DOI:
10.1039/C8RA05243C
(Paper)
RSC Adv., 2018,
8, 28843-28846
Functionalization of silver nanoparticles with mPEGylated luteolin for selective visual detection of Hg2+ in water sample†
Received
19th June 2018
, Accepted 2nd August 2018
First published on 14th August 2018
Abstract
A novel colorimetric sensor based on mPEGylated luteolin-functionalized silver nanoparticles (mPEGylated luteolin-AgNPs) in an aqueous solution was prepared. The mPEGylated luteolin-AgNP solution was utilized to detect Hg2+ with high sensitivity and selectivity in the presence of other metal cations including Na+, K+, Mg2+, Zn2+, Ni2+, Mn2+, Ba2+, Pb2+, Sr2+, Ca2+, Cd2+, Al3+ and Cu2+. The solution could be induced to aggregate, and a color change from yellow-brown to colorless was observed in the presence of Hg2+. Meanwhile, the sensor was successfully used to detect Hg2+ in tap water with satisfactory recovery ranges using the standard addition method.
1. Introduction
As is widely known, Hg2+ is one of the most dangerous heavy metal pollutants in the environment because it can harm our brain, kidneys, and nervous and endocrine systems.1–3 Therefore, the development of effective methods to monitor Hg2+ in aqueous solutions is crucial. Generally, Hg2+ is determined using common analytical methods including inductively coupled plasma mass spectrometry,4 atomic absorption/emission spectroscopy,5 and atomic fluorescence spectrometry.6 However, these techniques are costly, time-consuming and complicated in terms of the sample preparation processes. Thus, developing a simple and sensitive method is currently a challenge. Metal nanoparticles, especially silver nanoparticles (AgNPs), have been of high interest in the sensor field because of their excellent optical properties.7,8 Until now, AgNPs have been widely used for the detection of Hg2+.9–12 It is known that colorimetric sensing based on the naked eye response cannot be replaced by other methods because of its online monitoring without the need of any sophisticated instruments. Recently, colorimetric sensors based on functionalized AgNPs have also attracted the attention of researchers.13–16
In our previous study, a facile route for the green synthesis of mPEGylated luteolin-capped silver nanoparticles (mPEGylated luteolin-AgNPs) was reported.17 This study mainly focused on the preparative and antimicrobial aspects of the system. Herein, mPEGylated luteolin-AgNPs were considered as a colorimetric sensor for the detection of Hg2+ in aqueous solutions. The mPEGylated luteolin-AgNP sensor was found to be highly sensitive and selective towards Hg2+ without any interference from other metal cations. Finally, the sensor was successfully used to detect Hg2+ in a tap water sample with satisfactory recovery ranges. The sensor exhibited high potential for practical applications due to its selective monitoring of Hg2+ in aqueous solutions.
2. Experimental
2.1. Materials and instrumentation
Silver nitrate (AgNO3), luteolin and mPEG (MW = 1900) were purchased from Sigma-Aldrich. Benzyl bromide, 4-toluene sulfonylchloride, cesium carbonate and anhydrous dimethyl formamide were purchased from J&K Chemical Technology (Beijing China). All the different cations including NaNO3, KNO3, Pb(NO3)2, Sr(NO3)2, Mg(NO3)2·6H2O, Zn(NO3)2·6H2O, Ni(NO3)2·6H2O, Ca(NO3)2·4H2O, Al(NO3)3·9H2O, Cd(NO3)2·4H2O, Mn(NO3)2·4H2O, Cu(NO3)2·3H2O, HgSO4 and BaCl2·2H2O were of analytical grade and were used without further purification; they were supplied by Sinopharm Chemical Reagent Co. Ltd (Shanghai, China). UV-Vis spectroscopic studies were carried out on a TU-1900 spectrophotometer (Beijing Purkinje General Instrument Co. Ltd.) with 1 cm quartz cell. The morphologies were studied on JEM-2100 (JEOL Co., Japan). The average size and zeta potential were recorded on Nano ZS 90 at 25 °C. The photographs of mPEGylated luteolin-AgNP solution used for visual colorimetric detection were taken with a digital camera.
2.2. Synthesis of the mPEGylated luteolin-AgNPs
As shown in Fig. S1,† the synthetic method of mPEGylated luteolin-AgNPs was in accordance with previously reported methods.17,18 The freshly prepared mPEGylated luteolin-AgNP solution was stored at 4 °C in a refrigerator.
2.3. The colorimetric determination of Hg2+
For colorimetric detection of Hg2+, the prepared mPEGylated luteolin-AgNPs were diluted with deionized water, and the resulting concentration was calculated to be 10−4 mol L−1. To study the metal ion detection ability of mPEGylated luteolin-AgNPs, 0.1 mL aliquots of representative metal cations (0.01 mol L−1) including Na+, K+, Mg2+, Zn2+, Ni2+, Mn2+, Hg2+, Ba2+, Pb2+, Sr2+, Ca2+, Cd2+, Al3+ and Cu2+ were added into the prepared mPEGylated luteolin-AgNP solution (2 mL) under similar conditions. After the solution had been mixed for 2 minutes, the changes in UV-Vis absorption spectra were monitored at room temperature. The photographs were also taken with a digital camera.
3. Results and discussion
3.1. The formation of mPEGylated luteolin-AgNPs
The redox potentials of luteolin and Ag+ were 0.35 and 0.779 V, respectively, which were easy to achieve by in situ synthesis.19 Here, Ag+ was easily reduced to Ag0 by two adjacent hydroxyls of mPEGylated luteolin; at the same time, dihydroxy underwent two-electron oxidation to quinine form. The prepared AgNPs were stabilized through electrostatic interactions and mPEG.
The prepared AgNP solution exhibited a yellow-brown color in aqueous solution, and the maximum absorbance value was detected at 440 nm by recording UV-Vis spectra at different time intervals (Fig. 1a). Fig. 1a shows that the absorbance intensity no longer increased after 30 minutes. The corresponding zeta potential of AgNPs showed a sharp peak at −25.5 mV (Fig. 1b), which indicated that the surface was negatively charged and the solution would be stable for a long period.20 Meanwhile the FT-IR spectrum (Fig. S2†) showed that the band at 3423 cm−1 corresponded to the O–H stretching vibration, and the bands at 1637 and 1738 cm−1 corresponded to the C
O stretching vibration. The above results proved that the surface of AgNPs was rich in hydroxyl and carbonyl groups.
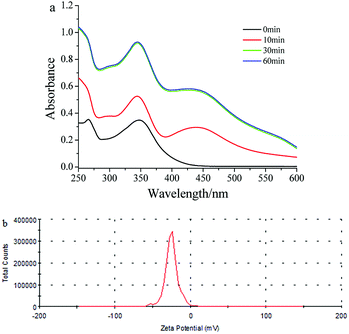 |
| Fig. 1 (a) UV-Vis spectra recorded during the formation of mPEGylated luteolin-AgNP solution at different time intervals. (b) Zeta potential stability measurements for mPEGylated luteolin-AgNPs. | |
The pH value was tuned between 2.0 and 10.0 using HCl or NaOH solution (0.01 mol L−1); then, the stability of the mPEGylated luteolin-AgNPs was studied. Fig. S3† shows the UV-Vis spectrum of the mPEGylated luteolin-AgNPs at different pH values (2, 4, 6, 7, 8 and 10). The changes in pH did not cause any visual change in the color, and there were some blue shifts in the peak positions at pH 2 and 4, which showed that the mPEGylated luteolin-AgNPs could be used in the pH range of 6–10.
3.2. Selective recognition of mPEGylated luteolin-AgNPs towards Hg2+
The UV-Vis spectra of mPEGylated luteolin-AgNP solution (2.0 mL) after the addition of fourteen varieties of metal ions in aqueous solution (0.1 mL, 0.01 mol L−1) are shown in Fig. 2. The color change from yellow-brown to colorless immediately in the presence of Hg2+, as observed in Fig. 2a, corresponded to disappearance of the absorption peak at 440 nm in Fig. 2b. The colorimetric response of mPEGylated luteolin-AgNPs to various metal ions is shown in Fig. 2c, and a clear increase in the ΔA value was observed in the presence of Hg2+. Here, ΔA is the UV absorption difference value between A0 and A. A0 was measured in the presence of only AgNPs, and A was measured in the presence of both AgNPs and various metal ions. Furthermore, as shown in Fig. S4,† the colorimetric detection ability of Hg2+ was studied in the presence of equal amounts of Hg2+ and other metal ions, and there was still no remarkable interference in color. All in all, the other metal ions had no clear effect on either the color or the UV-Vis spectra; thus, it was confirmed that mPEGylated luteolin-AgNPs were effectively selective for Hg2+.
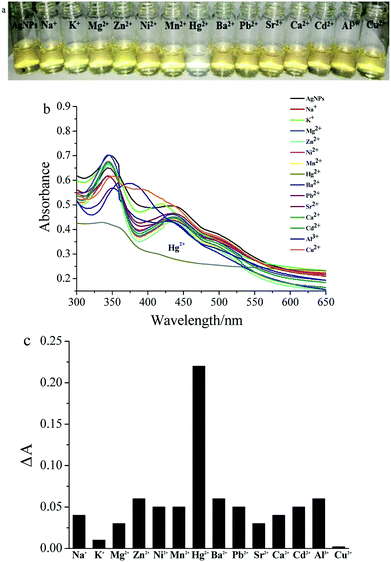 |
| Fig. 2 (a) The photographic images, (b) UV-Vis spectra of mPEGylated luteolin-AgNP solution in the presence of different metal ions; (c) the bar diagram exhibits the magnitude of change ΔA for various cations. | |
3.3. Interaction of mPEGylated luteolin-AgNPs with Hg2+
After addition of different concentrations of Hg2+ to an mPEGylated luteolin-AgNP solution, the solution color became clearer gradually until it turned colorless (Fig. 3a). Related UV-Vis spectra are shown in Fig. 3b, and the results showed that the absorption peak at 440 nm gradually decreased and finally disappeared.
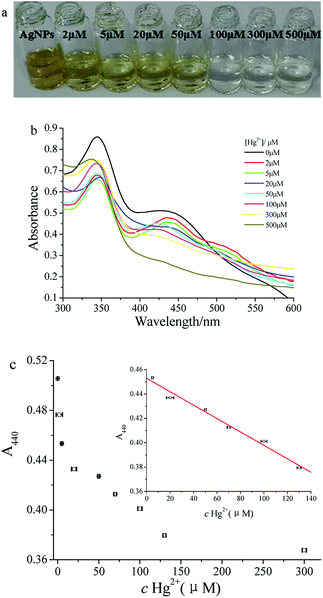 |
| Fig. 3 (a) Images, (b) UV-Vis spectra and (c) A440 of mPEGylated luteolin-AgNP solution with various concentrations of Hg2+. | |
To study the reaction mechanism between mPEGylated luteolin-AgNPs and Hg2+, TEM results of the mPEGylated luteolin-AgNPs in the presence of Hg2+ are shown in Fig. 4. Before the addition of Hg2+, mPEGylated luteolin-AgNPs were dispersed uniformly in aqueous solution. After the addition of Hg2+, the degree of mPEGylated luteolin-AgNP aggregation increased with the increasing Hg2+ concentration. As shown in Fig. S5a,† when the concentration of Hg2+ was in the range of 0–500 μM, the average size of mPEGylated luteolin-AgNPs increased from 77 to 170 nm, which was in good agreement with the TEM results.21 The aggregation phenomenon resulted in changes in not only color but also instability of the mPEGylated luteolin-AgNPs. As shown in Fig. S5b,† the absolute value of zeta potential decreased, which proved that the nanoparticles were increasingly unstable.
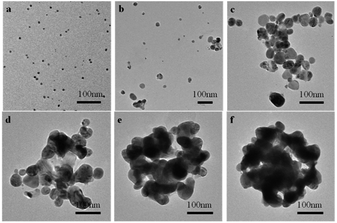 |
| Fig. 4 TEM images of the mPEGylated luteolin-AgNPs with various concentrations of Hg2+. (a) 0, (b) 2 μM, (c) 20 μM, (d) 100 μM, (e) 300 μM, (f) 500 μM. | |
To further discuss the possible reaction mechanisms, the 1HNMR spectra of mPEGylated luteolin-AgNPs in the absence and presence of Hg2+ were characterized (Fig. S6†). From Fig. S6,† it can be observed that the proton peaks on the benzene ring have basically disappeared in the presence of Hg2+, which showed that the mPEGylated luteolin-AgNPs possessed typical H donor ligand binding to Hg2+, and the possible mechanism is shown in Fig. 5. Moreover, an amalgam may be formed in this process.22
 |
| Fig. 5 Possible schematic representation of mPEGylated luteolin-AgNPs for detection of Hg2+. | |
3.4. Application of mPEGylated luteolin-AgNPs in tap water samples
Quantitative analysis of Hg2+ was studied by UV-Vis spectroscopy, which mainly monitored the color change of the solution. Typically, 0.1 mL aliquots of various concentrations of Hg2+ were added into 2.0 mL of mPEGylated luteolin-AgNP solution and mixed well for 2 minutes; then, A440 was tested (Fig. 3c). As shown in the inset of Fig. 3c, the calibration curve (A = 0.4529–5.509 × 10−4 c) was linearly proportional to the concentration of Hg2+ between 5 and 130 μM with a correlation coefficient of 0.9924. The detection limit for Hg2+ was 0.97 μM. Compared with some previously reported sensors (Table 1), the present colorimetric sensor showed more sensitivity and a wider linear range.
Table 1 Comparison of Hg2+ determination with previously reported results
Functionalization of AgNPs |
Linear range (μM) |
LOD (μM) |
Ref. |
mPEG-luteolin |
5–130 |
0.9 |
This work |
Garlic extract |
— |
2 |
23 |
Soap-root extract |
10–100 |
2.2 |
24 |
p-PDA |
0.99–9.09 |
0.8 |
16 |
Gln-His |
100–1000 |
25.48 |
25 |
Gln-His/NaCl |
1–500 |
0.9 |
To verify the feasibility of this method, a tap water sample was chosen for the determination of Hg2+. The standard addition method was employed for the calculation recovery, and the tap water sample was spiked with different known concentrations of Hg2+. The detected recovery rates for the prepared sensor ranged from 95.4% to 107.5% with RSD below 2%. These results indicated that our prepared sensor was reliable, feasible and sensitive to detect Hg2+ in water samples.
4. Conclusions
In summary, the mPEGylated luteolin-AgNP solution was first utilized for the detection of Hg2+. The Hg2+ concentration could be directly monitored using the color change of the mPEGylated luteolin-AgNP solution; this sensor is a novel colorimetric sensor for rapid and easy Hg2+ detection. In this study, the simple detection and good recovery of the proposed method were demonstrated, and it has potential applications in environmental and analytical chemistry fields.
Conflicts of interest
There are no conflicts to declare.
Acknowledgements
This work was supported by Program for Science & Technology Innovation Talents in Universities of Henan Province in China (No. 17HASTIT003), and Natural Science Foundation of Henan Province in China (No. 182300410235).
Notes and references
- J. Wang, X. Feng, C. Anderson, Y. Xing and L. Shang, J. Hazard. Mater., 2012, 221–222, 1 Search PubMed
. - E. M. Nolan and S. J. Lippard, Chem. Rev., 2008, 108, 3443 CrossRef PubMed
. - T. W. Clarkson, L. Magos and G. J. Myers, N. Engl. J. Med., 2003, 349, 1731 CrossRef PubMed
. - B. Fong, W. Mei, T. Siu, J. Lee, K. Sai and S. Tam, J. Anal. Toxicol., 2007, 31, 281 CrossRef PubMed
. - Q. Yang, Q. Tan, K. Zhou, K. Xu and X. Hou, J. Anal. At. Spectrom., 2005, 20, 760 RSC
. - J. Nevadoa, R. Martin-Doimeadiosb, F. Bernardo and M. Moreno, J. Chromatogr. A, 2005, 1093, 21 CrossRef PubMed
. - S. Bothra, R. Kumar, A. Kuwar, N. Singh and S. Sahoo, Mater. Lett., 2015, 145, 34 CrossRef
. - S. Bothra, R. Kumar, R. Pati, A. Kuwar, H. Choi and S. Sahoo, Spectrochim. Acta, Part A, 2015, 149, 122 CrossRef PubMed
. - Y. Zhang, I. D. Mckelvie, R. W. Cattrall and S. D. Kolev, Talanta, 2016, 152, 410 CrossRef PubMed
. - Y. Chen, L. Yao, Y. Deng, D. Pan, E. Ogabiela, J. Cao, S. B. Adeloju and W. Chen, Microchim. Acta, 2015, 182, 2147 CrossRef
. - J. Du, L. Jiang, Q. Shao, X. Liu, R. S. Marks, J. Ma and X. Chen, Small, 2013, 9, 1467 CrossRef PubMed
. - M. Annadhasan and N. Rajendiran, RSC Adv., 2015, 5, 94513 RSC
. - G. Maduraiveeran and R. Ramaraj, Anal. Methods, 2016, 8, 7966 RSC
. - L. Rastogi, R. Sashidhar, D. Karunasagar and J. Arunachalam, Talanta, 2014, 118, 111 CrossRef PubMed
. - S. Bothra, J. Solanki, S. Sahoo and J. Callan, RSC Adv., 2014, 4, 1341 RSC
. - S. Bothra, J. Solanki and S. Sahoo, Sens. Actuators, B, 2013, 188, 937 CrossRef
. - W. Qing, Y. Wang, X. Li, M. Lu and X. Liu, Colloids Surf., B, 2017, 160, 390 CrossRef PubMed
. - W. Qing, Y. Wang, H. Li, J. Zhu and X. Liu, RSC Adv., 2016, 6, 95812 RSC
. - A. Franzoi, I. Vieira, J. Dupont, C. Scheeren and L. liveira, Analyst, 2009, 134, 2320 RSC
. - K. Prabakara, P. Sivalingam, S. Mohamed Rabeeka, M. Muthuselvam, N. Devarajan, A. Arjunan, R. Karthick, M. Sureshd and J. Wembonyama, Colloids Surf., B, 2013, 104, 282 CrossRef PubMed
. - G. Nsengiyuma, R. Hu, J. Li, H. Li and D. Tian, Sens. Actuators, B, 2016, 236, 675 CrossRef
. - D. Sahu, N. Sarkar, G. Sahoo, P. Mohapatra and S. K. Swain, Sens. Actuators, B, 2017, 246, 96 CrossRef
. - S. Ghosh, S. Maji and A. Mondal, Colloids Surf., A, 2018, 555, 324 CrossRef
. - K. Farhadi, M. Forough, R. Molaei, S. Hajizadeh and A. Rafipour, Sens. Actuators, B, 2012, 161, 880 CrossRef
. - P. Buduru, B. Reddy and N. Naidu, Sens. Actuators, B, 2017, 244, 972 CrossRef
.
Footnote |
† Electronic supplementary information (ESI) available. See DOI: 10.1039/c8ra05243c |
|
This journal is © The Royal Society of Chemistry 2018 |
Click here to see how this site uses Cookies. View our privacy policy here.