DOI:
10.1039/C8RA05504A
(Paper)
RSC Adv., 2018,
8, 33845-33854
Variation in the main health-promoting compounds and antioxidant activity of whole and individual edible parts of baby mustard (Brassica juncea var. gemmifera)
Received
27th June 2018
, Accepted 21st September 2018
First published on 2nd October 2018
Abstract
Despite being a brassicaceous vegetable that is widely consumed in winter and spring in Southwest China, there is lack of information available on baby mustard. The aim of this study was to determine the contents of soluble proteins, soluble sugars, chlorophylls, carotenoids, ascorbic acid, proanthocyanidins, flavonoids, total phenolics, and glucosinolates, as well as the antioxidant activity of the whole edible parts and seven individual edible parts (swollen stem: petioles, peel, flesh; lateral bud: leaves, petioles, peel, flesh) of baby mustard. The results showed that significant differences in health-promoting compounds and antioxidant activity existed between the different edible parts. The lateral bud of baby mustard possessed greater health-promoting compounds than the swollen stem. In particular, the lateral bud leaves possessed abundant antioxidant compounds and antioxidant activity, indicating that these should be conserved during harvesting due to their potential contribution to human health. Furthermore, aliphatic glucosinolates were predominant, and sinigrin was the most abundant glucosinolate in all the assessed parts of baby mustard, the content of which was 15.81 μmol g−1 dry weight, accounting for more than 87% of the total glucosinolate content. However, the sinigrin content in baby mustard is lower than tuber mustard, which explains the less pungent flavor of baby mustard and its better suitability as a fresh vegetable. In addition, antioxidant activity was highly correlated with total phenolics, whereas gluconasturtiin and soluble sugars were negatively correlated with the majority of antioxidants.
Introduction
The concentration and composition of glucosinolates, which constitute important secondary metabolites of brassicaceous plants,1 are influenced by many environmental factors and also vary greatly between different tissues within the same plant.2,3 By mid-2014, more than 130 different glucosinolates had been identified.4 Based on the structure of different amino acid precursors, glucosinolates can be grouped into three classes, namely aliphatic, with a methionine precursor; indole, with a tryptophan precursor; and aromatic, with an aromatic amino acid precursor.5 It is widely recognized that glucosinolates and their breakdown products all exhibit strong biological activity. When cruciferous plant tissues suffer mechanical damage, glucosinolates will react with myrosinase to destabilize isothiocyanates,6 which has also been shown to be effective in reducing the risk of cancer, particularly bladder, colon, and lung cancer.7–9 In addition to glucosinolates, Brassica vegetables also contain many health-promoting compounds, including ascorbic acid, carotenoids, and various phenolics.10–13
The cruciferous vegetable baby mustard (Brassica juncea var. gemmifera) is a popular vegetable that is grown in the moist climate of Southwest China. It is a variant of stem mustard that was discovered in China in 1985 and is eaten in the winter and spring seasons when fewer fresh vegetables are available.14 It is tender with a sweet and fragrant flavor and can be eaten raw, cooked, or pickled.15 There are a few varieties of baby mustard, and ‘Linjiang-Ercai’ is the primary variety consumed in Southwest China. Vegetables are indispensable to the human diet and are valued for their nutritional properties. A number of studies are available on various Brassica vegetables; however, there have been far fewer studies on baby mustard, and even fewer regarding the nutritional composition of the individual edible parts. The objective of this study was thus to determine the content and composition of the main health-promoting compounds and the antioxidant activities in the whole and individual edible parts of baby mustard. These findings will provide a guideline for the human diet.
Experimental
Plant material and cultivation
Baby mustard plants were grown in an open field at an experimental base located at the Sichuan Agricultural University, China (29°58′54.23′′N; 102°59′57.54′′E) on September 2nd, 2016, and were harvested on December 25th, 2016. The cultivar of this experimental material is ‘Linjiang-Ercai’, which is the main cultivar in China. Five baby mustard plants were divided into one group as a repeat, and four repeats were used in this experiment. After harvesting, whole edible parts of baby mustard (Fig. 1A) exhibiting similar growth tendencies were transported to the laboratory immediately and divided into seven individual parts according to their morphological and botanical difference, including swollen stem petioles, swollen stem peel, swollen stem flesh, lateral bud leaves, lateral bud petioles, lateral bud peel, and lateral bud flesh. The whole edible parts of baby mustard also constituted a sample (Fig. 1B). The samples were then lyophilized in a freeze-dryer and stored at −20 °C until further analysis.
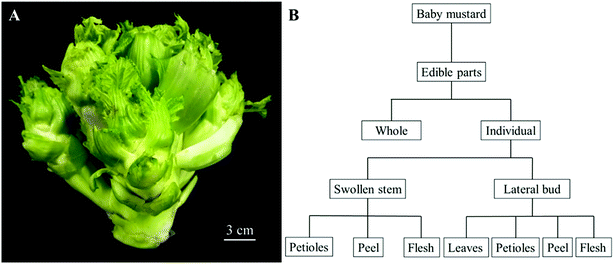 |
| Fig. 1 The edible parts of baby mustard. (A) The whole edible parts; (B) the division of the edible parts. | |
Quality assessment
Glucosinolate composition and content. Glucosinolates were extracted and analyzed as previously described.16,17 Freeze-dried samples (100 mg) were boiled in 5 mL water for 10 min. The supernatant was collected after centrifugation (5 min, 4000g), and the residues were washed once with water (5 mL), centrifuged and then combined with the previous extract. The aqueous extract was applied to a DEAE-Sephadex A-25 (40 mg) column (pyridine acetate form) (GE Healthcare, Piscataway, NJ). The glucosinolates were converted into their desulpho analogues by overnight treatment with 100 μL of 0.1% (1.4 units) aryl sulphatase (Sigma), and the desulphoglucosinolates were eluted with 2 × 0.5 mL water. HPLC analysis of desulphoglucosinolates was carried out using a Waters High-performance Liquid Chromatography (HPLC) instrument equipped with a Model 2996 PDA absorbance detector (Waters, USA). Samples (20 μL) were separated at 30 °C on a Waters Spherisorb C18 column (250 × 4.6 mm i.d.; 5 μm particle size) using acetonitrile and water at a flow rate of 1.0 mL min−1. Absorbance was detected at 226 nm. ortho-Nitrophenyl-β-D-galactopyranoside (Sigma) was used as an internal standard for HPLC analysis.
Soluble protein content. The soluble protein content was determined using the method of Bradford.18 Fifty milligrams of freeze-dried powdered material was soaked in 10 mL of distilled water. The solution was stirred for 30 s using a vortex mixer, after which it was allowed to settle for 30 min. The solution was then centrifuged for 5 min at 4000g and 1 mL transferred to a polypropylene tube. Subsequently, Coomassie brilliant blue G-250 was combined with 1 mL of supernatant. The absorbance was measured at 595 nm within 20 min after the reaction. Soluble proteins in the samples were calculated based on a standard curve of bovine serum albumin.
Soluble sugar content. The determination of soluble sugar content was performed using the method proposed by Morris.19 Fifty milligrams of powder was extracted in 10 mL of distilled water for 20 min at 90 °C, following which the homogenates were centrifuged at 4000g for 5 min. A combination of 1 mL of sample extract, 0.5 mL anthrone-ethyl acetate reagent, and 5 mL concentrated sulfuric acid was homogenized and boiled for 5 min, and then cooled rapidly using ice water. The absorbance of the reaction mixtures was measured at 630 nm using a spectrophotometer, and the soluble sugar content was determined using a standard curve of sucrose.
Chlorophyll content. Fifty mg powder of lateral bud leaves and 300 mg of other samples were ground and extracted with 10 mL ethanol, centrifuged at 4000g at room temperature for 5 min, respectively. The supernatant was collected and total chlorophyll content was measured by reading the absorbance at 665 nm and 649 nm with a spectrophotometer. Chlorophyll contents were expressed as mg kg−1 dry weight.13
Total carotenoid content. Fifty mg of powder was extracted with 10 mL of a mixture of acetone and petroleum ether (1
:
1, v/v), total carotenoid content was measured by reading the absorbance at 451 nm with spectrophotometer. Total carotenoid contents were expressed as mg kg−1 dry weight.13
Ascorbic acid content. Ascorbic acid content was determined using the methods of Sun et al.13 Fifty mg of sample powder was extracted with 5 mL 1.0% (w/v) oxalic acid, subsequently centrifuged 5 min at 4000g. Each sample was filtered through a 0.45 μm cellulose acetate filter. HPLC analysis of ascorbic acid was carried out using a Waters instrument with a Model 2996 PDA detector (Waters Inc., Milford, USA). Sample (20 μL) were separated at room temperature on a Waters Spherisorb C18 column (150 × 4.6 mm id; 5 μm particle size), using a solvent of 0.1% oxalic acid at a flow rate of 1.0 mL min−1. The amount of ascorbic acid was calculated from absorbance values at 243 nm, using authentic ascorbic acid as a standard. The results were expressed as mg g−1 dry weight.
Proanthocyanidin content. Proanthocyanidin content was determined according to the method described by Prior et al.20 Forty milligrams of the lyophilized powder was transferred to 4 mL of the extracting reagent (acetone
:
distilled water
:
acetic acid = 150
:
49
:
1, v/v). The solution was vigorously vortexed for 5 min, shaken for 1 h, and then centrifuged at 4000g for 5 min. Subsequently, 2.1 mL p-dimethylaminocinnamaldehyde (DMACA) reagent was added to 700 μL of supernatant. The absorbance of the mixture was spectrophotometrically detected at 640 nm after 20 min, and the proanthocyanidin content was determined using a standard curve of procyanidin B2.
Flavonoid content. Forty milligrams of sample powder was extracted in 50% ethanol and incubated at room temperature for 24 h in the dark. The suspension was then centrifuged at 4000g for 5 min at room temperature. A 1.2 mL aliquot of the supernatant was mixed with 60 μL 2% aluminum trichloride, the same volume (60 μL) of 1 mol L−1 potassium acetate, and 1.680 mL distilled water. Absorption was read at 415 nm after 40 min. The flavonoid content was determined using a standard calibration curve with quercetin in 50% ethanol as a reference standard and expressed as mg of quercetin equivalence per g dry weight.21
Total phenolic content. Total phenolics were extracted with 50% ethanol and incubated at room temperature for 24 h in the dark. The suspension was centrifuged at 4000g for 5 min at room temperature. The supernatant (300 μL) was mixed with 1.5 mL 0.2 mol L−1 Folin–Ciocalteu reagent in a polypropylene tube, after 3 min, 1.2 mL saturated sodium carbonate was added to each polypropylene tube. The mixtures were allowed to stand for 20 min at room temperature and the absorbance was measured at 760 nm with the spectrophotometer as previously described.22 Gallic acid was used as a standard and the results were expressed as mg garlic acid equivalent (GAE) per g dry weight.
Ferric reducing antioxidant power (FRAP). FRAP assay was performed according to the method of Benzie and Strain.23 The working FRAP reagent was prepared daily by mixing 300 mmol L−1 acetate buffer (pH 3.6), 20 mmol L−1 ferric chloride, and 10 mmol L−1 2,4,6-tripyridyl-S-triazine in 40 mmol L−1 HCl in the ratio of 10
:
1
:
1 (v/v/v). The extracted samples (300 μL) were added to 2.7 mL of the FRAP working solution incubated at 37 °C and vortexed. The absorbance was then recorded at 593 nm using a UV-Vis spectrophotometer after the mixture had been incubated in at 37 °C for 10 min. FRAP values were calculated based on FeSO4·7H2O standard curves and expressed as mmol g−1 dry weight.
2,2-Azinobis(3-ethyl-benzothiazoline-6-sulfonic acid) (ABTS). ABTS antioxidant activity was performed according to the method of Subhasree et al.24 The radicals ABTS+ were generated by the addition of 2.45 mmol L−1 ammonium persulfate to a 7 mM ABTS solution, and the ABTS+ solution was stored in darkness for 16 h. The ABTS+ solution was adjusted with acetate buffer (pH 4.5) to an absorbance of 0.700 (± 0.020) at 734 nm. An aliquot of 300 μL of each extracted sample was added to 3 mL of ABTS+ solution. The absorbance was measured spectrophotometrically at 734 nm after exactly 2 h. The percentage inhibition was calculated according to the formula: % inhibition = [(Acontrol − Asample)/Acontrol] × 100%.
Statistical analysis
All assays were performed in quadruplicate. The results are shown as the mean ± standard deviation (SD). Statistical analysis was performed using the SPSS package program version 18 (SPSS Inc., Chicago, IL, USA). Data were analyzed using one-way analysis of variance (ANOVA). The means were compared using the Least Significant Differences (LSD) test at a significance level of 0.05. Hierarchical clustering analysis (HCA) and correlation analysis by means of a heatmap were generated using TIGR MeV software (Version 4.1). Principal component analysis (PCA) and partial least squares-discriminant analysis (PLS-DA) were performed in SIMCA-P 11.5 Demo software (Umetrics, Sweden) with unit variance (UV)-scaling to decipher the relationships among samples.25
Results
Glucosinolates
Unsurprisingly, all the individual baby mustard parts contained glucosinolates. Four indole (4-hydroxy glucobrassicin, glucobrassicin, 4-methoxyglucobrassicin, and neoglucobrassicin), three aliphatic (sinigrin, glucoalyssin, and gluconapin), and one aromatic (gluconasturtiin) glucosinolates were detected by High-Performance Liquid Chromatography (HPLC). There were remarkable differences in the glucosinolate composition and content among the individual edible parts of baby mustard. The total glucosinolate content of the edible parts ranged from 6.09 μmol g−1 in the swollen stem petioles to 33.60 μmol g−1 in the lateral bud peel. The total glucosinolate content of whole baby mustard was 18.11 μmol g−1. Aliphatic glucosinolates were the main contributor, contributing 90.78% of the glucosinolates in the whole edible parts, followed by indole glucosinolates (7.62%) and aromatic glucosinolates (1.55%). Among all of the glucosinolates, sinigrin was the major glucosinolate and accounted for 87.30% of the whole edible parts. A similar distribution was observed in the individual edible parts. In addition, among the edible parts, eight glucosinolates were detected in the lateral bud peel and lateral bud flesh; seven glucosinolates were detected in the swollen stem petioles, swollen stem peel, and lateral bud petioles; and five glucosinolates were observed in the lateral bud leaves. In contrast, only three glucosinolates were identified in the swollen stem flesh, including one indole, one aliphatic, and one aromatic glucosinolate. It should be noted that glucoalyssin was identified in all of the four parts of the lateral bud, but were not detected in the swollen stem. Excepting the lateral bud leaves, the distribution of glucosinolates in the swollen stem and lateral bud was concordant. The peel had the highest glucosinolate content, followed by the flesh and petioles whether in the swollen stem or lateral bud. Furthermore, for the same tissue, the contents of total glucosinolates in the lateral bud were significantly higher than in the swollen stem (Table 1).
Table 1 The composition and content of glucosinolates in whole and seven individual edible parts of baby mustard (μmol g−1 DW)a
Gulcosinolates |
Whole |
Swollen stem |
Lateral bud |
Petioles |
Peel |
Flesh |
Leaves |
Petioles |
Peel |
Flesh |
Data are expressed as mean ± standard deviation. Same letter in the same row means no significant differences among values (p < 0.05) according to the LSD's test. |
Aliphatic |
Sinigrin |
15.81 ± 1.86 c |
4.88 ± 0.47 f |
11.44 ± 2.74 d |
5.42 ± 0.57 f |
10.01 ± 0.84 de |
8.70 ± 1.30 e |
29.64 ± 1.31 a |
21.54 ± 0.41 b |
Glucoalyssin |
0.15 ± 0.03 c |
n.d. |
n.d. |
n.d. |
0.99 ± 0.12 a |
0.35 ± 0.11 b |
0.05 ± 0.07 c |
0.09 ± 0.06 c |
Gluconapin |
0.49 ± 0.04 c |
0.16 ± 0.01 d |
0.50 ± 0.13 c |
n.d. |
0.26 ± 0.10 d |
0.66 ± 0.22 b |
1.02 ± 0.05 a |
0.70 ± 0.01 b |
Total aliphatic |
16.44 ± 1.91 c |
5.05 ± 0.48 f |
11.94 ± 2.87 d |
5.42 ± 0.57 f |
11.27 ± 0.87 de |
9.71 ± 1.36 e |
30.71 ± 1.43 a |
22.33 ± 0.36 b |
![[thin space (1/6-em)]](https://www.rsc.org/images/entities/char_2009.gif) |
Indole |
4-Hydroxy glucobrassicin |
0.29 ± 0.06 b |
0.10 ± 0.02 c |
0.15 ± 0.10 bc |
n.d. |
n.d. |
n.d. |
0.60 ± 0.10 a |
0.56 ± 0.19 a |
Glucobrassicin |
0.51 ± 0.08 b |
0.22 ± 0.02 c |
0.45 ± 0.08 b |
n.d. |
0.55 ± 0.13 ab |
0.45 ± 0.29 b |
0.72 ± 0.03 a |
0.03 ± 0.02 d |
4-Methoxy glucobrassicin |
0.34 ± 0.06 d |
0.31 ± 0.03 d |
0.22 ± 0.03 d |
0.21 ± 0.02 d |
2.20 ± 0.34 a |
1.66 ± 0.11 b |
0.66 ± 0.05 c |
0.35 ± 0.01 d |
Neoglucobrassicin |
0.24 ± 0.04 a |
0.18 ± 0.01 b |
0.05 ± 0.01 d |
n.d. |
n.d. |
0.09 ± 0.02 c |
0.21 ± 0.02 b |
0.11 ± 0.00 c |
Total indole |
1.38 ± 0.21 c |
0.81 ± 0.06 d |
0.86 ± 0.23 d |
0.21 ± 0.02 e |
2.75 ± 0.28 a |
2.19 ± 0.41 b |
2.19 ± 0.19 b |
1.04 ± 0.21 d |
![[thin space (1/6-em)]](https://www.rsc.org/images/entities/char_2009.gif) |
Aromatic |
Gluconasturtiin |
0.28 ± 0.06 de |
0.22 ± 0.03 e |
0.90 ± 0.21 a |
0.55 ± 0.03 c |
n.d. |
0.34 ± 0.14 de |
0.71 ± 0.06 b |
0.41 ± 0.01 cd |
Total aromatic |
0.28 ± 0.06 de |
0.22 ± 0.03 e |
0.90 ± 0.21 a |
0.55 ± 0.03 c |
n.d. |
0.34 ± 0.14 de |
0.71 ± 0.06 b |
0.41 ± 0.01 cd |
Total glucosinolates |
18.11 ± 2.18 c |
6.09 ± 0.58 e |
13.71 ± 3.30 d |
6.18 ± 0.59 e |
14.02 ± 0.82 d |
12.24 ± 1.61 d |
33.60 ± 1.61 a |
23.78 ± 0.54 b |
Soluble proteins and soluble sugars
The composition and content of the main nutrients and health-promoting compounds in baby mustard are shown in Table 2. The soluble protein content in the seven individual edible parts ranged from 38.81 mg g−1 to 91.10 mg g−1. Overall, the soluble protein content was highest in the lateral bud leaves, followed by the lateral bud petioles, and was lowest in the swollen stem petioles. The soluble proteins in the flesh were highest, followed by the peel and then the petioles in the swollen stem, while an opposite pattern was observed in the lateral bud. A comparison of the seven edible parts with the entire baby mustard plant indicated that the lateral bud leaves, petioles, and peel were higher in soluble protein than the whole plant, while the other parts were lower in soluble protein content.
Table 2 The composition and content of main health-promoting compounds in whole and seven individual edible parts of baby mustarda
Index |
Whole |
Swollen stem |
Lateral bud |
Petioles |
Peel |
Flesh |
Leaves |
Petioles |
Peel |
Flesh |
Data are expressed as mean ± standard deviation. Same letter in the same row means no significant differences among values (p < 0.05) according to the LSD's test. |
Soluble proteins (mg g−1) |
75.95 ± 2.76 c |
38.81 ± 1.86 f |
59.04 ± 0.99 e |
60.18 ± 1.01 de |
91.10 ± 1.13 a |
80.97 ± 4.50 b |
79.08 ± 2.23 bc |
63.11 ± 1.12 d |
Soluble sugars (mg g−1) |
424.06 ± 11.51 ab |
378.25 ± 30.69 bc |
273.79 ± 17.35 d |
337.96 ± 4.38 cd |
155.31 ± 3.03 e |
336.38 ± 29.32 cd |
341.67 ± 59.89 cd |
463.85 ± 35.69 a |
Chlorophylls (mg kg−1) |
161.89 ± 3.15 d |
313.58 ± 8.83 c |
94.46 ± 6.72 f |
10.71 ± 2.24 h |
3345.13 ± 22.56 a |
689.64 ± 8.47 b |
134.11 ± 7.99 e |
47.42 ± 4.43 g |
Carotenoids (mg kg−1) |
22.50 ± 1.13 c |
16.95 ± 1.64 cd |
9.64 ± 1.17 cde |
0.88 ± 0.22 e |
335.02 ± 15.88 a |
69.48 ± 1.63 b |
19.22 ± 1.02 cd |
6.14 ± 0.61 de |
Ascorbic acid (mg g−1) |
2.69 ± 0.33 d |
2.93 ± 0.13 cd |
2.59 ± 0.21 d |
4.58 ± 0.28 a |
4.63 ± 0.42 a |
5.11 ± 0.33 a |
3.45 ± 0.29 bc |
3.84 ± 0.4 b |
Proanthocyanidins (mg g−1) |
1.08 ± 0.10 b |
0.64 ± 0.05 c |
0.55 ± 0.06 cd |
0.20 ± 0.02 e |
3.35 ± 0.06 a |
1.14 ± 0.02 b |
1.18 ± 0.03 b |
0.42 ± 0.05 d |
Flavonoids (mg g−1) |
4.02 ± 0.16 e |
3.58 ± 0.39 e |
1.84 ± 0.22 f |
12.06 ± 0.23 b |
19.30 ± 0.55 a |
4.91 ± 0.28 d |
2.07 ± 0.27 f |
8.68 ± 0.09 c |
Total phenolics (mg g−1) |
3.26 ± 0.25 d |
2.84 ± 0.27 de |
1.96 ± 0.10 f |
4.01 ± 0.19 c |
17.66 ± 0.35 a |
5.50 ± 0.29 b |
2.53 ± 0.11 e |
2.96 ± 0.26 de |
The soluble sugar contents ranged between 155.31 mg g−1 in the lateral bud leaves and 463.85 mg g−1 in the lateral bud flesh. The difference among the samples was nearly three-fold. The soluble sugar content of the petioles was highest in the swollen stem, whereas the flesh in the lateral bud had the highest soluble sugar content. Of the edible parts, only the lateral bud flesh was higher in comparison to the whole parts (Table 2).
Chlorophylls and carotenoids
Chlorophyll content ranged from 10.71 mg kg−1 in the swollen stem flesh to 3345.13 mg kg−1 in the lateral bud leaves. A similar distribution was observed with the carotenoid content in the various edible parts. The carotenoid content ranged from 0.88 mg kg−1 in the swollen stem flesh to 335.02 mg kg−1 in the lateral bud leaves. Thus, the chlorophyll and carotenoid contents in the lateral bud leaves were generally more than 300 times greater than those in the swollen stem flesh (Table 2).
Ascorbic acid
The ascorbic acid content was lowest in the swollen stem peel (2.59 mg g−1) and highest in the lateral bud petioles (5.11 mg g−1). In the swollen stem, the ascorbic acid content in the flesh was highest, followed by the petioles and the peel. In the lateral bud, the petioles had the highest content of ascorbic acid, followed by the leaves, flesh, and peel (Table 2).
Proanthocyanidins, flavonoids, and total phenolics
There were significant differences in the contents of proanthocyanidins, flavonoids, and total phenolics among the different edible parts. The highest content of proanthocyanidins, flavonoids, and total phenolics were observed in the lateral bud leaves (3.35 mg g−1, 19.30 mg g−1, and 17.66 mg g−1, respectively). The lowest proanthocyanidin content was recorded in the swollen stem flesh (0.20 mg g−1). However, the lowest contents of flavonoids and total phenolics were both detected in the swollen stem peel (1.84 mg g−1 and 1.96 mg g−1, respectively). With the exception of the lateral bud leaves, the distribution of flavonoids in the swollen stem and lateral bud was concordant. The flesh contained the highest flavonoid content, followed by the petioles and peel whether in the swollen stem or lateral bud. Furthermore, the lowest proanthocyanidin content was detected in the flesh, regardless of whether it was the swollen stem or lateral bud. However, no significant difference in proanthocyanidin content was found between the petioles and peel. Nevertheless, the distribution of total phenolics in the swollen stem and lateral bud among the petioles, peel, and flesh differed. The highest total phenolic content in the swollen stem was detected in the flesh, followed by the petioles and the peel. In contrast, in the lateral bud, the phenolic content in the petioles was higher than in the flesh, while the peel still contained the lowest content of total phenolics (Table 2).
Antioxidant activity
The results obtained for the antioxidant activity based on the FRAP and ABTS assays are displayed in Fig. 2. Significant differences were observed in the individual edible parts. The highest FRAP and ABTS values (121.17 mmol kg−1 and 69.59%, respectively) were measured in the lateral bud leaves. The swollen stem peel was characterized by the lowest FRAP and ABTS activities (33.92 mmol kg−1 and 19.90%, respectively). The antioxidant activity of the flesh was significantly higher than the peel in both the swollen stem and lateral bud. Additionally, the antioxidant activities of the swollen stem petioles, peel, and lateral bud peel were significantly lower than the whole edible parts, while those of the remaining parts were higher.
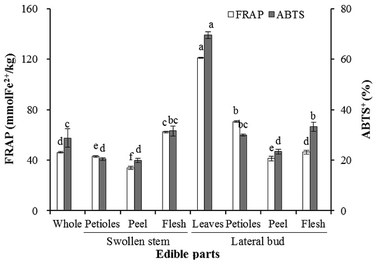 |
| Fig. 2 Antioxidant activities in the whole and individual edible parts of baby mustard. Standard deviations are expressed by bars. For each tissue, same letter in the same testing method means no significant differences (p < 0.05) according to the LSD's test. | |
HCA
HCA of the whole and individual edible parts was performed to visualize the main health-promoting compounds and antioxidant activities (Fig. 3). To determine the relationships and trends of these different parts, the heatmap was divided into four groups. Group A only contained ascorbic acid and flavonoids, which were highest in the lateral bud leaves and relatively high in the flesh of the swollen stem and the lateral bud. Group B is the largest group in Fig. 3 and contained antioxidant activities and most of the health-promoting compounds. The lateral bud leaves were highest in these indices. Group C included gluconasturtiin, a type of aromatic glucosinolate, and total aromatic glucosinolates, which were highest in the peel whether in the swollen stem or lateral bud. Sinigrin, the principal contributor of total aliphatic glucosinolates and total glucosinolates, together with soluble sugars and several other glucosinolates, was located in group D. These were most highly expressed in the peel and flesh of the lateral bud.
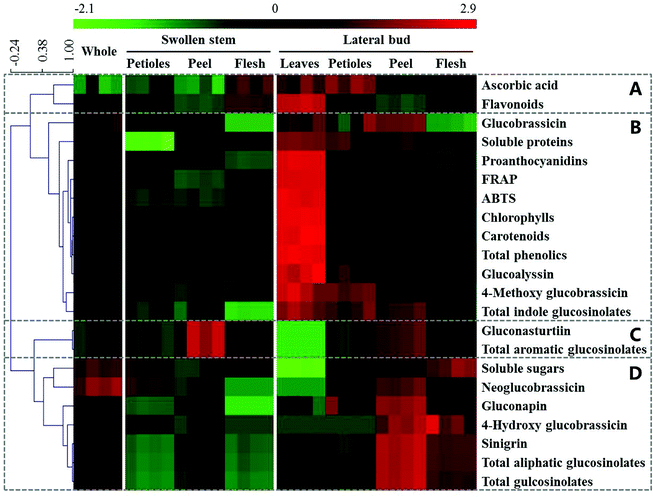 |
| Fig. 3 Heatmap and HCA of the main health-promoting compounds and antioxidant activities in whole and individual parts of baby mustard. Green and red reflect the relative concentrations. | |
PCA
PCA was performed to compare the distribution of the main health-promoting compounds and antioxidant activity among the whole and individual parts of baby mustard. As shown in Fig. 4, the first component accounted for 52%, while the second component represented 26%. The whole edible part samples grouped close to the origin. The leaves and petioles of the lateral bud could be discriminated from the other parts by PC1. Additionally, the swollen stem could be discriminated from the lateral bud by PC2 (Fig. 4A). The results of the PCA and PLS-DA were congruent and indicated that the whole edible parts were similar to the lateral bud flesh in terms of the measured constituents, which is to be expected as the lateral bud flesh is the primary edible part in baby mustard (Fig. 4B). Fig. 4C shows that PC1 was dominated by total phenolics, FRAP, chlorophylls, carotenoids, glucoalyssin, ABTS, proanthocyanidins, 4-methoxy glucobrassicin, flavonoids, soluble sugars, gluconasturtiin, and total aromatic glucosinolates. In contrast, PC2 was dominated by total glucosinolates, total aliphatic glucosinolates, sinigrin, gluconapin, and flavonoids. The lateral bud leaves and other parts were primarily discriminated based on chlorophylls, carotenoids, total phenolics, FRAP, ABTS, glucoalyssin, 4-methoxy glucobrassicin, and proanthocyanidins. In contrast, gluconapin, sinigrin, total aliphatic glucosinolates, and total glucosinolates play an important role in the lateral peel.
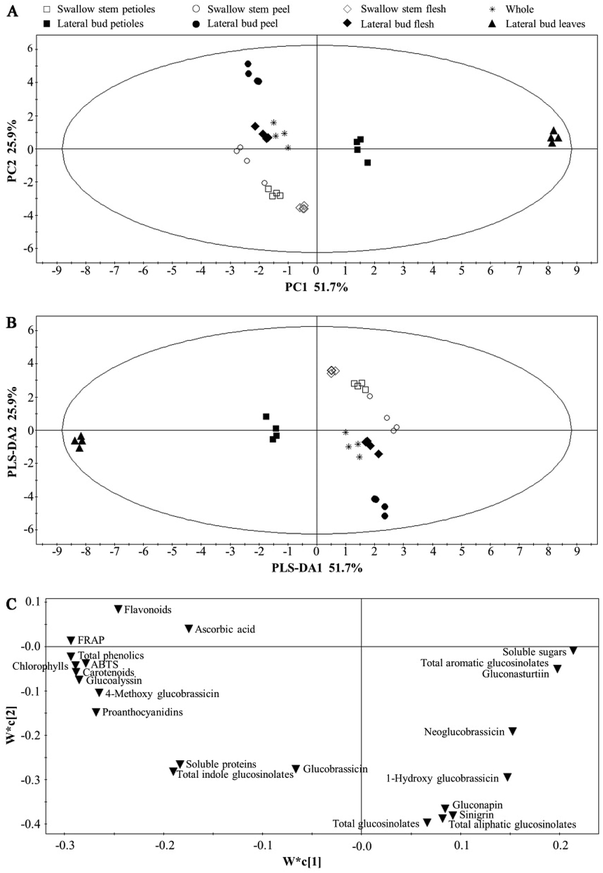 |
| Fig. 4 PCA and PLS-DA analysis of whole and individual parts of baby mustard. (A) PCA score plot; (B) PLS-DA score plot; (C) PLS-DA loading plot. | |
Correlation analysis
The correlation between the health-promoting compounds and antioxidant activity was expressed as a heatmap (Fig. 5). Correlation analysis indicated two significantly positively correlated connections between the variables. The first was the correlation between glucobrassicin, ascorbic acid, flavonoids, FRAP, ABTS, chlorophylls, carotenoids, total phenolics, glucoalyssin, proanthocyanidins, 4-methoxy glucobrassicin, soluble proteins, and total indole glucosinolates, and the second was the association between gluconasturtiin, total aromatic glucosinolates, soluble sugars, neoglucobrassicin, 4-hydroxy glucobrassicin, gluconapin, sinigrin, total aliphatic glucosinolates, and total glucosinolates, with the exception that gluconasturtiin and total aromatic glucosinolates were not correlated with soluble sugars and neoglucobrassicin. However, most of the variables between the first group and second group were negatively correlated with each other. In addition, the positive correlations between chlorophylls and carotenoids, as well as among sinigrin, total aliphatic glucosinolates, and total glucosinolates, were highest, while the greatest negative correlations were observed between gluconasturtiin, total aromatic glucosinolates, soluble sugars and flavonoids, FRAP, ABTS, chlorophylls, carotenoids, total phenolics, glucoalyssin, proanthocyanidins, and 4-methoxy glucobrassicin.
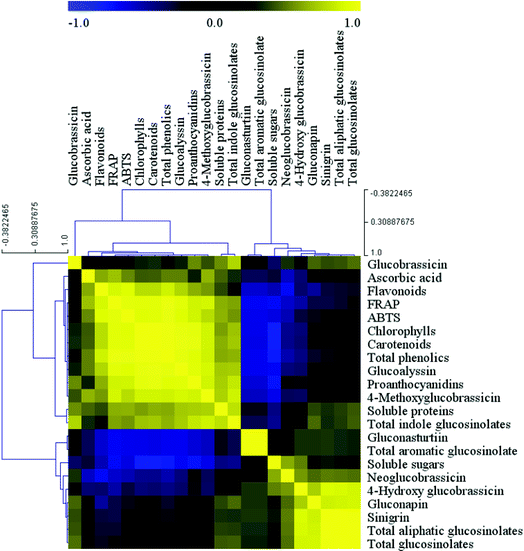 |
| Fig. 5 Correlation analysis among the main health-promoting compounds and antioxidant activity in baby mustard. Yellow indicates a positive correlation, black intermediate no relevance, and blue indicates a negative correlation. | |
The ABTS and FRAP results were consistent with each other. The correlation analysis indicated that antioxidant activity was positively associated with ascorbic acid, chlorophylls, carotenoids, total phenolics, flavonoids, glucoalyssin, proanthocyanidins, 4-methoxy glucobrassicin, soluble proteins, and total indole glucosinolates. Specifically, the correlation with total phenolics was highest, whereas the remaining components were negatively correlated, with the exception of glucobrassicin. Glucosinolates could be divided into three parts based on the correlation analysis. The first part contained glucoalyssin, which was positively correlated with 4-methoxy glucobrassicin, both of which were negatively correlated with gluconasturtiin, total aromatic glucosinolates, neoglucobrassicin, and 4-hydroxy glucobrassicin. The second part contained gluconasturtiin, which was highly positively correlated with total aromatic glucosinolates. The third part contained neoglucobrassicin, 4-hydroxy glucobrassicin, sinigrin, total aliphatic glucosinolates, and total glucosinolates, all of which were positively correlated with one another.
Discussion
The present study is the first to comprehensively assess the primary health-promoting compounds, identify the glucosinolate compositions, and evaluate the antioxidant activities of the whole and individual edible parts of baby mustard. Our results showed that significant differences existed among the different individual edible parts, and the nutritional value of the lateral bud was higher than the swollen stem. Eight glucosinolates were detected, and sinigrin was found to be the major glucosinolate.
Many studies have indicated that significant differences exist among different plant organs/tissues in terms of nutritional composition and content.26,27 Generally, the leaves are more nutritious than the petioles, roots, and other organs.28 Our results are consistent with this, except with regards to the ascorbic acid and soluble sugar contents. In our study, the content of ascorbic acid in the flesh was higher than the peel, which corroborates a previous study.25 Interestingly, the soluble protein, chlorophyll, carotenoid, proanthocyanidin, and majority of glucosinolate contents in the lateral bud were higher than in the swollen stem in the corresponding tissues, which might be explained by the source-sink theory.
Glucosinolates and their breakdown products are beneficial to human health. Several studies have suggested that aliphatic glucosinolates constitute a predominant component of total glucosinolates in Brassica.29–31 In this study, aliphatic glucosinolates accounted for almost 90% of the whole edible parts of baby mustard. Additionally, the glucosinolate composition and content in the different edible parts differed significantly in our study, which corroborates the results of Maldini et al.32 and Sun et al.16 Furthermore, the content of aromatic glucosinolates in the peel was higher than the other edible parts of baby mustard. Considering that the peel constitutes the protective screen of plants, we inferred that the high content of aromatic glucosinolates in the peel protects the plants by resisting disease and pathogens.33
Many studies have confirmed that glucosinolate compositions differ among distinct species. The most abundant glucosinolate in mustard is sinigrin,34 while in broccoli it is glucoraphanin;17 in Chinese kale it is gluconapin;16 in loose-curd cauliflower it is glucoiberin;35 in cabbage it is glucobrassicin; and in the different tissues of wild radish it is glucoraphanin and glucoraphasatin.32 However, glucosinolate compositions are typically similar in the same species. Seven types of glucosinolates have previously been detected in Indian mustard,36 nine have been detected in tuber mustard, and eight were detected in baby mustard in the present study. In our study, the content of sinigrin in the various tissues of the edible parts of baby mustard accounted for about 70% to 90% of the total glucosinolate composition. Sinigrin is also the dominant glucosinolate in tuber mustard. However, there was a slight difference in aliphatic glucosinolate composition between baby mustard and tuber mustard, whereas the composition of other glucosinolates was the same.37 Sinigrin was found to be one of the most important glucosinolates influencing the flavor of cruciferous plants, and is an important component of pungency in mustard. However, the sinigrin content in baby mustard (15.81 μmol g−1 DW) was significantly lower than in tuber mustard (29.09 μmol g−1 DW), which is reflected by their different uses in the everyday human diet. Tuber mustard is typically made into preserved Szechuan pickle by the people of Southwest China, the unique flavor of which is mainly attributed to the abundant glucosinolate hydrolysates. Baby mustard has been artificially bred for its low glucosinolate content, and particularly its sinigrin content, which renders the flavor of baby mustard less pungent and more suitable for being eaten fresh.
Antioxidant activity between different plant organs/tissues generally differs significantly.38,39 Our results also indicated that the leaves, petioles, peel, and flesh of the edible parts of baby mustard exhibited good and distinct antioxidant activity as determined by ABTS and FRAP, and the tissue with the highest antioxidant activity was the leaves, while the swollen stem peel had the lowest activity. The antioxidant activity of the peel in tuber mustard and bamboo shoots mustard is higher than the flesh,25 and similar results have been found in apple.40 However, in our study, the antioxidant activity of the peel and flesh in baby mustard exhibited a contrary pattern. Antioxidant activity is correlated with total phenolic content in many studies,41 and the total phenolic content in the flesh was also higher than in the peel in baby mustard.
Gluconasturtiin, soluble sugars, and many antioxidants were negatively correlated, whereas gluconasturtiin and soluble sugars did not exhibit any correlation. Interestingly, glucosinolates, soluble sugars, and antioxidant activity are all closely associated with stress resistance in plants.33,42,43 Usually, the soluble sugar content will increase and plant growth will decline when plants are subjected to adverse situations. We inferred that soluble sugars, as the primary energy material, respond to stress by increasing the osmotic pressure in plants, thus resulting in growth suppression. As the situation becomes more serious, the soluble sugar content will increase accordingly. However, the high level of antioxidant activity will be beneficial for reducing injury in plants, and thus the soluble sugar content will decrease. Glucosinolate metabolites are one of the most predominant defense and immunity components in plants. It has been reported that glucosinolates will interact with each other to maintain cellular homeostasis in plants.44 In our study, glucoalyssin and 4-methoxy glucobrassicin demonstrated a synergistic interaction, and both were negatively correlated with gluconasturtiin.
Conclusions
This is the first report on the content and composition of health-promoting compounds, glucosinolates, and antioxidant activity in the whole and individual edible parts of baby mustard. The contents of these significantly differed among the whole and individual parts. Glucosinolate content was highest in the lateral bud peel in comparison to the other parts. The lateral bud had higher nutritional value than the swollen stem. In addition, the lateral bud leaves contained high levels of antioxidants and antioxidant activity, and thus should be conserved during harvesting for their health-promoting properties. Besides tissues, varieties and developmental stages also have a significant influence on health-promoting compounds, which will be explored in further study. In summary, the information in this study provides a theoretical reference for human dietary nutrition and a foundation for the further study of baby mustard.
Conflicts of interest
The authors declare no conflict of interest.
Acknowledgements
This work was supported by National Natural Science Foundation of China (31500247), Key Project of Department of Education of Sichuan Province (14ZA0016), and National Student Innovation Training Program (201710626030). We thank Accdon for its linguistic assistance during the preparation of this manuscript.
References
- E. Ciska, N. Drabińska, A. Narwojsz and J. Honke, Food Chem., 2016, 203, 340–347 CrossRef CAS PubMed.
- B. L. Petersen, S. Chen, C. H. Hansen, C. E. Olsen and B. A. Halkier, Planta, 2002, 214, 562–571 CrossRef CAS PubMed.
- R. Verkerk, M. Schreiner, A. Krumbein, E. Ciska, B. Holst, I. Rowland, R. De Schrijver, M. Hansen, C. Gerhäuser, R. Mithen and M. Dekker, Mol. Nutr. Food Res., 2009, 53, S219 CrossRef PubMed.
- N. Agerbirk and C. E. Olsen, Phytochemistry, 2015, 115, 143–151 CrossRef CAS PubMed.
- R. Agneta, F. Lelario, S. D. Maria, C. Möllers, S. A. Bufo and A. R. Rivelli, Phytochemistry, 2014, 106, 178–187 CrossRef CAS PubMed.
- G. Yuan, B. Sun, J. Yuan and Q. Wang, Food Chem., 2010, 118, 774–781 CrossRef CAS.
- G. Padilla, M. E. Cartea, P. Velasco, A. Haro and A. Ordás, Phytochemistry, 2007, 68, 536–545 CrossRef CAS PubMed.
- I. E. Sønderby, F. Geu-Flores and B. A. Halkier, Trends Plant Sci., 2010, 15, 283–290 CrossRef PubMed.
- A. T. Dinkova-Kostova and R. V. Kostov, Trends Plant Sci., 2012, 18, 337–347 CAS.
- X. Jin, T. Oliviero, R. G. M. van der Sman, R. Verkerk, M. Dekker and A. J. B. van Boxtel, LWT--Food Sci. Technol., 2014, 59, 189–195 CrossRef CAS.
- S. Park, M. V. Arasu, M. K. Lee, J. H. Chun, J. M. Seo, S. W. Lee, N. A. Al-Dhabi and S. J. Kim, Food Chem., 2014, 145, 77–85 CrossRef CAS PubMed.
- A. P. Vale, J. Santos, N. V. Brito, V. Peixoto, R. Carvalho, E. Rosa and M. B. P. P. Olierira, Food Chem., 2015, 178, 292–300 CrossRef CAS PubMed.
- B. Sun, H. Yan, F. Zhang and Q. Wang, Food Res. Int., 2012, 48, 359–366 CrossRef CAS.
- Y. Lin and S. X. Li, Acta Hortic. Sin., 1985, 12, 41–44 Search PubMed.
- W. M. Jin, S. Y. Zou, W. S. Peng and S. Z. Mai, J. Shanghai Agric. Coll., 1999, 17, 123–126 Search PubMed.
- B. Sun, N. Liu, Y. Zhao, H. Yan and Q. Wang, Food Chem., 2011, 124, 941–947 CrossRef CAS.
- J. Wang, H. Gu, H. Yu, Z. Zhao, X. Sheng and X. Zhang, Food Chem., 2012, 133, 735–741 CrossRef CAS.
- M. M. Bradford, Anal. Biochem., 1976, 72, 248–254 CrossRef CAS PubMed.
- D. L. Morris, Science, 1948, 107, 254–255 CrossRef CAS PubMed.
- R. L. Prior, E. Fan, H. Ji, A. Howell, C. Nio, M. J. Payne and J. Reed, J. Sci. Food Agric., 2010, 90, 1473–1478 CrossRef CAS PubMed.
- C. C. Chang, M. H. Yang, H. M. Wen and J. C. Chern, J. Food Drug Anal., 2002, 10, 178–182 CAS.
- E. A. Ainsworth and K. M. Gillespie, Nat. Protoc., 2007, 2, 875–877 CrossRef CAS PubMed.
- I. F. Benzie and J. J. Strain, Anal. Biochem., 1996, 239, 70–76 CrossRef CAS PubMed.
- B. Subhasree, R. Baskar, R. L. Keerthana, R. L. Susan and P. Rajasekaran, Food Chem., 2009, 115, 1213–1220 CrossRef CAS.
- B. Sun, X. Xia, J. Gu, Y. Tian, F. Zhang, Q. Chen, Y. Hou, J. Ma and H. Tang, Acta Agric. Nucl. Sin., 2016, 30, 485–492 Search PubMed.
- S. C. Liu, J. T. Lin, C. C. Hu, B. Y. Shen, T. Y. Chen, Y. L. Chang, C. H. Shih and D. J. Yang, Food Chem., 2017, 215, 284–291 CrossRef CAS PubMed.
- I. M. Abu-Reidah, Á. Gil-Izquierdo, S. Medina and F. Ferreres, Food Res. Int., 2017, 100, 494–500 CrossRef CAS PubMed.
- E. A. Brisibe, U. E. Umoren, F. Brisibe, P. M. Magalhäes, J. F. S. Ferreira, D. Luthria, X. Wu and R. L. Prior, Food Chem., 2009, 115, 1240–1246 CrossRef CAS.
- H. S. Helland, A. Leufvén, G. B. Bengtsson, J. Skaret, P. Lea and A. Wold, Postharvest Biol. Technol., 2016, 111, 150–160 CrossRef CAS.
- H. Shi, Y. Zhao, J. Sun, L. Yu and P. Chen, J. Food Compos. Anal., 2017, 61, 67–72 CrossRef CAS.
- M. Thomas, A. Badr, Y. Desjardins, A. Gosselin and P. Angers, Food Chem., 2018, 245, 1204–1211 CrossRef CAS PubMed.
- M. Maldini, M. Foddai, F. Natella, G. L. Petretto, J. P. Rourke, M. Chessa and G. Pintore, J. Food Compos. Anal., 2017, 61, 20–27 CrossRef CAS.
- G. Brader, M. D. Mikkelsen, B. A. Halkier and E. T. Palva, Plant J., 2006, 46, 758–767 CrossRef CAS PubMed.
- V. M. Koritsas, J. A. Lewis and G. R. Fenwick, Ann. Appl. Biol., 1991, 118, 209–221 CrossRef.
- J. Wang, Z. Zhao, X. Sheng, H. Yu and H. Gu, LWT--Food Sci. Technol., 2015, 61, 177–183 CrossRef CAS.
- M. V. Palmer, S. P. Yeung and J. P. Sang, J. Agric. Food Chem., 1987, 35, 262–265 CrossRef CAS.
- Y. Li, X. Wang, Y. Wang, Q. Meng, J. Sun and B. Wang, Acta Hortic. Sin., 2011, 38, 1356–1364 CAS.
- J. Kubola and S. Siriamornpun, Food Chem., 2008, 110, 881–890 CrossRef CAS PubMed.
- Z. Amri, F. Zaouay, H. Lazreg-Aref, H. Soltana, A. Mneri, M. Mars and M. Hammami, Int. J. Biol. Macromol., 2017, 104, 174–180 CrossRef PubMed.
- F. G. K. Vieira, G. D. S. C. Borges, C. Copetti, P. F. D. Pietro, E. D. C. Nunes and R. Fett, Sci. Hortic., 2011, 128, 261–266 CrossRef CAS.
- M. Shiraishi, R. Shinomiya and H. Chijiwa, Sci. Hortic., 2018, 227, 272–277 CrossRef CAS.
- H. Sasaki, K. Ichimura, K. Okada and M. Oda, Sci. Hortic., 1998, 76, 161–169 CrossRef CAS.
- S. J. Yang, M. Hosokawa, Y. Mizuta, J. G. Yun, J. Mano and S. Yazawa, Sci. Hortic., 2001, 88, 59–69 CrossRef CAS.
- T. Gigolashvili, M. Engqvist, R. Yatusevich, C. Müller and U. Flügge, New Phytol., 2008, 177, 627–642 CrossRef CAS PubMed.
Footnote |
† These authors contributed equally to this work. |
|
This journal is © The Royal Society of Chemistry 2018 |
Click here to see how this site uses Cookies. View our privacy policy here.