DOI:
10.1039/C8RA00524A
(Paper)
RSC Adv., 2018,
8, 8450-8454
Iridium-catalysed direct sulfamidation of quinazolinones†
Received
25th January 2018
, Accepted 3rd February 2018
First published on 23rd February 2018
Abstract
Ir-catalysed direct sulfamidation of quinazolinones has been achieved. A series of ortho-diamided quinazolinones were obtained in up to 96% yields. This transformation could proceed smoothly with a low catalyst loading under mild conditions with nitrogen released as the sole byproduct. This approach potentially provides an environmentally benign sulfamidation process for atom/step economic syntheses of useful pharmaceutical molecules or important building blocks.
Over the past two decades, C–H bond functionalization has attracted considerable attention for its outstanding advantages in atom efficiency and synthesis step efficiency compared to the traditional cross-coupling reactions.1 Prefunctionalization and multiple tedious purification steps can be avoided while enhancing the step economy of the transformation and decreasing the byproduct formation in these processes.2 Therefore, direct functionalization of C–H bond has become an increasingly attractive strategy for the synthesis and derivatization of natural products and pharmaceutically relevant building blocks.3 However, controlling site selectivity is one of the challenges in C–H bond activation. In this regard, the strategy involving regioselective C–H bond activation assisted by directing group shows high potential. So far, various directing groups containing oxygen or nitrogen atoms have been developed successfully. As is well-known, quinazolinones are a significant class of compounds because of their pharmaceutical applications and biological activities. In particular, quinazolinones have been found to be with various bioactivities, such as antibacterial,4 antifungal,5 antimalarial,6 anticancer,7 antihypertensive,8 antitubercular,9 and anticonvulsant.10 Forward this direction, our group has developed a series of easy methods in modification of quinazolinones via metal-catalysed C–H bond functionalization, in which quinazolinones act as directing groups since there are two N atoms in these structures.11 In addition, organic azide, as an internal oxidant and environmentally benign reagent, has been extensively explored in amidation reactions because nontoxic nitrogen gas is released as the only byproduct.12 Recently, our group has developed an Ir-catalysed amidation of benzaldehydes with sulfonyl azides as amino sources.13 In our continuing effort to develop green methods in C–H bond activation,14 herein, we disclose an Ir-catalysed direct amidation of 2-arylquinazolinones with sulfonyl azides as amino sources to produce ortho-diamided quinazolinones (Scheme 1), in which symmetrical di-amidated products was selectively obtained with high yields by controlling the amount of azide. This procedure could proceeded smoothly with a low catalyst loading and tolerate various substituent groups with N2 as the sole byproduct. The titled products are an important building block in organic synthesis and can be potentially converted into various highly valuable molecules via diverse transformations.
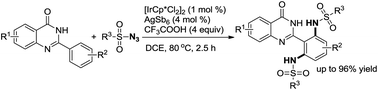 |
| Scheme 1 Ir-catalysed direct amidation of 2-arylquinazolinones. | |
Initially, the amidation of 2-phenylquinazolin-4-(3H)-one (1a) with para-toluenesulfonyl azide (2a) (3.0 equiv.) was chosen as a model reaction to examine the impact of various parameters on the reaction (Table 1). The results revealed that N,N′-(2-(4-oxo-3,4-dihydroquinazolin-2-yl)-1,3-phenylene)bis(4-methyl benzenesulfonamide) (3a) was obtained as a main product in 96% yield in DCE at 80 °C when [IrCp*Cl2]2 (1 mol%) was used as a catalyst with AgSbF6 (4 mol%), CF3COOH (4 equiv.) under air (Table 1, entry 1). We tried the first set of conditions displayed in entry 1 of Table 1 firstly, and found they are the optimum reaction conditions fortunately. When the loading amount of 2a was reduced to 2.0 or 1.0 equivalent, the reaction was complex and gave the mixture of the mono- (4a, Scheme 2a) and di-amidated products (3a). Only 75% and 60% yield of the target product 3a was achieved when the reaction was carried out under O2 and N2 protection (Table 1, entries 2–3). Other metal salts, such as Pd(OAc)2 and [RhCp*Cl2]2 were screened, showing that the reaction did not proceed without Ir catalyst and the yield of 3a was decreased when the loading of catalyst was reduced to 0.5 mol% (Table 1, entry 1 vs. entries 4–7). Moreover, AgSbF6 was shown to favor this reaction than other Ag salts (Table 1, entry 1 vs. entries 8–11). TFA was chosen to be better acid (Table 1, entry 1 vs. entries 12–14). On the other hand, DCE was demonstrated to be the best solvent (Table 1, entry 1 vs. entries 15–17). The yield of 3a decreased when the reaction temperature and reaction time were changed (Table 1, entries 18–21). Based on the results, under the optimum reaction conditions, the target product 3a was obtained in 96% yield in DCE at 80 °C when [IrCp*Cl2]2 (1 mol%) was used as a catalyst with AgSbF6 (4 mol%), CF3COOH (4 equiv.) under air (Table 1, entry 1).
Table 1 Optimization of the reaction conditionsa
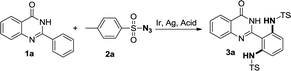
|
Entry |
Catalyst |
Silver |
Acid |
Solvent |
Temp (°C) |
t (h) |
Yieldb (%) |
Reaction conditions: 1a (0.20 mmol), 2a (0.60 mmol), Ir (1 mol%), Ag (4 mol%), acid (4.0 equiv.), solvent (2 mL). Isolated yields. O2. N2. Ir (0.5 mol%), Ag (2 mol%). nd = not detected. |
1 |
[IrCp*Cl2]2 |
AgSbF6 |
TFA |
DCE |
80 |
2.5 |
96 |
2c |
[IrCp*Cl2]2 |
AgSbF6 |
TFA |
DCE |
80 |
2.5 |
75 |
3d |
[IrCp*Cl2]2 |
AgSbF6 |
TFA |
DCE |
80 |
2.5 |
60 |
4e |
[IrCp*Cl2]2 |
AgSbF6 |
TFA |
DCE |
80 |
2.5 |
77 |
5 |
— |
AgSbF6 |
TFA |
DCE |
80 |
2.5 |
nd |
6 |
Pd(OAc)2 |
AgSbF6 |
TFA |
DCE |
80 |
2.5 |
nd |
7 |
[RhCp*Cl2]2 |
AgSbF6 |
TFA |
DCE |
80 |
2.5 |
nd |
8 |
[IrCp*Cl2]2 |
— |
TFA |
DCE |
80 |
2.5 |
nd |
9 |
[IrCp*Cl2]2 |
AgOAc |
TFA |
DCE |
80 |
2.5 |
40 |
10 |
[IrCp*Cl2]2 |
Ag2CO3 |
TFA |
DCE |
80 |
2.5 |
15 |
11 |
[IrCp*Cl2]2 |
AgNTf2 |
TFA |
DCE |
80 |
2.5 |
52 |
12 |
[IrCp*Cl2]2 |
AgSbF6 |
— |
DCE |
80 |
2.5 |
nd |
13 |
[IrCp*Cl2]2 |
AgSbF6 |
AcOH |
DCE |
80 |
2.5 |
nd |
14 |
[IrCp*Cl2]2 |
AgSbF6 |
PivOH |
DCE |
80 |
2.5 |
nd |
15 |
[IrCp*Cl2]2 |
AgSbF6 |
TFA |
DMF |
80 |
2.5 |
nd |
16 |
[IrCp*Cl2]2 |
AgSbF6 |
TFA |
THF |
80 |
2.5 |
nd |
17 |
[IrCp*Cl2]2 |
AgSbF6 |
TFA |
NMP |
80 |
2.5 |
nd |
18 |
[IrCp*Cl2]2 |
AgSbF6 |
TFA |
DCE |
90 |
2.5 |
86 |
19 |
[IrCp*Cl2]2 |
AgSbF6 |
TFA |
DCE |
70 |
2.5 |
70 |
20 |
[IrCp*Cl2]2 |
AgSbF6 |
TFA |
DCE |
80 |
3.0 |
88 |
21 |
[IrCp*Cl2]2 |
AgSbF6 |
TFA |
DCE |
80 |
2.0 |
81 |
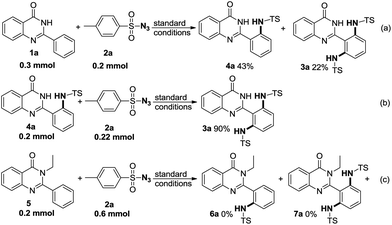 |
| Scheme 2 Exploration of the reaction mechanism. | |
With the optimized reaction conditions in hand, the scope of the substrates was examined (Table 2). First, toluenesulfonyl azide (2a) reacted smoothly with 2-phenylquinazolin-4-(3H)-one (1a) and its derivatives (1b–1l) to give 3a–3l in good to excellent yields (60–96%). Halogen groups, such as F, Cl, and methoxyl at the 5- or 6-position of quinazolinones provided the corresponding products 3b–3d in 86%, 78% and 92% yields, respectively, which indicated that the electron density on the moiety of the quinazolinones did not significantly influence the efficiency of the reaction. F group at the meta- and para-position of 2-phenyl in 2-aryl-quinazolin-4-(3H)-one provided the corresponding products 3e–3f in 65% and 94% yields. Other groups, such as Cl, Br, trifluoromethyl, methyl, t-butyl, and methoxyl could be well tolerated and gave the corresponding products in satisfactory yields (3g–3l) (60–96%). However, no product was obtained with functional groups such as –CN, –NO2, and –OH in 2-aryl-quinazolin-4-(3H)-one. 2-Phenylquinazolin-4-(3H)-one (1a) also reacted smoothly with substituted azides (2b–2d) to give the desired products 3m–3o in 80–89% yields. It can be seen that the electron density on the aromatic moiety of the azides did not significantly influence the efficiency of the reaction, and alkyl azide could also be tolerated in this procedure.
Table 2 Scope of substrates a,b
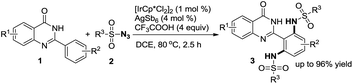
|
Entry |
1 |
2 |
3 |
Yieldb (%) |
Reaction conditions: 1 (0.20 mmol), 2 (0.6 mmol), Ir (1 mol%), Ag (4 mol%), TFA (4.0 equiv.), solvent (2 mL). Isolated yields. |
1 |
1a, R1 = H; R2 = H |
2a, R3 = 4-CH3C6H4 |
3a |
96 |
2 |
1b, R1 = 5-F; R2 = H |
2a, R3 = 4-CH3C6H4 |
3b |
86 |
3 |
1c, R1 = 6-Cl; R2 = H |
2a, R3 = 4-CH3C6H4 |
3c |
78 |
4 |
1d, R1 = 6-OCH3; R2 = H |
2a, R3 = 4-CH3C6H4 |
3d |
92 |
5 |
1e, R1 = H; R2 = 3-F |
2a, R3 = 4-CH3C6H4 |
3e |
65 |
6 |
1f, R1 = H; R2 = 4-F |
2a, R3 = 4-CH3C6H4 |
3f |
94 |
7 |
1g, R1 = H; R2 = 4-Cl |
2a, R3 = 4-CH3C6H4 |
3g |
60 |
8 |
1h, R1 = H; R2 = 4-Br |
2a, R3 = 4-CH3C6H4 |
3h |
87 |
9 |
1i, R1 = H; R2 = 4-CF3 |
2a, R3 = 4-CH3C6H4 |
3i |
96 |
10 |
1j, R1 = H; R2 = 4-CH3 |
2a, R3 = 4-CH3C6H4 |
3j |
70 |
11 |
1k, R1 = H; R2 = 4-t-Bu |
2a, R3 = 4-CH3C6H4 |
3k |
91 |
12 |
1l, R1 = H; R2 = 4-OCH3 |
2a, R3 = 4-CH3C6H4 |
3l |
90 |
13 |
1a, R1 = H; R2 = H |
2b, R3 = Ph |
3m |
83 |
14 |
1a, R1 = H; R2 = H |
2c, R3 = 4-CH3OC6H4 |
3n |
89 |
15 |
1a, R1 = H; R2 = H |
2d, R3 = CH3 |
3o |
80 |
To clarify the reaction mechanism, some control experiments were carried out (Scheme 2). First, 4-methyl-N-(2-(4-oxo-3,4-dihydroquinazolin-2-yl)phenyl)benzenesulfonamide (4a) and 3a were obtained in 43% and 22% yields when 1a (0.3 mmol) was treated with 2a (0.2 mmol) under the optimized conditions (Scheme 2a). Additionally, 4a (0.2 mmol) could react with 2a (0.22 mmol) to give the expected product 3a in 90% yield (Scheme 2b). To confirm which N atom of quinazolinones (1a) was coordinating with Ir(III), NH-protected quinazolinone (5) was introduced to the reaction. No target product (6a or 7a) was obtained (Scheme 2c). These results suggested that NH of quinazolinones (1a) played as a directing group in the catalytic cycle, and compound 4a was the key intermediate in this reaction.
Based on the results obtained and the literatures,15 a plausible reaction pathway for this Ir(III)-catalysed amidation reaction was proposed and shown in Scheme 3. Initially, the dimeric precursor [IrCp*Cl2]2 was converted into a cationic species A by the aid of silver salt. The five-membered iridacycle B with one vacant accessible site was formed by the coordination of the iridium atom with the nitrogen atom, and subsequently an electrophilic attack at the ortho-position carbon atom. Then, intermediate C was formed through interaction of azide with the cationic metal center. It was proposed that intermediate D from complex C occurred in an oxidative manner to release the N2 molecule. A new C–N bond was formed by insertion of the N–Ts imines species into a new iridacycle E. The compound E was protodemetalated to deliver the sulfamidated product 4a. Finally, the desired product 3a was generated via another same catalytic cycle from compound 4a.
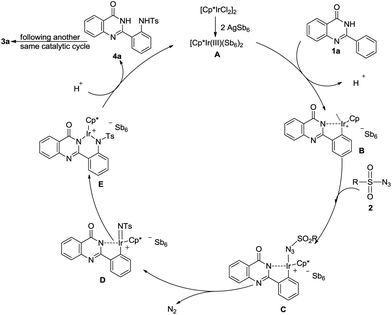 |
| Scheme 3 The proposed reaction mechanism. | |
Conclusions
In summary, we have demonstrated an Ir-catalysed direct amidation of 2-arylquinazolinones with sulfonyl azides as amino sources to produce a series of ortho-diamided quinazolinones. This transformation involves two C–N bonds formation with low catalyst loading under mild conditions and wide functional group tolerance. Moreover, this approach provided a fast-track strategy for atom/step economical syntheses of useful pharmaceutical molecules with N2 as the sole byproduct. The products obtained are important building blocks in organic synthesis and could be easily converted into various highly valuable molecules via diverse transformations. Further study on the application of this reaction is ongoing in our laboratory.
Conflicts of interest
There are no conflicts to declare.
Acknowledgements
This work was supported by the NSF of China (No. 21572072 and 21602064), Xiamen Southern Oceanographic Center (15PYY052SF01) and Y. F. thanks the Graduate Innovation Fund of Huaqiao University.
Notes and references
- For selected reviews see:
(a) M. P. Doyle and K. I. Goldberg, Acc. Chem. Res., 2012, 45, 777 CrossRef CAS PubMed;
(b) T. Bruckl, R. D. Baxter, Y. Ishihara and P. S. Baran, Acc. Chem. Res., 2012, 45, 826 CrossRef CAS PubMed;
(c) P. B. Arockiam, C. Bruneau and P. H. Dixneuf, Chem. Rev., 2012, 112, 5879 CrossRef CAS PubMed;
(d) C.-L. Sun, B.-J. Li and Z.-J. Shi, Chem. Rev., 2011, 111, 1293 CrossRef CAS PubMed;
(e) T. W. Lyons and M. S. Sanford, Chem. Rev., 2010, 110, 1147 CrossRef CAS PubMed;
(f) J. Yamaguchi, A. D. Yamaguchi and K. Itami, Angew. Chem., Int. Ed., 2012, 51, 8960 CrossRef CAS PubMed;
(g) D. A. Colby, R. G. Bergman and J. A. Ellman, Chem. Rev., 2010, 110, 624 CrossRef CAS PubMed;
(h) J. Wencel-Delord, T. Drcge, F. Liu and F. Glorius, Chem. Soc. Rev., 2011, 40, 4740 RSC;
(i) T. Satoh and M. Miua, Chem.–Eur. J., 2010, 16, 11212 CrossRef CAS PubMed;
(j) G. Y. Song, F. Wang and X. Li, Chem. Soc. Rev., 2012, 41, 3651 RSC;
(k) L. Ackermann and J. Pospech, Org. Lett., 2011, 13, 4153 CrossRef CAS PubMed;
(l) T. Ueyama, S. Mochida, T. Fukutani, K. Hirano, T. Satoh and M. Miura, Org. Lett., 2011, 13, 706 CrossRef CAS PubMed;
(m) H. Weissman, X. Song and D. Milstein, J. Am. Chem. Soc., 2001, 123, 337 CrossRef CAS PubMed;
(n) J. Wu, X. Cui, X. Mi, Y. Li and Y. Wu, Chem. Commun., 2010, 46, 6771 RSC;
(o) L. Ackermann, L. Wang and A. V. Lygin, Chem. Sci., 2012, 3, 177 RSC.
-
(a) W. Yang, J. Wang, Z. Wei, Q. Zhang and X. Xu, J. Org. Chem., 2016, 81, 1675 CrossRef CAS PubMed;
(b) S. E. Walker, J. A. Jordan-Hore, D. G. Johnson, S. A. Macgregor and A. L. Lee, Angew. Chem., Int. Ed., 2014, 53, 13876 CrossRef CAS PubMed;
(c) A. Honraedt, F. Le Callonnec, E. Le Grognec, V. Fernandez and F.-X. Felpin, J. Org. Chem., 2013, 78, 4604 CrossRef CAS PubMed;
(d) T. Zhou, L. Li, B. Li, H. Song and B. Wang, Org. Lett., 2015, 17, 4204 CrossRef CAS PubMed;
(e) Y. Fujiwara, V. Domingo, I. B. Seiple, R. Gianatassio, M. D. Del Bel and P. S. Baran, J. Am. Chem. Soc., 2011, 133, 3292 CrossRef CAS PubMed;
(f) S. Shaaban, A. Jolit, D. Petkova and N. Maulide, Chem. Commun., 2015, 51, 13902 RSC;
(g) G. A. Jardim, J. F. Bower and S. J. E. Da, Org. Lett., 2016, 18, 4454 CrossRef CAS PubMed;
(h) B.-J. Li and Z.-J. Shi, Chem. Soc. Rev., 2012, 41, 5588 RSC;
(i) N. Kuhl, N. Schroder and F. Glorius, Adv. Synth. Catal., 2014, 356, 1443 CrossRef CAS;
(j) J. Mo, L. Wang, Y. Liu and X. Cui, Synthesis, 2015, 47, 439 CrossRef CAS.
-
(a) L. Ackermann, R. Vicente and A. R. Kapdi, Angew. Chem., Int. Ed., 2009, 48, 9792 CrossRef CAS PubMed;
(b) D. Alberico, M. E. Scott and M. Lautens, Chem. Rev., 2007, 107, 174 CrossRef CAS PubMed;
(c) T. W. Lyons and M. S. Sanford, Chem. Rev., 2010, 110, 1147 CrossRef CAS PubMed;
(d) R. Giri, B.-F. Shi, K. M. Engle, N. Maugel and J.-Q. Yu, Chem. Soc. Rev., 2009, 38, 3242 RSC;
(e) L. McMurray, F. O'Hara and M. J. Gaunt, Chem. Soc. Rev., 2011, 40, 1885 RSC;
(f) M. A. Battiste, P. M. Pelphrey and D. L. Wright, Chem.–Eur. J., 2006, 12, 3438 CrossRef CAS PubMed;
(g) H. Butenschön, Angew. Chem., Int. Ed., 2008, 47, 5287 CrossRef PubMed;
(h) K. E. O. Ylijoki and J. M. Stryker, Chem. Rev., 2013, 113, 2244 CrossRef CAS PubMed;
(i) M. Harmata, Chem. Commun., 2010, 46, 8886 RSC.
- A. K. Nanda, S. Ganguli and R. Chakraborty, Molecules, 2007, 12, 2413 CrossRef CAS PubMed.
- J.-H. Chan, J.-S. Hong, L. F. Kuyper, D. P. Baccanari, S. S. Joyner, R. L. Tansik, C. M. Boytos and S. K. Rudolph, J. Med. Chem., 1995, 38, 3608 CrossRef CAS PubMed.
- H. Kikuchi, K. Yamamoto, S. Horoiwa, S. Hirai, R. Kasahara, N. Hariguchi, M. Matsumoto and Y. Oshima, J. Med. Chem., 2006, 49, 4698 CrossRef CAS PubMed.
-
(a) Y. Takase, T. Saeki, N. Watanabe, H. Adachi, S. Souda and I. Saito, J. Med. Chem., 1994, 37, 2106 CrossRef CAS PubMed;
(b) M. Dupuy, F. Pinguet, O. Chavignon, J. M. Chezal, J. C. Teulade, J. P. Chapat and Y. Blache, Chem. Pharm. Bull., 2001, 49, 1061 CrossRef CAS PubMed;
(c) P. M. Chandrika, T. Yakaiah, A. R. R. Rao, B. Narsaiah, N. C. Reddy, V. Sridhar and J. V. Rao, Eur. J. Med. Chem., 2008, 43, 846 CrossRef CAS PubMed.
- M.-H. Yen, J.-R. Sheu, I.-H. Peng, Y.-M. Lee and J.-W. J. Chern, Pharmacol. Pharm., 1996, 48, 90 CrossRef CAS.
-
(a) J. Kunes, J. Bazant, M. Pour, K. Waisser, M. Slosarek and J. Janota, Farmaco, 2000, 55, 725 CrossRef CAS PubMed;
(b) K. Waisser, J. Gregor, H. Dostal, J. Kunes, L. Kubicova, V. Klimesova and J. Kaustova, Farmaco, 2001, 56, 803 CrossRef CAS PubMed.
- A. Archana, V. K. Shrivastava, R. Chandra and A. Kumar, Indian J. Chem., Sect. B: Org. Chem. Incl. Med. Chem., 2002, 41, 2371 Search PubMed.
- Y. Feng, N. Tian, Y. Li, C. Jia, X. Li, L. Wang and X. Cui, Org. Lett., 2017, 19, 1658 CrossRef CAS PubMed.
-
(a) J. Kim and S. Chang, Angew. Chem., Int. Ed., 2014, 53, 2203 CrossRef CAS PubMed;
(b) T. Kang, Y. Kim, D. Lee, Z. Wang and S. Chang, J. Am. Chem. Soc., 2014, 136, 4141 CrossRef CAS PubMed;
(c) H. Hwang, J. Kim, J. Jeong and S. Chang, J. Am. Chem. Soc., 2014, 136, 10770 CrossRef CAS PubMed;
(d) C. Pi, X. Cui and Y. Wu, J. Org. Chem., 2015, 80, 7333 CrossRef CAS PubMed;
(e) B. Zhu, X. Cui, C. Pi, D. Chen and Y. Wu, Adv. Synth. Catal., 2016, 358, 326 CrossRef CAS;
(f) Y. Park, Y. Kim and S. Chang, Chem. Rev., 2017, 117, 9247 CrossRef CAS PubMed;
(g) D. Lee, Y. Kim and S. Chang, J. Org. Chem., 2013, 78, 11102 CrossRef CAS PubMed;
(h) T. Kang, H. Kim, J. G. Kim and S. Chang, Chem. Commun., 2014, 50, 12073 RSC;
(i) H. Kim, G. Park, J. Park and S. Chang, ACS Catal., 2016, 6, 5922 CrossRef CAS.
- Y. Li, Y. Feng, L. Xu, L. Wang and X. Cui, Org. Lett., 2016, 18, 4924 CrossRef CAS PubMed.
-
(a) Y. Li, M. Gao, L. Wang and X. Cui, Org. Biomol. Chem., 2016, 14, 8428 RSC;
(b) M. Gao, Y. Li, L. Xie, R. Chauvin and X. Cui, Chem. Commun., 2016, 52, 2846 RSC;
(c) M. Wei, L. Wang and X. Cui, Chin. Chem. Lett., 2015, 26, 1336 CrossRef CAS;
(d) L. Wang, Z. Yang, M. Yang, R. Zhang, C. Kuai and X. Cui, Org. Biomol. Chem., 2017, 15, 8302 RSC.
-
(a) J. Kim and S. Chang, Angew. Chem., Int. Ed., 2014, 53, 2203 CrossRef CAS PubMed;
(b) J. Kim and S. Chang, Chem.–Eur. J., 2013, 19, 7328 CrossRef CAS PubMed;
(c) J. Ryu, K. Shin, S. H. Park, J. Y. Kim and S. Chang, Angew. Chem., Int. Ed., 2012, 51, 9904 CrossRef CAS PubMed;
(d) T. Kang, Y. Kim, D. Lee, Z. Wang and S. Chang, J. Am. Chem. Soc., 2014, 136, 4141 CrossRef CAS PubMed;
(e) H. Hwang, J. Kim, J. Jeong and S. Chang, J. Am. Chem. Soc., 2014, 136, 10770 CrossRef CAS PubMed;
(f) C. Pi, X. Cui and Y. Wu, J. Org. Chem., 2015, 80, 7333 CrossRef CAS PubMed;
(g) K. Shin, H. Kim and S. Chang, Acc. Chem. Res., 2015, 48, 1040 CrossRef CAS PubMed;
(h) Y. Park, K. T. Park, J. G. Kim and S. Chang, J. Am. Chem. Soc., 2015, 137, 4534 CrossRef CAS PubMed;
(i) K. Shin, Y. Baek and S. Chang, Angew. Chem., Int. Ed., 2013, 52, 8031 CrossRef CAS PubMed;
(j) J. Y. Kim, S. H. Park, J. Ryu, S. H. Cho, S. H. Kim and S. Chang, J. Am. Chem. Soc., 2012, 134, 9110 CrossRef CAS PubMed;
(k) B. Zhu, X. Cui, C. Pi, D. Chen and Y. Wu, Adv. Synth. Catal., 2016, 358, 326 CrossRef CAS;
(l) L. Xu, L. Wang, Y. Feng, Y. Li, L. Yang and X. Cui, Org. Lett., 2017, 19, 4343 CrossRef CAS PubMed;
(m) Z. Qi, S. Yu and X. Li, Org. Lett., 2016, 18, 700 CrossRef CAS PubMed;
(n) Y. Li, Z. Qi, H. Wang, X. Yang and X. Li, Angew. Chem., Int. Ed., 2016, 128, 12056 CrossRef;
(o) K. Shin, H. Kim and S. Chang, Acc. Chem. Res., 2015, 48, 1040 CrossRef CAS PubMed;
(p) H. Kim, K. Shin and S. Chang, J. Am. Chem. Soc., 2014, 136, 5904 CrossRef CAS PubMed;
(q) J. Ryu, J. Kwak, K. Shin, D. Lee and S. Chang, J. Am. Chem. Soc., 2013, 135, 12861 CrossRef CAS PubMed.
Footnote |
† Electronic supplementary information (ESI) available: General information, experimental procedures, copies of 1H, 13C and 19F NMR spectra for products. See DOI: 10.1039/c8ra00524a |
|
This journal is © The Royal Society of Chemistry 2018 |
Click here to see how this site uses Cookies. View our privacy policy here.