DOI:
10.1039/C8RA05311A
(Paper)
RSC Adv., 2018,
8, 26828-26836
Copper-catalyzed C–S direct cross-coupling of thiols with 5-arylpenta-2,4-dienoic acid ethyl ester†
Received
21st June 2018
, Accepted 19th July 2018
First published on 27th July 2018
Abstract
A selective copper (Cu)-catalyzed C–S bond direct cross-coupling of thiols with 5-arylpenta-2,4-dienoic acid ethyl ester was developed. Notably, various biologically active 5-phenyl-3-phenylsulfanylpenta-2,4-dienoic acid ethyl ester derivatives were efficiently synthesized under moderate conditions. Finally, a plausible Cu(I)/Cu(III) reaction mechanism was proposed.
1 Introduction
As one of the most important compounds, organic thioethers are being widely applied in organic synthesis, the pharmaceutical industry, and functional materials.1 C–H bonds functionalization has considerably progressed.2 In theory, due to larger atomic radius and higher electron density, sulfur has more reactivity and is easy to modify (Scheme 1).3 In comparison with the state art of C–C coupling,4 acylation5 and amination,6 C–H bond direct thiolation has been seldom described in the literature. The main reason is that sulfur easily poisons transition metals.7 Therefore, developing more efficient strategies for C–H bond thiolation is still required.
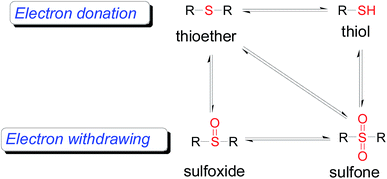 |
| Scheme 1 Conversions of sulfur-containing groups. | |
Selective C–H bond functionalization, either chemoselective or regioselective, has been long pursued.8 The progress on transition-metal catalyzed C–N and C–O cross-coupling has been prompted. However C–H bonds activated by alkenes is rarely reported.9 Considering the significance of diversifying synthetic strategies, we focused our interest on sulfur-directed C–H bond functionalization.10 5-Arylpenta-2,4-dienoic acid ethyl esters is an excellent scaffold which ubiquitous in natural biological products, the pharmaceutical chemistry and functionalized materials.11 However, the earlier reported synthetic methods have many disadvantages, such as low efficiency, inconvenience, and requiring harsh conditions. Efficient synthetic methods of 5-phenylpenta-2,4-dienoic acid ethyl esters are still required. Due to the functional group tolerance and economic attractiveness, copper catalysts have been extensively used in C–H bonds functionalization.12 Herein, we report a selective copper-catalyzed C–S bond direct cross-coupling reaction of thiols with 5-arylpenta-2,4-dienoic acid ethyl ester. In this reaction, various biological activity 5-aryl-3-arylsulfanylpenta-2,4-dienoic acid ethyl ester derivatives were efficiently synthesized under moderate conditions. Finally, a plausible reaction mechanism was proposed.
2 Results and discussion
The reaction conditions were screened based on a model reaction of thiophenol 1a with 5-arylpenta-2,4-dienoic acid ethyl ester 2a. These reactions are mainly based on the use of the enaminone ligand, which was previously discovered in our laboratory as an effective ligand for the C–N coupling of Ullmann reactions between aryl halides and various azoles.10 At the beginning, various structurally similar enaminone ligands L1–L9 were investigated (Scheme 2). The yields increased by changing the substituent R to R′. Additionally, other enaminone ligands, such as L7, L8, and L9, were observed to be less effective. Analyzing the results, L4 was considered the best ligand.
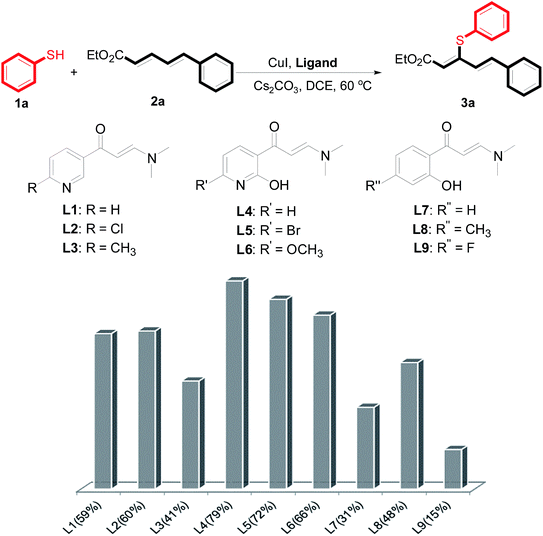 |
| Scheme 2 Ligand performance in copper-catalyzed C–S direct cross coupling. | |
Furthermore, other reaction parameters were optimized (Table 1). Experimental results demonstrated that the Cu(I) salt resulted in a higher yield than the Cu(II) salt (entries 1–4). Additionally, the results also demonstrated that the reaction temperature was as an important parameter. The desired product had a 63% yield at 50 °C (entry 8) and a 75% yield at 70 °C (entry 9). Furthermore, the reaction in the absence of the ligand did not occur (entry 11). Finally, the desired product 3a was formed with 81% yield when used the catalyst system L4 with CuI at 60 °C (entry 10).
Table 1 Optimization of the model reactiona
Entry |
Copper salt |
Base |
1a : 2a |
3a b(%) |
Unless otherwise noted, reactions conditions were 1a (0.3 mmol), copper source (10 mol%), L5 (10 mol%), Cs2CO3 (2 equiv.), DCE (4 mL), 60 °C for 24 h, in N2. Isolated yield. At 50 °C. At 70 °C. Absence of the ligand. |
1 |
Cu(OAc)2 |
Cs2CO3 |
1 : 1 |
15 |
2 |
CuSO4 |
Cs2CO3 |
1 : 1 |
42 |
3 |
CuBr2 |
Cs2CO3 |
1 : 1 |
NR |
4 |
CuBr |
Cs2CO3 |
1 : 1 |
53 |
5 |
CuI |
Cs2CO3 |
1 : 1 |
79 |
6 |
CuI |
Na2CO3 |
1 : 1.2 |
NR |
7 |
CuI |
K3PO4 |
1 : 1.2 |
41 |
8 |
CuI |
Cs2CO3 |
1 : 1.2 |
63c |
9 |
CuI |
Cs2CO3 |
1 : 1.2 |
75d |
10 |
CuI |
Cs2CO3 |
1 : 1.2 |
81 |
11 |
CuI |
Cs2CO3 |
1 : 1.2 |
NRe |
Under the optimized conditions, the reaction scope was next investigated. A wide array of aryl thiols 1 and 5-arylpenta-2,4-dienoic acid ethyl esters 2 were obtained as the productivity with good to excellent yields (Table 2). We found that both the electron-donating and electron-withdrawing aryl thiols 1 reacted smoothly with 5-arylpenta-2,4-dienoic acid ethyl esters 2. The aryl thiols 1 bearing electron-donating groups showed better activity than those with electron-withdrawing groups. 5-Arylpenta-2,4-dienoic acid ethyl esters 2 bearing electron-withdrawing groups showed better activity than those bearing electron-donating groups. The C
C configuration of the 5-arylpenta-2,4-dienoic acid ethyl esters 2 was retained in the corresponding products.
Table 2 Copper-catalyzed C–S direct cross-coupling of aryl thiols with 5-arylpenta-2,4-dienoic acid ethyl estera
Next, we focused on other thiols (Table 3). Aliphatic thiols worked well in this reaction. The corresponding products were isolated with 76–88% yields. The C
C configuration of the 5-phenylpenta-2,4-dienoic acid ethyl esters 2 were also retained in corresponding products.
Table 3 Copper-catalyzed C–S direct cross-coupling of aliphatic thiols with 5-arylpenta-2,4-dienoic acid ethyl estera
There were two reasons of the ester group essential for the regioselectivity in those reactions. First, ethyl ester was more common and more meaningful than other alkyl esters in the field of synthetic industry and functional materials. Secondly, ethyl ester had better coordination ability than other alkyl ester. Based on the above results, a reaction mechanism was proposed (Scheme 3). After the coordination of CuI with L4, a corresponding intermediate 6 was generated.13 Next, an intermediate 7 was formed from intermediate 6 with aryl thiols through a ligand exchange step. Next, intermediate 7 reacted with 5-arylpenta-2,4-dienoic acid ethyl esters to produce intermediate 8 via an intermolecular oxidative addition. Finally, intermediate 8 furnished the desired product 3 and concomitantly generated intermediate 6, which re-entered the catalytic cycle. Furthermore, the specific reaction mechanism is still under study via high-resolution electrospray ionisation mass spectrometry (HR-ESI-MS) and density functional theory (DFT).
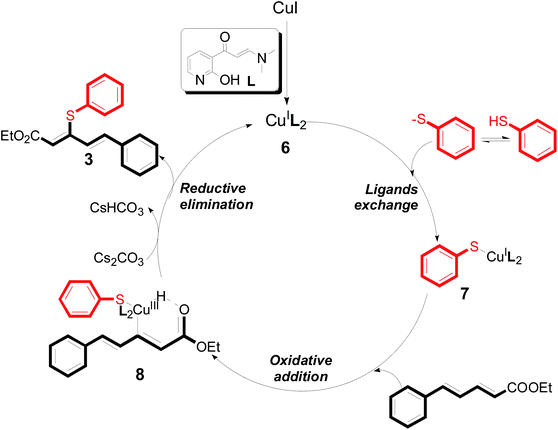 |
| Scheme 3 A plausible reaction mechanism. | |
3 Conclusions
In conclusion, a selective copper-catalyzed C–S bond direct cross-coupling of thiols with 5-arylpenta-2,4-dienoic acid ethyl esters was developed. Using this methodology, various biological activity 5-aryl-3-phenylsulfanylpenta-2,4-dienoic acid ethyl ester derivatives were efficiently synthesized. The process used inexpensive catalysts and under mild conditions. The reaction mechanism of the Cu(I)/Cu(III) catalysis cycle was proposed.
4 Experimental section
4.1. General procedure for preparation of L1–L10
Dimethylformamide dimethylacetal (DMFDMA) (10 mmol, 1.19 g) and 1-pyridin-3-yl-ethanone (10 mmol, 1.21 g) were dissolved in p-xylene (2 mL). And the mixture was refluxed during a period of 5 to 12 hours, during which time the formation of yellow precipitate. The precipitate was filtered out and washed with petroleum ether three times. The solid was vacuum-dried, and 1.65 g (yield 94%) of a yellow solid was obtained L1 3-dimethylamino-1-pyridin-3-yl-propenone. 1H NMR (500 MHz, d6-DMSO): δ 14.51 (s, 1 H), 7.92–7.90 (t, J = 7.5 Hz, 2 H), 7.37–7.34 (s, J = 7.8 Hz, 1 H), 6.83 (d, J = 2.0 Hz, 1 H), 5.98–5.95 (d, J = 12 Hz, 1 H), 3.19 (s, 3 H), 2.98 (s, 3 H); 13C NMR (125 MHz, d6-DMSO): δ 191.1, 163.6, 134.9, 129.9, 121.2, 119.1, 118.7, 90.4, 46.1, 38.6.
4.2. General procedure for preparation of 3 and 5
A mixture of benzenethiol 1a (33.0 mg, 0.3 mmol), 5-phenylpenta-2,4-dienoic acid ethyl ester 2a (72.7 mg, 0.36 mmol), CuI (5.7 mg, 10 mol%), 3-dimethylamino-1-(2-hydroxypyridin-3-yl)-propenone L4 (5.8 mg, 10 mol%) and Cs2CO3 (195.6 mg, 2 equiv.) in DMSO (4 mL) was stirred under a N2 atmosphere. After the reaction mixture was stirred at 60 °C for 24 h, it was allowed to cool to ambient temperature. Then the mixture was quenched with saturated salt water (10 mL), and the solution was extracted with ethyl acetate (3 × 10 mL). The organic layers were combined and dried by sodium sulfate and concentrated in vacuo. The pure product 5-phenyl-3-phenylsulfanylpenta-2,4-dienoic acid ethyl ester 3a (75.3 mg, 81% yield) was obtained by flash column chromatography on silica gel.
4.2.1 5-Phenyl-3-phenylsulfanylpenta-2,4-dienoic acid ethyl ester (3a). 75.3 mg, 81% yield; yellow soild; mp 111–113 °C; 1H NMR (500 MHz, CDCl3): δ 8.41 (dd, 1 H, J = 15.9 Hz, 0.8 Hz), 8.20 (d, 2 H, J = 8.9 Hz), 7.57 (d, 2 H, J = 8.9 Hz), 7.50–7.55 (m, 2 H), 7.30–7.43 (m, 4 H), 6.73 (m, 1 H), 5.90 (m, 1 H), 4.23 (q, 2 H), 1.35 (t, 3 H); 13C NMR (125 MHz, CDCl3): 160.8 (C), 145.9 (C), 142.8 (C), 140.7 (C), 136.9 (CH), 133.7 (CH), 129.0 (CH), 127.5 (CH), 126.8 (CH), 125.8 (CH), 122.4 (CH), 121.3 (CH), 120.1 (CH), 58.6 (CH2), 11.3 (CH3); ESI-HRMS m/z: calcd for C19H19O2S+ [M + H]+: 311.1100; found 311.0997.
4.2.2 3-Cyclohexylsulfanyl-5-(3,4-dimethoxyphenyl)penta-2,4-dienoic acid ethyl ester (5a). 99.4 mg, 88% yield; yellow oil; 1H NMR (500 MHz, CDCl3): δ 8.19 (dd, 1 H, J = 16.1, 0.8 Hz), 7.25 (d, 1 H, J = 16.1 Hz), 7.05–7.13 (m, 2 H), 6.84 (d, 1 H, J = 12.3 Hz), 5.71 (s, 1 H), 4.19 (q, 2 H), 3.92 (s, 3 H), 3.90 (s, 3 H), 3.14–3.25 (m, 1 H), 2.02–2.14 (m, 2 H), 1.76–1.86 (m, 2 H), 1.60–1.70 (m, 1 H), 1.37–1.54 (m, 5 H), 1.26–1.36 (m, 3 H); 13C NMR (125 MHz, CDCl3): δ 165.5 (C), 154.0 (C), 150.0 (C), 149.1 (C), 136.1 (CH), 129.5 (C), 123.1 (CH), 121.4 (CH), 111.4 (CH), 111.1 (CH), 109.7 (CH), 59.9 (CH2), 55.94 (CH3), 55.91 (CH3), 44.0 (CH), 32.7 (CH2), 26.0 (CH2) 25.9 (CH2), 14.5 (CH3); ESI-HRMS m/z: calcd for C21H29O4S+ [M + H]+: 377.1781; found 377.1778.
Conflicts of interest
There are no conflicts to declare.
Acknowledgements
This work was financially supported by the National Natural Science Foundation of China (Grant No. 21702186). We also thank Analysis and Test Center of Zhejiang Agriculture and Forestry University providing instruments for analysis.
References
-
(a) M. N. Birkholz, Z. Freixa and P. W. N. M. van Leeuwen, Bite angle effects of diphosphines in C-C and C-X bond forming cross coupling reactions, Chem. Soc. Rev., 2009, 38, 1099–1118 RSC;
(b) I. P. Beletskaya and V. P. Ananikov, Transition-metal-catalyzed C-S, C-Se, and C-Te bond formation via cross-coupling and atom-economic addition reactions, Chem. Rev., 2011, 111, 1596–1636 CrossRef PubMed;
(c) Y. J. Lian, R. G. Bergman, L. D. Lavis and A. E. Jonathan, Rhodium (III)-catalyzed indazole synthesis by C-H bond functionalization and cyclative capture, J. Am. Chem. Soc., 2013, 135, 7122–7125 CrossRef PubMed.
-
(a) A. Wangweerawong, R. G. Bergman and J. A. Ellman, Asymmetric synthesis of α-branched amines via Rh (III)-catalyzed C-H bond functionalization, J. Am. Chem. Soc., 2014, 136, 8520–8523 CrossRef PubMed;
(b) T. Besset, T. Poisson and X. Pannecoucke, Recent progress in direct introduction of fluorinated groups on alkenes and alkynes by means of C-H bond functionalization, Chem.–Eur. J., 2014, 20, 16830–16845 CrossRef PubMed;
(c) H. Fei and S. M. Cohen, Metalation of a thiocatechol-functionalized Zr(IV)-based metal-organic framework for selective C-H functionalization, J. Am. Chem. Soc., 2015, 137, 2191–2194 CrossRef PubMed.
-
(a) K. Pericherla, A. Jha and B. Khungar, Copper-catalyzed tandem azide-alkyne cycloaddition, Ullmann type C-N coupling, and intramolecular direct arylation, Org. Lett., 2013, 15, 4304–4307 CrossRef PubMed;
(b) C. Lin, D. Li and B. Wang, Direct ortho-thiolation of arenes and alkenes by nickel catalysis, Org. Lett., 2015, 17, 1328–1331 CrossRef PubMed;
(c) Z. H. Yang, Y. An, L. Y. Chen, Z. Y. Shao and S. Y. Zhao, Copper(I) iodide-catalyzed sulfenylation of maleimides and related 3-indolylmaleimides with thiols, Adv. Synth. Catal., 2016, 358, 3869–3875 CrossRef;
(d) K. Liao, F. Zhou, J. S. Yu, W. M. Gao and J. Zhou, Catalytic asymmetric sulfenylation to structurally diverse dithioketals, Chem. Commun., 2015, 51(90), 16255–16258 RSC;
(e) J. S. Yu, H. M. Huang, P. G. Ding, X. S. Hu, F. Zhou and J. Zhou, Catalytic enantioselective construction of sulfur-containing tetrasubstituted carbon stereocenters, ACS Catal., 2016, 6, 5139–5144 Search PubMed.
-
(a) J. K. Cheng and T. P. Loh, Copper- and cobalt-catalyzed direct coupling of sp3 α-carbon of alcohols with alkenes and hydroperoxides, J. Am. Chem. Soc., 2014, 137, 42–45 CrossRef PubMed;
(b) L. Guo, C. C. Hsiao, H. Yue, X. Liu and M. Rueping, Nickel-catalyzed Csp2-Csp3 cross-coupling via C-O bond activation, ACS Catal., 2016, 6, 4438–4442 CrossRef.
-
(a) S. Sharma, M. Kim, J. Park, M. Kim, J. H. Kwak, Y. H. Jung, J. S. Oh, Y. Lee and I. S. Kim, Palladium-catalyzed direct acylation of ketoximes and aldoximes from the alcohol oxidation level through C-H bond activation, Eur. J. Org. Chem., 2013, 6656–6665 CrossRef;
(b) F. Jafarpour, H. Hazrati and M. Darvishmolla, Acylation of pyrroles and their free (N-H)-derivatives via palladium-catalyzed carbopalladation of nitriles, Adv. Synth. Catal., 2014, 356, 3784–3788 CrossRef.
-
(a) N. Jiao, C. Tang, M. Zou, J. Liu, X. Wen, X. Sun and Y. Zhang, Rh-catalyzed direct amination of unactivated C(sp3)-H bond with anthranils under mild conditions, Chem.–Eur. J., 2016, 22, 11165–11169 CrossRef PubMed;
(b) H. Lyu, Y. Quan and Z. Xie, Transition metal catalyzed direct amination of the cage B(4)-H bond in o-carboranes: synthesis of tertiary, secondary, and primary o-carboranyl amines, J. Am. Chem. Soc., 2016, 138, 12727–12730 CrossRef PubMed.
- A. Garca-Rubia, R. G. Arrays and J. C. Carretero, Palladium (II)-catalyzed regioselective direct C2 alkenylation of indoles and pyrroles assisted by the N-(2-pyridyl)
sulfonyl protecting group, Angew. Chem., Int. Ed., 2009, 48, 6511–6515 CrossRef PubMed.
-
(a) M. H. Shaw, V. W. Shurtleff, J. A. Terrett, J. D. Cuthbertson and D. W. MacMillan, Native functionality in triple catalytic cross-coupling: sp3 C-H bonds as latent nucleophiles, Science, 2016, 352, 1304–1308 CrossRef PubMed;
(b) T. Kang, Y. Kim, D. Lee, Z. Wang and S. Chang, Iridium-catalyzed intermolecular amidation of sp3 C-H bonds: late-stage functionalization of an unactivated methyl group, J. Am. Chem. Soc., 2014, 136, 4141–4144 CrossRef PubMed.
-
(a) H. T. Chang, T. T. Jayanth, C. C. Wang and C. H. Cheng, Cobalt-catalyzed reductive coupling of activated alkenes with alkynes, J. Am. Chem. Soc., 2007, 129(39), 12032–12041 CrossRef PubMed;
(b) T. Wu, X. Mu and G. Liu, Palladium-catalyzed oxidative arylalkylation of activated alkenes: dual C-H bond cleavage of an arene and acetonitrile, Angew. Chem., Int. Ed., 2011, 50, 12578–12581 CrossRef PubMed;
(c) M. B. Zhou, R. J. Song, X. H. Ouyang, Y. Liu, W. T. Wei and G. B. Deng, Metal-free oxidative tandem coupling of activated alkenes with carbonyl C(sp2)-H bonds and aryl C(sp2)-H bonds using TBHP, ChemInform, 2013, 4(6), 2690–2694 Search PubMed;
(d) D. Zhou, Z. Li, J. Li, S. Li, M. Wang, X. Luo, G. Ding, R. Sheng, M. Fu and S. Tang, Copper-catalysed alkylarylation of activated alkenes using AIBN and beyond: an access to cyano-containing oxindoles, Eur. J. Org. Chem., 2015, 1606–1612 CrossRef.
-
(a) R. S. Xu, J. P. Wan, H. Mao and Y. J. Pan, Facile synthesis of 2-(phenylthio) phenols by copper(I)-catalyzed tandem transformation of C-S coupling/C-H functionalization, J. Am. Chem. Soc., 2010, 132, 15531–15533 CrossRef PubMed;
(b) R. S. Xu, L. Yue and Y. J. Pan, Regioselective copper(I)-catalyzed C-H hydroxylation/C-S coupling: expedient construction of 2-(styrylthio) phenols, Tetrahedron, 2012, 68, 5046–5052 CrossRef.
-
(a) S. Jana, S. Dalapati, S. Ghosh and N. Guchhait, Excited state intramolecular charge transfer process in 5-(4-dimethylamino-phenyl)-penta-2,4-dienoic acid ethyl ester and effect of acceptor functional groups, J. Photochem. Photobiol., A, 2013, 261(6), 31–40 CrossRef;
(b) S. Kashino and M. Haisa, The structures of 5-phenyl-2,4-pentadienoic acid (ppa) and 1,5-diphenyl-2,4-pentadien-1-one (dpo), Acta Crystallogr., 2010, 36(2), 346–353 CrossRef;
(c) W. Bollag, M. Klaus, P. Mohr, P. Panina-Bordignon, M. Rosenberger, F. Sinigaglia, Retinoid antagonists and use thereof, CA, WO2000053562A1, 2000.
-
(a) C. U. Maheswari, G. S. Kumar and K. R. Reddy, Recent Advances in copper-catalyzed oxidative cross-coupling chemistry, Curr. Org. Chem., 2016, 20, 512–579 CrossRef;
(b) X. X. Guo, D. W. Gu, Z. Wu and W. Zhang, Copper-catalyzed C-H functionalization reactions: efficient synthesis of heterocycles, Chem. Rev., 2015, 115, 1622–1651 CrossRef PubMed.
-
(a) S. D. McCann and S. S. Stahl, Copper-catalyzed aerobic oxidations of organic molecules: pathways for two-electron oxidation with a four-electron oxidant and a one-electron redox-active catalyst, Acc. Chem. Res., 2015, 48, 1756–1766 CrossRef PubMed;
(b) S. A. Girard, T. Knauber and C.-J. Li, The cross-dehydrogenative coupling of C-H bonds: a versatile strategy for C-C bond formations, Angew. Chem., Int. Ed., 2014, 53, 74–100 CrossRef PubMed;
(c) S. E. Allen, R. R. Walvoord, R. Padilla-Salinas and M. C. Kozlowski, Aerobic copper-catalyzed organic reactions, Chem. Rev., 2013, 113, 6234–6458 CrossRef PubMed.
Footnote |
† Electronic supplementary information (ESI) available. See DOI: 10.1039/c8ra05311a |
|
This journal is © The Royal Society of Chemistry 2018 |
Click here to see how this site uses Cookies. View our privacy policy here.