DOI:
10.1039/C8RA05855E
(Paper)
RSC Adv., 2018,
8, 31682-31689
Retracted Article: Down-regulation of Rab10 inhibits hypoxia-induced invasion and EMT in thyroid cancer cells by targeting HIF-1α through the PI3K/Akt pathway
Received
9th July 2018
, Accepted 28th August 2018
First published on 12th September 2018
Abstract
Rab10, a member of the Rab family, is localized to endocytic compartments and serves as a regulator of intracellular vesicle trafficking. Previous studies mainly paid attention to the role of Rab10 in transport. Recently, Rab10 has been reported to be involved in the progression of various cancers. However, the biological functions of Rab10 in thyroid cancer remain unknown. In this study, we demonstrated that Rab10 was highly expressed in thyroid cancer tissues and cell lines. Down-regulation of Rab10 inhibited hypoxia-induced migration, invasion and epithelial–mesenchymal transition (EMT) of thyroid cancer cells. Moreover, HIF-1α and the PI3K/Akt pathway were involved in the inhibitory effect of Rab10 down-regulation on thyroid cancer cell invasion and EMT induced by hypoxia. Taken together, our study provided further evidence to support the role of Rab10 as a therapeutic target for thyroid cancer.
I. Introduction
Thyroid cancer is a common type of tumor related to the endocrine system and its incidence has been steadily rising in the past decades.1–3 This disease mainly originates from follicular cells encompassing a range of differentiation from indolent cancer types (follicular or papillary thyroid cancer) to invasive and lethal cancer types (undifferentiated thyroid cancer).4 This broad spectrum of development is partly linked to epigenetic dysregulation of genes associated with tumor differentiation, aggressive ability and metastatic potential.5 Increasing evidence has demonstrated that the epithelial–mesenchymal transition (EMT) process plays a crucial role in cancer invasion and metastasis.6–8 Therefore, it is desperately needed to explore novel biomarkers related to EMT and investigate the underlying molecular mechanisms for the purpose of facilitating the treatment of thyroid cancer.
The Rab family, belonging to the RAS superfamily of small GTPases, contains more than 60 members.9,10 These members act as a regulator of intercellular vesicle trafficking, receptor recycling and signal transduction.11 These processes, in turn, mediate the normal membrane polarity.12–14 Besides, these Rab GTPases can be activated by distinct types of Rab GEFs and down-regulated by Rab GAPs.15 Rab10, a member of the Rab family, is an important participant in formation of endoplasmic reticulum, secretion of basement membrane and translocation of glucose transporter type 4.16–18 Furthermore, Rab10 plays a crucial role in innate immune responses via regulating transport of toll-like receptor 4.19,20 Recently, Rab10 has been demonstrated to be aberrantly expressed in some types of cancers and show biological significance in cancer progression.21–23 However, the specific role of Rab10 in thyroid cancer remains unknown.
In this study, we demonstrated that Rab10 was elevated in thyroid cancer tissues and cell lines. Down-regulation of Rab10 inhibited hypoxia-induced migration, invasion and EMT of thyroid cancer cells. In addition, we found that Rab10 down-regulation exerted the inhibitory effect on thyroid cancer cells exposed to hypoxia by targeting HIF-1α through the PI3K/Akt pathway.
II Results
Expression of Rab10 is up-regulated in thyroid cancer tissues and cell lines
To investigate the effect of Rab10 on thyroid cancer, we first measured the expression levels of Rab10 mRNA and protein in thyroid cancer tissues and adjacent normal tissues from 42 patients. The results showed that the expression of Rab10 in thyroid cancer tissues was significantly increased at both mRNA and protein levels in comparison with the normal tissues (Fig. 1A and B). The expression of Rab10 was also evaluated in thyroid cancer cell lines. As shown in Fig. 1C and D, Rab10 mRNA and protein levels in WRO and 8505C cell lines were markedly higher than those in the normal thyroid cell line Nthy-ori 3-1.
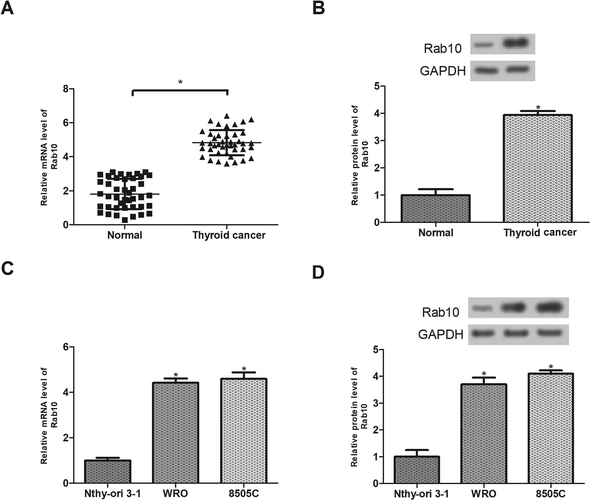 |
| Fig. 1 Expression of Rab10 is up-regulated in thyroid cancer tissues and cell lines. (A and B) RT-PCR and western blot analysis of Rab10 expression in thyroid cancer tissues and adjacent normal tissues. (n = 42). (C and D) RT-PCR and western blot analysis of Rab10 expression in thyroid cancer cell lines (WRO and 8505C) and the normal thyroid cell line Nthy-ori 3-1. n = 3. *p < 0.05. | |
Down-regulation of Rab10 inhibits the viability of thyroid cancer cells exposed to hypoxia
We first examined the effect of hypoxia on thyroid cancer cell viability. As shown in Fig. 2A and B, no obvious effect on the cell viability was observed after hypoxia exposure for 24 h while the viability was significantly reduced after hypoxia exposure for 48 h. Therefore, WRO and 8505C cells were treated with hypoxia for 24 h in the subsequent experiments.
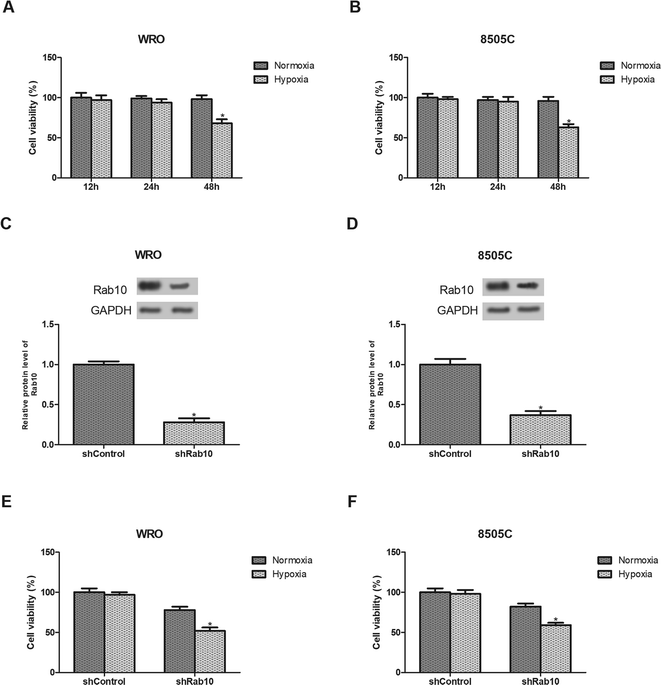 |
| Fig. 2 Down-regulation of Rab10 inhibits the viability of thyroid cancer cells exposed to hypoxia. (A and B) WRO and 8505C cells were cultured under normoxic or hypoxic conditions for indicated times. Cell viability was detected by the MTT assay. (C and D) Rab10 expression levels in WRO and 8505C cells were measured by western blot analysis after transfection of shRab10 or shControl. (E and F) WRO and 8505C cell viability were determined using the MTT assay after transfection and treatment with normoxia or hypoxia. n = 3. *p < 0.05. | |
Next, we decreased the expression of Rab10 in thyroid cancer cells. Down-regulation of Rab10 in WRO and 8505C cells was confirmed by western blot analysis (Fig. 2C and D). Then we detected the effect of Rab10 down-regulation on cell viability under hypoxic conditions. The results showed that the inhibitory effect of Rab10 down-regulation was much stronger on WRO cell viability under hypoxic conditions than those incubated under normoxic conditions (Fig. 2E). Similar results were obtained in 8505C cells (Fig. 2F).
Down-regulation of Rab10 inhibits hypoxia-induced migration, invasion and EMT of thyroid cancer cells
Hypoxia has been demonstrated to induce cancer cell migration and invasion.24 So we detected the effect of Rab10 down-regulation on hypoxia-induced cell migration and invasion. As shown in Fig. 3A and B, hypoxia remarkably increased the migratory and invasive abilities of WRO cells, which were decreased by Rab10 down-regulation. Similarly, we found that down-regulation of Rab10 inhibited hypoxia-induced migration and invasion of 8505C cells (Fig. 3C and D).
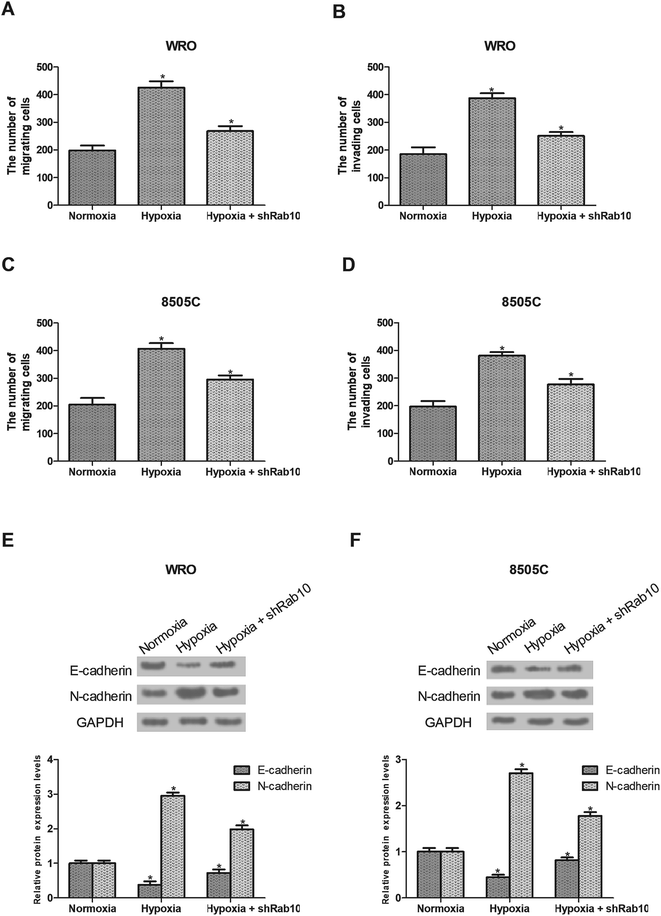 |
| Fig. 3 Down-regulation of Rab10 inhibits hypoxia-induced migration, invasion and EMT of thyroid cancer cells. (A–D) WRO and 8505C cell migration and invasion were detected using the transwell assay. (E and F) The expression levels of E-cadherin and N-cadherin in WRO and 8505C cells were assessed by western blot analysis. n = 3. *p < 0.05. | |
The EMT process plays an essential role in cancer progression and the hypoxic environment can stimulate this process,6–8,25 so we investigated the effect of Rab10 down-regulation on hypoxia-induced EMT in thyroid cancer cells. As shown in Fig. 3E, the expression of E-cadherin was decreased while the expression of N-cadherin was increased after hypoxia treatment in WRO cells. But the EMT process was reversed by Rab10 down-regulation in WRO cells cultured under hypoxic conditions (Fig. 3E). Similar results were found in 8505C cells (Fig. 3F).
HIF-1α and the PI3K/Akt pathway are involved in the inhibitory effect of Rab10 down-regulation on thyroid cancer cell invasion and EMT induced by hypoxia
HIF-1α is known as a regulator of cancer cell invasion and EMT under hypoxic conditions.26–29 So, we investigated whether Rab10 down-regulation exerted the inhibitory effect on hypoxia-induced invasion and EMT by regulating the HIF-1α expression. As expected, Rab10 down-regulation decreased hypoxia-induced HIF-1α expression in WRO cells (Fig. 4A). To further confirm the involvement of HIF-1α in hypoxia-regulated invasion and EMT, HIF-1α shRNA was transfected into the WRO cells and the knockdown efficiency was verified by western blot analysis (Fig. 4B). Moreover, the cell invasion assay showed that HIF-1α knockdown inhibited hypoxia-induced invasion of WRO cells and Rab10 down-regulation enhanced the inhibitory effect (Fig. 4C). We also found that HIF-1α knockdown suppressed hypoxia-induced decrease in E-cadherin expression in WRO cells and the suppressive effect was potentiated by Rab10 down-regulation (Fig. 4D).
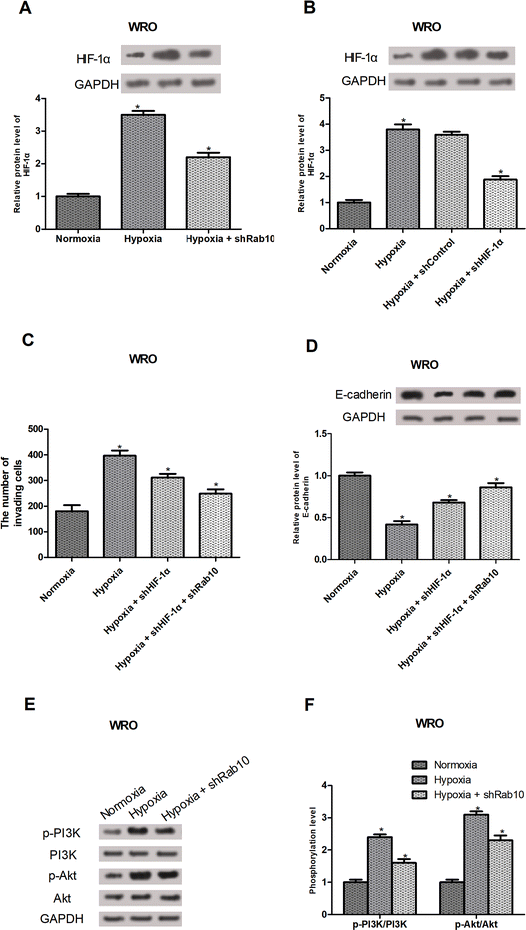 |
| Fig. 4 HIF-1α and the PI3K/Akt pathway are involved in the inhibitory effect of Rab10 down-regulation on thyroid cancer cell invasion and EMT induced by hypoxia. (A and B) Western blot analysis of HIF-1α expression in WRO cells after different treatment. (C) WRO cell invasion was measured using the transwell assay. (D) Western blot analysis of E-cadherin expression in WRO cells. (E) Western blot analysis of the protein expression of p-PI3K, PI3K, p-Akt and Akt in WRO cells. (F) Quantification of the protein expression levels of p-PI3K, PI3K, p-Akt and Akt. n = 3. *p < 0.05. | |
Numerous studies have shown that the PI3K/Akt signaling pathway could regulate the levels of HIF-1α.30,31 Thus, we assumed that Rab10 down-regulation might mediate the expression of HIF-1α via the PI3K/Akt pathway. The results showed that hypoxia treatment obviously increased the expression of p-PI3K and p-Akt in WRO cells, but the effect was significantly impaired by Rab10 down-regulation (Fig. 4E and F).
III. Discussion
According to statistics, over 6% of thyroid cancers are characterized by vigorous migratory and invasive capabilities which are considered crucial signs of a lethal outcome.32,33 Thus, prevention of migration and invasion is a necessity for thyroid cancer therapy.
Rab10, a member of the Rab family, is localized to endocytic compartments and serves as a regulator of intracellular vesicle trafficking.34 Rab10 deletion could decrease cell adhesion and affect a number of cell functions.35 Previous studies mainly paid attention to the role of Rab10 in transport. Recently, Rab10 has been reported to be involved in progression of various cancers. For example, Wang et al. demonstrated the oncogenic role of Rab10 in hepatocellular cancer and its association with the poor prognosis of hepatocellular cancer patients.22 Furthermore, Rab10 has been targeted to inhibit cell migration and invasion in osteosarcoma.23 In this study, we found that Rab10 was highly expressed in thyroid cancer tissues and cell lines, indicating the potential role of Rab10 in thyroid cancer.
The EMT process is an essential step during cancer cell migration and invasion.6–8 A distinct hallmark of EMT is the loss or reduction of E-cadherin expression.36 So far, a variety of EMT inducers have been identified and one of the vital factors is hypoxia.37–41 Tissue hypoxia is caused by the poor development of angiogenic vessels.42 Under hypoxic conditions, cell morphology will change and subsequently lead to a mesenchymal-like phenotype, which is an obvious feature of the EMT process.8 In this study, we found that down-regulation of Rab10 inhibited hypoxia-induced migration, invasion and EMT of thyroid cancer cells. All these observations suggested that Rab10 down-regulation blocked thyroid cancer cell migration and invasion by suppressing EMT under hypoxic conditions.
HIF-1α, a signal transcription factor, is a hallmark of cell invasion and a crucial regulator of the EMT process.26,27,43 HIF-1α could be induced upon hypoxia, which will influence the expression and activity of some important transcription factors and subsequently cause inhibition of E-cadherin in cancer cells.27–29 In this study, we found that Rab10 down-regulation decreased hypoxia-induced HIF-1α expression in thyroid cancer cells; HIF-1α knockdown inhibited hypoxia-induced decrease in E-cadherin expression and the inhibitory effect was enhanced by Rab10 down-regulation. Our findings suggested that Rab10 down-regulation blocked hypoxia-induced EMT in thyroid cancer cells by suppressing HIF-1α expression.
Increasing evidence has demonstrated that the PI3K/Akt pathway could mediate HIF-1α expression in cancer cells. For example, Sun et al. reported that PI3K inhibitors significantly prevented HIF-1α induction under hypoxic conditions in prostate cancer.44 In our study, we found that hypoxia treatment markedly elevated the expression of p-PI3K and p-Akt in thyroid cancer cells and this effect was reversed after Rab10 down-regulation. These results indicated that down-regulation of Rab10 inhibited hypoxia-induced EMT in thyroid cancer cells by reducing HIF-1α expression through regulation of the PI3K/Akt signaling pathway.
In conclusion, we demonstrated that Rab10 was highly expressed in thyroid cancer tissues and cell lines. Down-regulation of Rab10 inhibited hypoxia-induced migration, invasion and EMT of thyroid cancer cells. Moreover, HIF-1α and the PI3K/Akt pathway were involved in the inhibitory effect of Rab10 down-regulation on thyroid cancer cell invasion and EMT induced by hypoxia. Taken together, our study provided further evidence to support the role of Rab10 as a therapeutic target for thyroid cancer.
IV. Materials and methods
Patients and tissue samples
This study was approved by the Ethics Committee of Huaihe Hospital of Henan University (Kaifeng, China). A total of 42 patients with thyroid cancer participated in the study and provided informed consent. None of the patients received any adjuvant therapies before surgery. All thyroid cancer tissues and corresponding adjacent normal tissues were collected from the patients and then stored at −80 °C for future experiments. This study was approved by the Ethics Committee of Huaihe Hospital of Henan University, and written informed consent was obtained from all patients.
Cell lines and cell culture
Human thyroid cancer cell lines (WRO and 8505C) and thyroid follicular epithelial cell line Nthy-ori 3-1 were purchased from the American Type Culture Collection (ATCC, Manassas, VA, USA). All cell lines were cultured in RPMI-1640 (Gibco, Rockville, MD, USA) containing 10% fetal bovine serum (FBS; Gibco) and 100 U ml−1 penicillin/streptomycin and incubated at 37 °C in a standard humidified atmosphere with 5% CO2.
To induce hypoxia, cells were seeded in a six-well plate and continuously gassed with 5% CO2 and 1% O2 (balanced with N2) at 37 °C in a sealed incubator (Thermo Fisher Scientific, Waltham, MA, USA) for different time. Control cells were cultured under a normoxic condition (5% CO2 and 21% O2) at room temperature.
Quantitative real-time polymerase chain reaction (qRT-PCR)
Total RNA was isolated from tissues or cells using TRIzol reagent (Invitrogen, Carlsbad, CA, USA). An equal amount of sample was reversely transcribed using M-MLV Reverse Transcriptase (Invitrogen). The RT-PCR was performed using a PTC-200 PCR system (Bio-Rad, Hercules, CA, USA) under the following condition: 95 °C for 10 min, 40 cycles of 95 °C for 10 s, 55 °C for 10 s and 72 °C for 10 min. The primers were: Rab10, 5′-TTTCACACCATCACAACCTCC-3′ (forward) and 5′-GGTACAACTCTTTTGTCGTCCATA-3′ (reverse); GAPDH, 5′-TGACTTCAACAGCGACACCCA-3′ (forward) and 5′-CACCCTGTTGCTGTAGCCAAA-3′ (reverse). GAPDH was used as an internal control. The relative expression was calculated using the 2−ΔΔCt method.
Western blot analysis
Tissues or cells were lysed with lysis buffer and the protein lysates were separated by 12% SDS-PAGE and then transferred onto PVDF membranes (Millipore, Billerica, MA, USA). After blocking in 5% non-fat milk for 2 h, the membranes were incubated with the primary antibodies against Rab10 (1:1000), E-cadherin (1:1000), N-cadherin (1:1000), HIF-1α (1:1000), p-PI3K (1:2000), PI3K (1:2000), p-Akt (1:2000), Akt (1:2000) and GAPDH (1:2000). Subsequently, the membranes were washed and incubated with appropriate secondary antibodies. All antibodies were obtained from Invitrogen. The immunoreactive bands were detected using an enhanced chemiluminescence kit (Pierce, Rockford, IL, USA) and quantified using the Quantity One software.
Cell transfection
The shRNA against Rab10 or HIF-1α was purchased from GeneCopoeia (Rockville, MD, USA). A non-specific shRNA was used as a negative control. All the shRNA sequences were as follows: shRab10, 5′-GCTACAGCGAGTACGTTTACCT-3′; shHIF-1α, 5′-CCTATATCCCAATGGATGA-3′; shControl, 5′-GCTACAGCGAGTACGTTTACCT-3′. Cells were transfected with shRab10, shHIF-1α or shControl using Lipofectamine 2000 reagent (Invitrogen) according to the manufacturer's instructions. The transfection efficiency was confirmed by western blot analysis.
Cell viability assay
The effect of hypoxia on cell viability was determined by the MTT assay. In brief, cells (1 × 104 per well) were exposed to hypoxia for indicated times in a 96-well plate. Then MTT solution (5 mg ml−1; Sigma, St. Louis, MO, USA) was added to each well and cells were further cultured for 4 h at 37 °C. Subsequently, the culture medium was removed and DMSO (Sigma) was added. The optical density was measured at 570 nm using a microplate reader.
Transwell assay
Transwell chambers were used to assess cell migration and invasion. 24-well plates with Matrigel-free inserts were for cell migration and those with Matrigel-coated inserts were for cell invasion. Briefly, the upper chamber was filled with serum-free medium and 2 × 104 cells were added. Culture medium with 10% FBS was added to the lower chamber as a chemoattractant. After incubation for 24 h, cells on the upper surface of the insert were removed and cells on the lower surface of the insert were fixed and stained with 0.1% crystal violet. The number of cells in five random fields was counted under a microscope (400×).
Statistical analysis
Data were collected from three independent experiments and shown as means ± standard deviation (SD). Statistical analysis was performed using SPSS 19.0 software. Student's t-test or one-way ANOVA was used for the comparison between different groups. Differences were considered statistically significant when the p value was less than 0.05.
Conflicts of interest
The authors declare that they have no conflicts of interest.
Acknowledgements
None.
References
- A. Jemal, R. Siegel, J. Xu and E. Ward, Cancer statistics, Ca-Cancer J. Clin., 2011, 61, 69–90 CrossRef PubMed.
- A. Y. Chen, A. Jemal and E. M. Ward, Increasing incidence of differentiated thyroid cancer in the United States, 1988–2005, Cancer, 2009, 115, 3801–3807 CrossRef PubMed.
- X. Wang, W. Cheng, J. Li, A. Su, T. Wei, F. Liu and J. Zhu, Endocrine tumours: familial nonmedullary thyroid carcinoma is a more aggressive disease: a systematic review and meta-analysis, Eur. J. Endocrinol., 2015, 172, R253–R262 Search PubMed.
- T. Kondo, S. Ezzat and S. L. Asa, Pathogenetic mechanisms in thyroid follicular-cell neoplasia, Nat. Rev. Cancer, 2006, 6, 292–306 CrossRef PubMed.
- T. Kondo, S. Asa and S. Ezzat, Epigenetic Dysregulation in Thyroid Neoplasia, Endocrinol. Metab. Clin. North Am., 2008, 37, 389–400 CrossRef PubMed.
- J. Yang and R. A. Weinberg, Epithelial-mesenchymal transition: at the crossroads of development and tumor metastasis, Dev. Cell, 2008, 14, 818–829 CrossRef PubMed.
- M. A. Huber, N. Kraut and H. Beug, Molecular requirements for epithelial–mesenchymal transition during tumor progression, Curr. Opin. Cell Biol., 2005, 17, 548–558 CrossRef PubMed.
- J. P. Thiery, Epithelial-mesenchymal transitions in tumour progression, Nat. Rev. Cancer, 2002, 2, 442–454 CrossRef PubMed.
- A. M. Rojas, G. Fuentes, A. Rausell and A. Valencia, The Ras protein superfamily: evolutionary tree and role of conserved amino acids, J. Cell Biol., 2012, 196, 189–201 CrossRef PubMed.
- J. Cherfils and M. Zeghouf, Regulation of small GTPases by GEFs, GAPs, and GDIs, Physiol. Rev., 2013, 93, 269–309 CrossRef PubMed.
- H. Stenmark and V. M. Olkkonen, The Rab GTPase family, Genome Biol., 2001, 2, REVIEWS3007 CrossRef PubMed.
- Y. Kitagishi and S. Matsuda, RUFY, Rab and Rap Family Proteins Involved in a Regulation of Cell Polarity and Membrane Trafficking, Int. J. Mol. Sci., 2013, 14, 6487–6498 CrossRef PubMed.
- M. S. Ioannou and P. S. Mcpherson, Rab-mediated membrane trafficking and the control of epithelial cell polarity, J. Cell Biol., 2016, 213, 301–303 CrossRef PubMed.
- F. R. Kiral, F. E. Kohrs, E. J. Jin and P. R. Hiesinger, Rab GTPases and Membrane Trafficking in Neurodegeneration, Curr. Biol., 2018, 28, R471–R486 CrossRef PubMed.
- L. Goitre, E. Trapani, L. Trabalzini and S. F. Retta, The Ras Superfamily of Small GTPases: The Unlocked Secrets, Methods Mol. Biol., 2014, 1120, 1–18 CrossRef PubMed.
- A. R. English and G. K. Voeltz, Rab10 GTPase regulates ER dynamics and morphology, Nat. Cell Biol., 2013, 15, 169–178 CrossRef PubMed.
- D. W. Lerner, D. Mccoy, A. J. Isabella, A. P. Mahowald, G. F. Gerlach, T. A. Chaudhry and S. Hornebadovinac, A Rab10-dependent mechanism for polarized basement membrane secretion during organ morphogenesis, Dev. Cell, 2013, 24, 159–168 CrossRef PubMed.
- H. Sano, G. R. Peck, A. N. Kettenbach, S. A. Gerber and G. E. Lienhard, Insulin-stimulated GLUT4 Protein Translocation in Adipocytes Requires the Rab10 Guanine Nucleotide Exchange Factor Dennd4C, J. Biol. Chem., 2011, 286, 16541–16545 CrossRef PubMed.
- M. D. Barbosa, S. A. Johnson, K. Achey, M. J. Gutierrez, E. K. Wakeland, M. Zerial and S. F. Kingsmore, The Rab Protein Family: Genetic Mapping of Six Rab Genes in the Mouse, Genomics, 1995, 30, 439–444 CrossRef PubMed.
- Y. T. Chen, C. Holcomb and H. P. Moore, Expression and localization of two low molecular weight GTP-binding proteins, Rab8 and Rab10, by epitope tag, Proc. Natl. Acad. Sci. U. S. A., 1993, 90, 6508–6512 CrossRef.
- H. He, F. Dai, L. Yu, X. She, Y. Zhao, J. Jiang, X. Chen and S. Zhao, Identification and characterization of nine novel human small GTPases showing variable expressions in liver cancer tissues, Gene Expression, 2002, 10, 231–242 CrossRef PubMed.
- W. Wang, W. D. Jia, B. Hu and Y. Y. Pan, RAB10 overexpression promotes tumor growth and indicates poor prognosis of hepatocellular carcinoma, Oncotarget, 2017, 8, 26434–26447 Search PubMed.
- W. Jiang, J. Liu, T. Xu and X. Yu, MiR-329 suppresses osteosarcoma development by downregulating Rab10, FEBS Lett., 2016, 590, 2973–2981 CrossRef PubMed.
- N. Burrows, J. Resch, R. L. Cowen, W. R. Von, C. Hoangvu, C. M. West, K. J. Williams and G. Brabant, Expression of hypoxia-inducible factor 1 alpha in thyroid carcinomas, Endocr.-Relat. Cancer, 2010, 17, 61–72 Search PubMed.
- Z. Zhou, S. Wang, C. Song and Z. Hu, Paeoniflorin prevents hypoxia-induced epithelial–mesenchymal transition in human breast cancer cells, OncoTargets Ther., 2016, 9, 2511–2518 CrossRef PubMed.
- D. Luo, Z. Wang, J. Wu, C. Jiang and J. Wu, The Role of Hypoxia Inducible Factor-1 in Hepatocellular Carcinoma, BioMed Res. Int., 2014, 2014, 409272 Search PubMed.
- G. Zhou, L. A. Dada, M. Wu, A. Kelly, H. Trejo, Q. Zhou, J. Varga and J. I. Sznajder, Hypoxia-induced alveolar epithelial-mesenchymal transition requires mitochondrial ROS and hypoxia-inducible factor 1, Am. J. Physiol.: Lung Cell. Mol. Physiol., 2009, 297, 1120–1130 CrossRef PubMed.
- A. V. Salnikov, L. Liu, M. Platen, J. Gladkich, O. Salnikova, E. Ryschich, J. Mattern, G. Moldenhauer, J. Werner and P. Schemmer, Hypoxia Induces EMT in Low and Highly Aggressive Pancreatic Tumor Cells but Only Cells with Cancer Stem Cell Characteristics Acquire Pronounced Migratory Potential, PLoS One, 2012, 7, e46391 CrossRef PubMed.
- Z. Lin, H. Gang, X. Li, Y. Zhang, J. Yan, J. Shen, L. Jia, Q. Wang, Z. Jin and X. Feng, Hypoxia induces epithelial-mesenchymal transition via activation of SNAI1 by hypoxia-inducible factor-1α in hepatocellular carcinoma, BMC Cancer, 2013, 13, 108 CrossRef PubMed.
- B. H. Jiang, G. Jiang, J. Z. Zheng, Z. Lu, T. Hunter and P. K. Vogt, Phosphatidylinositol 3-kinase signaling controls levels of hypoxia-inducible factor 1, Cell Growth Differ., 2001, 12, 363–369 Search PubMed.
- H. Zhong, K. Chiles, D. Feldser, E. Laughner, C. Hanrahan, M. M. Georgescu, J. W. Simons and G. L. Semenza, Modulation of hypoxia-inducible factor 1alpha expression by the epidermal growth factor/phosphatidylinositol 3-kinase/PTEN/AKT/FRAP pathway in human prostate cancer cells: implications for tumor angiogenesis and therapeutics, Cancer Res., 2000, 60, 1541–1545 Search PubMed.
- R. M. Tuttle, D. W. Ball, D. Byrd, R. A. Dilawari, G. M. Doherty, Q. Y. Duh, H. Ehya, W. B. Farrar, R. I. Haddad and F. Kandeel, Thyroid carcinoma, J. Natl. Compr. Cancer Network, 2010, 8, 1228–1274 CrossRef.
- D. K. Robie, C. W. Dinauer, R. M. Tuttle, D. T. Ward, R. Parry, D. Mcclellan, R. Svec, C. Adair and G. Francis, The impact of initial surgical management on outcome in young patients with differentiated thyroid cancer, J. Pediatr. Surg., 1998, 33, 1134–1138 CrossRef PubMed.
- N. J. Pavlos and R. Jahn, Distinct yet overlapping roles of Rab GTPases on synaptic vesicles, Small GTPases, 2011, 2, 77–81 CrossRef PubMed.
- A. J. Lindsay, F. Jollivet, C. P. Horgan, A. R. Khan, G. Raposo, M. W. Mccaffrey and B. Goud, Identification and characterization of multiple novel Rab-myosin Va interactions, Mol. Biol. Cell, 2013, 24, 3420–3434 CrossRef PubMed.
- C. B. De and G. Berx, Regulatory networks defining EMT during cancer initiation and progression, Nat. Rev. Cancer, 2013, 13, 97–110 CrossRef PubMed.
- M. Książkiewicz, A. Markiewicz and A. J. Zaczek, Epithelial-mesenchymal transition: a hallmark in metastasis formation linking circulating tumor cells and cancer stem cells, Pathobiology, 2012, 79, 195–208 CrossRef PubMed.
- J. Zavadil and E. P. Böttinger, TGF-beta and epithelial-to-mesenchymal transitions, Oncogene, 2005, 24, 5764–5774 CrossRef PubMed.
- J. Jiang, Y. L. Tang and X. H. Liang, EMT: a new vision of hypoxia promoting cancer progression, Cancer Biol. Ther., 2011, 11, 714–723 CrossRef PubMed.
- D. T. Marieegyptienne, I. Lohse and R. P. Hill, Cancer stem cells, the epithelial to mesenchymal transition (EMT) and radioresistance: potential role of hypoxia, Cancer Lett., 2013, 341, 63–72 CrossRef PubMed.
- G. L. Semenza, Hypoxia-inducible factors: mediators of cancer progression and targets for cancer therapy, Trends Pharmacol. Sci., 2012, 33, 207–214 CrossRef PubMed.
- R. Rajaganeshan, R. Prasad, P. J. Guillou, G. Poston, N. Scott and D. G. Jayne, The role of hypoxia in recurrence following resection of Dukes' B colorectal cancer, Int. J. Colorectal. Dis., 2008, 23, 1049–1055 CrossRef PubMed.
- C. Tan, L. Zhang, X. Cheng, X. F. Lin, R. R. Lu, J. D. Bao and H. X. Yu, Curcumin inhibits hypoxia-induced migration in K1 papillary thyroid cancer cells, Exp. Biol. Med., 2015, 240, 925–935 CrossRef PubMed.
- H. L. Sun, Y. N. Liu, Y. T. Huang, S. L. Pan, D. Y. Huang, J. H. Guh, F. Y. Lee, S. C. Kuo and C. M. Teng, YC-1 inhibits HIF-1 expression in prostate cancer cells: contribution of Akt/NF-kappaB signaling to HIF-1alpha accumulation during hypoxia, Oncogene, 2007, 26, 3941–3951 CrossRef PubMed.
Footnote |
† These authors contributed equally to this work. |
|
This journal is © The Royal Society of Chemistry 2018 |
Click here to see how this site uses Cookies. View our privacy policy here.