DOI:
10.1039/C8RA03870H
(Paper)
RSC Adv., 2018,
8, 24203-24208
Cu-MOF: an efficient heterogeneous catalyst for the synthesis of symmetric anhydrides via the C–H bond activation of aldehydes†
Received
6th May 2018
, Accepted 14th June 2018
First published on 3rd July 2018
Abstract
In this paper, an efficient and straightforward synthetic approach for the preparation of a number of symmetric carboxylic anhydrides was reported using Cu2(BDC)2(DABCO) as an efficient heterogeneous catalyst via the C–H bond activation of aldehydes with excellent yields and simple work up. This C–H bond activation reaction appears simple and convenient, has a wide substrate scope and makes use of cheap, abundant, and easily available reagents. The Cu-MOF catalyst was recycled and reused four times without any loss of catalytic activity.
Introduction
Metal–organic frameworks (MOFs), which are also called coordination polymers,1 are synthesized from organic linkers and metal ions or clusters.2 Recently, MOFs have attracted much more attention due to their structural and chemical diversities and have become very popular in diverse research areas such as catalysis,3–5 drug delivery,6,7 gas storage,8,9 adsorption,10,11 chemical sensing,12 conversion13 etc.
In spite of their low thermal and chemical stabilities which have limited their applications to only those under mild conditions, MOFs have achieved more attention in chemical catalysis.14 Their efficient catalytic activities are caused by several parameters such as the existence of suitable functional groups on the organic linkages, the flexibility of the framework which facilities the acceptance of the guest molecule(s), the internal channel surface area etc.15–17 The active metal sites in MOFs are fully exposed and thus provide a high degree of metal dispersion. In addition, MOFs provide a highly versatile alternative to well-established porous materials by constructing various transition metal nodes and a wide variety of organic bridging ligands for specific catalytic applications.18 Among MOFs, Cu-MOFs have attracted more interest because of their outstanding catalytic activities, regular structural configuration and some dominant electrical properties.18
Anhydrides belong to the carboxylic acid derivatives and are very useful reagents in organic synthesis19 and various other reactions including the lactonization of silyl esters,20 asymmetric esterifications,21 the condensation reaction of free carboxylic acids and alcohols22 and specially in the preparation of peptides and drugs.23 Symmetric anhydrides are well known as advantageous acylating agents because they prevent by-product generation due to their symmetric nature.24 The main classical synthetic procedures for anhydride synthesis involve utilizing dehydrating agents such as phosgene, acid chloride, thionyl chloride, benzenesulfonyl chloride, and phosphorous pentoxide in equivalent amounts which generally need harsh reaction conditions, are usually expensive, have inaccessible reagents and catalysts, have a time-consuming work-up etc. (Scheme 1).25,26
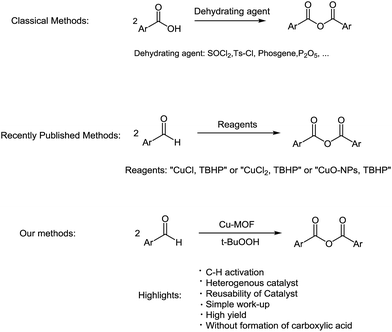 |
| Scheme 1 Different methods for the synthesis of anhydrides. | |
For these reasons, researchers are always looking for novel synthetic pathways which provide straightforward access to the desired anhydrides within a minimum reaction time with inexpensive starting materials and catalysts as well as mild reaction conditions.27–29 A new approach involves the self-coupling of aldehydes in the presence of transition metal catalysts such as copper.30–32 A major drawback related to earlier methodologies is homogeneous systems and as a result, the isolation of catalysts and work-up are difficult and time consuming. Therefore, the development of new heterogeneous systems for the formation of C–O bonds through C–H bond activation remains a great goal. A few examples were reported for C–O bond formation via C–H aldehyde bond activation.33 Corma34 recently published an example for C–O bond formation via C–H aldehyde bond activation with Cu-MOF as the heterogeneous catalyst.
So, according to what is mentioned above and in continuation of our interest in exploring the role of MOFs as efficient heterogeneous catalysts in organic synthesis and oxidative coupling reactions,35–37 herein, we report the first application of Cu-MOFs as efficient heterogeneous catalysts for the synthesis of symmetric carboxylic anhydrides 2 via the C–H activation of aldehydes 1 using TBHP as an oxidizing agent (Scheme 2). Good to excellent yields, easy work-up of the catalyst, reusability, and minimal reaction time are some of the beneficial features of this reaction.
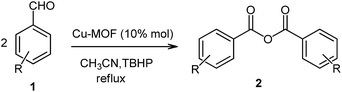 |
| Scheme 2 The Cu2(BDC)2(DABCO) catalyzed synthesis of anhydrides via C–H aldehyde bond activation. | |
Experimental section
Materials and methods
All of the chemicals were purchased from commercial sources and used without further purification. Cu2(BDC)2(DABCO) was synthesized according to a previously reported procedure.37 All of the reactions were monitored by thin layer chromatography (TLC) using plates coated with Merck 60 HF254 silica under UV light. The BET (Brunauer–Emmett–Teller) surface area of the samples was determined from N2 adsorption–desorption isotherms using a micromeritics ASAP 2020 analyzer. The sample was characterized using a ZEISS scanning electron microscope (SEM) at 30 kV with a gold coating. Transmission electron microscopy (TEM) was carried out using an EM10C-100 kV series microscope from the Zeiss Company, Germany. A TGA/DSC (thermogravimetric analysis & differential scanning calorimetry) by METTLER TOLEDO, Switzerland, was used for thermogravimetric analysis (TGA) with a heating rate of 10 °C min−1 under a nitrogen atmosphere. FT-IR spectra were recorded using a Shimadzu 8400s FT-IR spectrometer. 1H-NMR spectra were recorded with a BRUKER DRX 500-AVANCE FT-NMR instrument (CDCl3 solution) at 500 MHz.
Synthesis of carboxylic anhydrides via the C–H activation of aldehydes catalyzed by Cu2(BDC)2(DABCO)
To a solution of aldehyde 1 (1 mmol) and Cu2(BDC)2(DABCO) (10% mol) in CH3CN (2 ml), 1.5 eq. from a 6 M solution of TBHP in CH3CN was added slowly over 5 minutes while stirring. Then, the reaction temperature was increased to 80 °C and was allowed to be stirred for 2 h. The reaction progress was monitored by TLC. After completion of the reaction, the solvent was evaporated under reduced pressure, and the residue was purified using silica gel column chromatography (hexane/ethyl acetate (10
:
4)).
Selected spectra data
Benzoic anhydride (2a). Yield = 82%; liquid, 1H-NMR (500 MHz, CDCl3): δ 7.46–7.51 (m, 4H, CH of Ar), 7.60–7.65 (m, 2H, CH of Ar), 8.13 (d, 4H, 3J = 7.86 Hz). Anal. calcd for C14H10O3 (226.23): C, 74.33; H, 4.46. Found: C, 74.35; H, 4.47.
4-Nitrobenzoic anhydride (2i). Yield = 68%; mp: 188–190 °C, 1H-NMR (500 MHz, CDCl3): δ 8.14 (d, 4H, 3J = 8.9 Hz, CH of Ar), 8.26 (d, 4H, 3J = 8.9 Hz, CH of Ar). Anal. calcd for C14H8N2O7 (316.23): C, 53.18; H, 2.55; N, 8.86%. Found: C, 53.21; H, 2.58; N, 8.87%.
4-Hydroxy-3-methoxybenzoic anhydride (2m). Yield = 73%; 1H-NMR (500 MHz, CDCl3): δ 3.98 (s, 6H, MeO), 6.17 (bs, 2H, OH), 7.05 (d, 2H, 3J = 8.4 Hz, CH of Ar), 7.43–7.45 (m, 4H, CH of Ar). Anal. calcd for C16H14O7 (318.28): C, 60.38; H, 4.43. Found: C, 60.4; H, 4.46.
4-Hydroxybenzoic anhydride (2l). Yield = 70%; 1H-NMR (500 MHz, CDCl3): δ 5.6 (bs, 2H, OH), 6.95 (d, 4H, 3J = 8.6 Hz, CH of Ar), 7.82 (d, 4H, 3J = 8.6 Hz, CH of Ar). Anal. calcd for C14H10O5 (258.23): C, 65.12; H, 3.90. Found: C, 65.15; H, 3.94.
Results and discussion
In this work, Cu2(BDC)2(DABCO) was synthesized and characterized by various techniques and according to our previously reported procedure. The XRD powder patterns of the resulting solid were compared to the previously reported ones and sharp peaks were present at 8θ and 9θ, which confirmed the formation of Cu2(BDC)2(DABCO)37 (Fig. 2). The FT-IR spectra of Cu2(BDC)2(DABCO) exhibited the formation of Cu2(BDC)2(DABCO) compared to our previous reports37 (Fig. S5a†). The nitrogen adsorption isotherm of Cu2(BDC)2(DABCO) presented a typical type-I profile with a relatively high Langmuir surface area of 1496 m2 g−1 (Fig. S1†) and a median pore diameter of 3.9 nm (Fig. S1†). TGA results indicated that the material was stable up to over 250 °C (Fig. S6†). A SEM micrograph (Fig. S3†), consistent with the TEM observation (Fig. S4†), confirmed that a highly crystalline material was achieved.
The synthetic Cu2(BDC)2DABCO was employed as a catalyst in the C–H activation of aldehydes and the synthesis of anhydrides. Firstly, to achieve the optimum reaction conditions, various parameters such as the solvent, the oxidant, the amount of catalyst and the reaction time were examined.
Selection of the best solvent
The effect of various solvents such as dichloromethane (CH2Cl2), chloroform (THF), dimethylformamide (DMF), ethanol (C2H5OH), water (H2O) and acetonitrile (CH3CN) was investigated for the C–H activation of benzaldehyde in the presence of the catalyst and TBHP as a model reaction. The results are summarized in Table 1. It is obvious that the C–H activation of benzaldehyde is solvent-dependent. It can be seen that the maximum yield was obtained with the CH3CN solvent. So, CH3CN was chosen as an ideal solvent for this reaction.
Table 1 Solvent type optimization for the model reactiona
Entry |
Solvent |
Yielda (%) |
Reaction conditions: benzaldehyde (1 mmol), TBHP (1 mmol), catalyst (10 mol%) and solvent (2 ml). |
1 |
CH2Cl2 |
Trace |
2 |
THF |
10 |
3 |
DMF |
20 |
4 |
C2H5OH |
15 |
5 |
H2O |
25 |
6 |
CH3CN |
82 |
The effect of the amount of catalyst. For the investigation of the effect of the amount of catalyst on the C–H activation of aldehydes, as mentioned above the reaction of benzaldehyde in CH3CN as the solvent in the presence of TBHP was chosen as the model reaction. As shown in Table 2, 10 mol% of Cu-MOF was the best amount of catalyst for the synthesis of benzoic anhydride. The higher amounts of catalyst did not affect the reaction distinctly (Table 2, entries 5 and 6).
Table 2 Optimization of the amount of Cu2(BDC)2(DABCO) catalyst in the synthesis of benzoic anhydride
Entry |
Amount of catalyst (mol%) |
Yielda (%) |
Reaction conditions: benzaldehyde (1 mmol), TBHP (1 mmol) and CH3CN (2 ml). |
1 |
1 |
41 |
2 |
2.5 |
52 |
3 |
5 |
60 |
4 |
10 |
82 |
5 |
20 |
81 |
6 |
30 |
83 |
Selection of the best oxidant. For the optimization and selection of the best oxidant, air, TBHP, H2O2 and oxone were chosen and as shown in Table 3, entry 4, the best oxidant for this reaction was TBHP. It is noteworthy that when hydrogen peroxide was used as an oxidant a large part of aldehyde was converted to carboxylic acid and no product was observed.
Table 3 Effect of the type of oxidant in the synthesis of benzoic anhydride
Entry |
Oxidant |
Yielda (%) |
Reaction conditions: benzaldehyde (1 mmol), oxidant (1.5 mmol) and CH3CN (2 ml). |
1 |
Air |
— |
2 |
Oxone |
— |
3 |
H2O2 |
— |
4 |
TBHP |
82 |
After optimization of the model reaction, the reaction generality was evaluated using a vast number of aromatic aldehydes. The results are summarized in Table 4. The reaction presented good to excellent results using functionalized aromatic aldehydes with both electron-rich and electron-poor substituents. The results showed a high catalyst proficiency in C–H bond activation and the synthesis of anhydrides. Patel et al.32 have reported that aldehydes with electron-withdrawing groups such as NO2 in para or ortho positions in the presence of CuO nanoparticles resulted in no product being formed, while in the present work aldehydes with electron-withdrawing groups led to the corresponding anhydrides in relatively good yields. On the other hand, aldehydes with electron-donating groups such as vaniline, 4-methoxy benzaldehyde and 4-hydroxy benzaldehyde were converted to anhydrides with good to excellent yields.
Table 4 Generality investigation of the reaction through the synthesis of a vast number of asymmetric carboxylic anhydrides
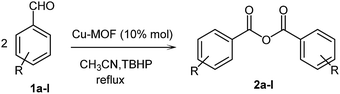
|
Entry |
R |
Anhydride |
Yielda (%) |
mp °C (ref.) |
Reaction conditions: aromatic aldehydes (1 mmol), TBHP (1.5 mmol) and time: 2 h. |
1a |
H |
2a |
82 |
Liquid [ref. 32] |
1b |
4-Me |
2b |
78 |
83–86 [ref. 32] |
1c |
4-MeO |
2c |
81 |
95–97 [ref. 32] |
1d |
4-Cl |
2d |
75 |
180–182 [ref. 26] |
1e |
4-Br |
2e |
79 |
218–220 [ref. 38] |
1f |
2-Br |
2f |
78 |
86–88 [ref. 41] |
1g |
2-Cl |
2g |
80 |
76–78 [ref. 39] |
1h |
3-Cl |
3h |
81 |
84–87 [ref. 41] |
1i |
4-NO2 |
2i |
68 |
188–190 [ref. 40] |
1j |
2-NO2 |
2j |
67 |
136–137 [ref. 41] |
1k |
2-Me |
2k |
76 |
Liquid [ref. 32] |
1l |
4-OH |
2l |
70 |
Oil |
1m |
4-OH, 3-MeO |
2m |
73 |
Oil |
1n |
3-Me |
2n |
78 |
Liquid [ref. 32] |
1o |
3-Meo |
2o |
80 |
Liquid [ref. 32] |
1p |
4-F |
2p |
78 |
112–114 [ref. 26b] |
1q |
2-Naphthaldehyde |
2q |
76 |
114–116 [ref. 32] |
In line with the results reported earlier by Saberi et al., and Khatun et al. the reaction of benzaldehyde in the presence of CuCl and CuCl2 and TBHP yielded 68 and 75% of the corresponding anhydrides, 2a, after 3 h, respectively (Table 5, entry 3 and 4). However, when CuCl and CuCl2 were replaced by Cu2(BDC)2DABCO, the reaction was considerably faster and afforded practically full conversion and full selectivity to 2a after 2 h (Table 5, entry 7). In addition, after the completion of the reaction, the catalyst was easily separated by filtration or by centrifuge and reused several times. As shown in Table 5, also, there are many other reports on the synthesis of anhydrides using aldehydes, but most of them have used an equivalent amount of reagents and some of them are expensive and environmentally destructive (Table 5, entry 1, 5 and 6). On the other hand, CuO particles displayed the worst catalytic performance (61% conversion), which indicates the crucial role of the microporous structure of Cu-MOF, which provides a higher availability of copper sites compared to the very low porosity structure of CuO32 (Table 5, entry 2). As a result, Cu-MOF is expected to have flexible and unsaturated coordination for aldehydes, which allows for the continuation of the reaction process and the avoidance of the reaction being blocked by very rigid coordination.42,43
Table 5 Comparison of the activity for different systems in the synthesis of benzoic anhydride
Entry |
Catalyst |
Reaction conditions |
Time |
Yield |
Ref. no. |
1 |
— |
CH2Cl2, TCCA, Et3N |
1 h |
98 |
26 |
2 |
CuO nanoparticle |
DCE, TBHP 120 °C |
5 h |
61 |
32 |
3 |
CuCl2 |
CH3CN, TBHP, 80 °C |
3 h |
68 |
30 |
4 |
CuCl |
DMSO, TBHP, rt |
1 h |
75 |
31 |
5 |
— |
TBHP, TBAI, CH3CN, 70 °C |
0.5 h |
87 |
28 |
6 |
— |
TBHP, TBAI, PhClO, 80 °C |
3 |
85 |
29 |
7 |
Cu2(BDC)2DABCO-MOF |
TBHP, CH3CN, 80 °C |
2 h |
82 |
Present work |
The proposed reaction mechanism is shown in Scheme 3.
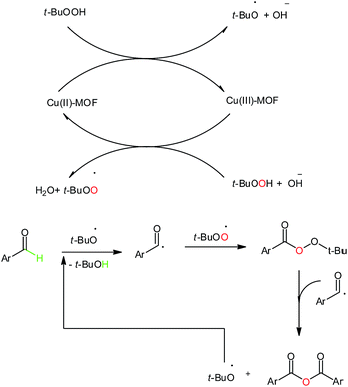 |
| Scheme 3 The proposed mechanism for the synthesis of symmetric carboxylic anhydrides via C–H activation. | |
In this reaction, firstly, electron transfer from an exposed Cu(II) at Cu-MOF to TBHP generates Cu(III) and tBuO˙. Then, Cu(III) eliminates an electron from the other TBHP molecule and gives Cu(II) and tBuOO˙ species. The activation of the hydrogen atom from aldehyde by tBuO˙ led to the formation of a benzoyl radical which coupled with tBuOO˙, subsequently. Then the cleavage of the O–O bond in the RCOOOtBu intermediate and it coupling with another benzoyl radical resulted in the corresponding anhydride and tBuo˙ species, which could be used in attracting the C–H bond of aryl-aldehyde.26
One of the important issues for heterogeneous catalysts is reusability. To investigate this issue for our catalyst, Cu-MOF was recovered by simple filtration and washed with methanol and dried in an oven. The recovered catalyst was reused for the C–H activation of benzaldehyde and the formation of benzoic anhydride four times. The results indicate that Cu2(BDC)2DABCO can be used for several cycles successfully with minimal loss of activity. As it is clear from the FESEM images, the morphology of the structure is not changed after 4 runs (Fig. 1).
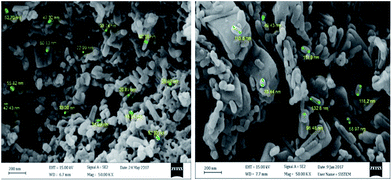 |
| Fig. 1 The FE-SEM image of fresh Cu2(BDC)2DABCO (left) and the FE-SEM image of recycled Cu2(BDC)2DABCO (right). | |
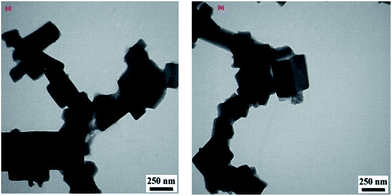 |
| Fig. 2 The TEM image of fresh Cu2(BDC)2(DABCO) (left) and the TEM image of recycled Cu2(BDC)2(DABCO) (right). | |
The TEM image of recycled Cu2(BDC)2DABCO shows that the crystalline structure of Cu2(BDC)2DABCO is maintained after 5 runs (Fig. 2).
Recycled Cu2(BDC)2DABCO after 5 runs maintained its crystallinity based on PXRD (Fig. 3), but a slight degradation of the structure and hydrolysis reaction44,45 could possibly justify the presence of the peaks at higher values of 2θ (30–40°), which probably indicates the presence of Cu(OH)2 or CuO,46 which are direct products of this reaction. In fact, under TBHP (70%) conditions, little water molecules are able to condense into the cavities of the material and it finally becomes energetically favorable for H2O to hydrolyze the Cu–O bond, breaking the crystalline structure.44,45
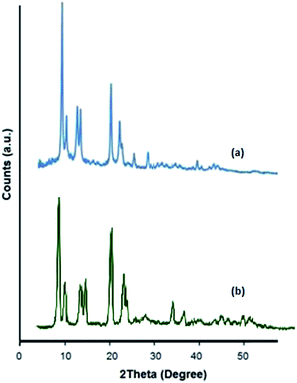 |
| Fig. 3 (a) The XRD pattern of fresh Cu2(BDC)2(DABCO). (b) The XRD pattern of recycled Cu2(BDC)2(DABCO). | |
Conclusion
In conclusion, we have studied a simple and effective method for the preparation of symmetric carboxylic anhydrides via C–H aldehyde bond activation catalyzed by Cu-MOF. In regards to the significant catalytic activity of Cu2(BDC)2(DABCO), a wide range of aromatic aldehydes with electron-donating groups and electron-withdrawing groups were converted to the corresponding anhydrides rapidly with good to excellent yields. Also, aldehydes with electron-withdrawing groups that had no yield in the methods reported in the literature were produced with relatively good efficiency using our method.
Conflicts of interest
There are no conflicts to declare.
Acknowledgements
We acknowledge the Science and Research Branch of Islamic Azad University for the partial financial support of this work.
References
- X. Hu, X. Lou, C. Li, Q. Chen, Q. Yang and B. Hu, New J. Chem., 2017, 41, 6415–6419 RSC.
- S. R. Batten, N. R. Champness, X. Chen, J. Garcia-Martinez, S. Kitagawa, L. Ohrstrom, M. O’Keeffe, M. P. Suh and J. Reedijk, CrystEngComm, 2012, 14, 3001–3004 RSC.
- S. M. Sadeghzadeh, R. Zhiani and S. Emrani, New J. Chem., 2018, 42, 988–994 RSC.
- F. Rouhani and A. Morsali, New J. Chem., 2017, 41, 15475–15484 RSC.
- Y. Takashima, M. Yokoyama, A. Horikoshi, Y. Sato, T. Tsuruoka and K. Akamatsu, New J. Chem., 2017, 41, 14409–14413 RSC.
- M.-X. Wu and Y.-W. Yang, Adv. Mater., 2017, 29, 3103–3121 Search PubMed.
- M. Al Haydar, H. R. Abid, B. Sunderland and S. Wang, Drug Des., Dev. Ther., 2017, 11, 2685–2695 CrossRef PubMed.
- B. Li, H. M. Wen, W. Zhou and B. Chen, J. Phys. Chem. Lett., 2014, 5, 3468–3479 CrossRef PubMed.
- S. Ma and H. C. Zhou, Chem. Commun., 2010, 46, 44–53 RSC.
- H. Furukawa, F. Gándara, Y. B. Zhang, J. Jiang, W. L. Queen, M. R. Hudson and O. M. Yaghi, J. Am. Chem. Soc., 2014, 136, 4369–4381 CrossRef PubMed.
- Z. Hu, B. J. Deibert and J. Li, Chem. Soc. Rev., 2014, 21, 5815–5840 RSC.
- L. E. Kreno, K. Leong, O. K. Farha, M. Allendorf, R. P. Van Duyne and J. T. Hupp, Chem. Rev., 2012, 112, 1105–1125 CrossRef PubMed.
- J. W. Maina, C. Pozo-Gonzalo, L. Kong, J. Schütz, M. Hill and L. F. Dumée, Mater. Horiz., 2017, 4, 345–361 RSC.
- M. Ghorbanlooa, V. Safarifard and A. Morsali, New J. Chem., 2017, 41, 3957–3965 RSC.
- J. Liu, L. Chen, H. Cui, J. Zhang, L. Zhang and C.-Y. Su, Chem. Soc. Rev., 2014, 43, 6011–6061 RSC.
- A. Dhakshinamoorthy and H. Garcia, Chem. Soc. Rev., 2014, 43, 5750–5765 RSC.
- A. Dhakshinamoorthy, M. Alvaro and H. Garcia, Catal. Sci. Technol., 2011, 1, 856–867 RSC.
- W. J. Shen, Y. Zhuo, Y. Chai and R. Yuan, Anal. Chem., 2015, 87, 11345–11352 CrossRef PubMed.
- M. A. Ogliaruso and J. F. Wolfe, Synthesis of Carboxylic Acids, Esters and Their Derivatives, John Wiley & Sons, New York, 1991, pp. 198–217 Search PubMed.
- T. Mukaiyama, J. Izumi, M. Miyashita and I. Shiina, Chem. Lett., 1993, 22, 907–910 CrossRef.
- I. Shiina, K. Nakata, K. Ono, Y. Onda and M. Itagaki, J. Am. Chem. Soc., 2010, 132, 11629–11641 CrossRef PubMed.
- I. Shiina, Tetrahedron, 2004, 59, 1587–1599 CrossRef.
-
(a) N. F. Albertson, Organic Reactions: Synthesis of Peptides with Mixed Anhydrides, John Wiley & Sons, Inc, 2011 Search PubMed;
(b) F. M. F. Chen, R. Stainauer and N. L. Benoiton, J. Org. Chem., 1983, 48, 2939–2941 CrossRef.
- J. Kim and D. O. Jang, Synth. Commun., 2001, 31, 395–399 CrossRef.
- C. Robert, F. de Montigny and Ch. M. Thomas, ACS Catal., 2014, 4, 3586–3589 CrossRef.
-
(a) S. Gaspa, A. Porcheddu and L. De Luca, Tetrahedron Lett., 2017, 58, 2533–2536 CrossRef;
(b) S. Gaspa, I. Amura, A. Porcheddu and L. D. Luca, New J. Chem., 2017, 41, 931–939 RSC.
- P. Chauhan, M. Ravi, R. Kant and P. Yadav, Org. Biomol. Chem., 2017, 15, 1080–1085 RSC.
- R. Singha, M. Ghosh, Y. Nuree and J. K. Ray, Tetrahedron Lett., 2016, 57, 1325–1327 CrossRef.
- M. Adib, R. Pashazadeh, S. Rajai-Daryasarei, P. Mirzaei and S. J. A. Gohari, Tetrahedron Lett., 2016, 57, 3071–3074 CrossRef.
- D. Saberi, F. Shojaeyan and K. Niknam, Tetrahedron Lett., 2016, 57, 566–569 CrossRef.
- Y. Nuree, R. Singha, M. Ghosh, P. Roy and J. K. Ray, Tetrahedron Lett., 2016, 57, 1479–1482 CrossRef.
- N. Khatun, S. K. Santra, A. Banerjee and B. K. Patel, Eur. J. Org. Chem., 2015, 1309–1313 CrossRef.
- S. Santoro, S. I. Kozhushkov, L. Ackermann and L. Vaccaro, Green Chem., 2016, 18, 3471–3493 RSC.
- I. Luz, A. Corma and F. X. L Xamena, Catal. Sci. Technol., 2014, 4, 1829–1836 RSC.
- S. Akbari, J. Mokhtari and Z. Mirjafary, RSC Adv., 2017, 7, 40881–40886 RSC.
- A. Khosravi, J. Mokhtari, M. R. Naimi-Jamal, S. Tahmasebi and L. Panahi, RSC Adv., 2017, 7, 46022–46027 RSC.
- L. Panahi, M. Reza Naimi-Jamal, J. Mokhtari and A. Morsali, Microporous Mesoporous Mater., 2017, 244, 208–217 CrossRef.
- Dictionary of Analytical Reagents, ed. A. Townshend, D. T. Burns, R. Lobinski, E. J. Newman, G. Guilbault, Z. Marczenko and H. Onishi, CRC Press Book, 1993, p. 175 Search PubMed.
- M. S. Newmann and J. A. Cella, J. Org. Chem., 1974, 39, 2084–2087 CrossRef.
- Y. L. Hu, X. E. Zhao and M. Lu, Bull. Chem. Soc. Ethiop., 2011, 25, 255–262 Search PubMed.
- Z. J. Kaminski, B. Kolesinska and M. Małgorzata, Synth. Commun., 2004, 34, 3349–3358 CrossRef.
- P. Valvekens, F. Vermoortele and D. De Vos, Catal. Sci. Technol., 2013, 3, 1435–1445 RSC.
- Y. Jiang, J. Huang, M. Hunger, M. Maciejewski and A. Baiker, Catal. Sci. Technol., 2015, 5, 897–902 RSC.
- P. M. Schoenecker, C. G. Carson, H. Jasuja, C. J. J. Flemming and K. S. Walton, Ind. Eng. Chem. Res., 2012, 51, 6513–6519 CrossRef.
- M. todaro, G. Buscarino, L. Sciortino, A. Alessi, F. Messina, M. Taddei, M. Ranocchiari, M. Cannas and F. M. Gelardi, J. Phys. Chem. C, 2016, 120, 12879–12889 CrossRef.
- N. Ba, L. Zhu, G. Zhang, J. Li and H. Li, Sens. Actuators, B, 2016, 227, 142–148 CrossRef.
Footnote |
† Electronic supplementary information (ESI) available. See DOI: 10.1039/c8ra03870h |
|
This journal is © The Royal Society of Chemistry 2018 |
Click here to see how this site uses Cookies. View our privacy policy here.