728 RSC.
- S. Y. Wang, W. Zhang, X. Zhong, Y. Q. Chai and R. Yuan, Anal. Methods, 2015, 7, 1471–1477 RSC.
- K. Y. Zhang, N. Zhang, J. G. Xu, H. Y. Wang, C. Wang, H. W. Shi and C. Liu, J. Appl. Electrochem., 2011, 41, 1419–1423 CrossRef CAS.
- J. Argüello, V. L. Leidens, H. A. Magosso, R. R. Ramos and Y. Gushikem, Electrochim. Acta, 2008, 54, 560–565 CrossRef.
- P. K. Aneesh, S. R. Nambiar, T. P. Rao and A. Ajayaghosh, Anal. Methods, 2014, 6, 5322–5330 RSC.
|
This journal is © The Royal Society of Chemistry 2018 |
Click here to see how this site uses Cookies. View our privacy policy here.