DOI:
10.1039/C8RA06938G
(Paper)
RSC Adv., 2018,
8, 40446-40453
A straightforward and convenient synthesis of functionalized allyl thiosulfonates and allyl disulfanes†
Received
19th August 2018
, Accepted 17th November 2018
First published on 4th December 2018
Abstract
A practical, highly flexible and eco-friendly method has been developed for the synthesis of allyl thiosulfonates using Morita–Baylis–Hillman (MBH) allyl bromides and sodium arylthiosulfonates, which were readily assembled without any reagent/catalyst. Moreover, the allyl thiosulfonates were successfully transformed into a set of two synthetically viable diallyl disulfanes and unsymmetrical allyl disulfanes in the presence of Cs2CO3. The present protocols are operationally simple and convenient to generate a wide range of functionalized allyl thiosulfonates and allyl disulfanes in good to excellent yields.
Introduction
Organosulfur compounds have received much attention in recent years.1 The direct construction of a carbon–sulfur bond is a potent method in modern organic synthesis.2 Among a variety of organosulfur compounds, the thiosulfonates (RS–SO2R1)3 and the disulfanes (RS–SR1)4 are a privileged class of compounds and they serve as electrophilic sulfenylating agents in organic synthesis. Additionally, the thiosulfonates and the disulfanes have several advantages, which include being an odourless sulfur source, higher reactivity, and better stability than other frequently used sulfenylating agents. In general, the thiosulfonates have shown a broad spectrum of clinical properties like antiviral, antimicrobial and fungicidal activities.5 A wide range of effective strategies have been established for the synthesis of S-aryl arenethiosulfonates.6–8 Although the reactions of sodium thiosulfonates with alkyl halides have been studied,9 a general method is still highly desirable. This fact motivated us to develop a new strategy for the synthesis of functionalized allyl thiosulfonates.
In contrast, the disulfanes also have significant importance in pharmaceutical chemistry10 and chemical biology.11 The S–S bond plays an indispensable role in allium compounds10a and disulfiram,10b–d which act as anti-thrombotic and anti-alcoholic drugs (Scheme 1). Numerous synthetic methods have been reported, which rely on the oxidative coupling of thiols to construct the S–S bond.12 However, these methods have some disadvantages, either they involve the tedious use of thiols or their equivalents or they are limited by commercial scarcity. Therefore, it is still necessary to develop a facile methodology for the synthesis of allyl disulfides from easily accessible starting materials.
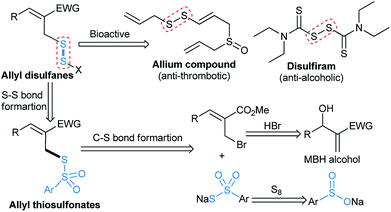 |
| Scheme 1 Strategy for synthesis of allyl thiosulfonates and allyl disulfanes. | |
According to retrosynthetic analysis, the proposed strategy has a great advantage of extensively available starting materials (Scheme 1). In fact, the allyl bromides can be derived from Morita–Baylis–Hillman (MBH) adducts13 and sodium thiosulfonates easily prepared from sulfinate salts.14 In particular, the sodium thiosulfonate precursors are preferable to use as sulfenylating agents since they are bench stable and generally crystalline solids with low toxicity.14b We have also envisaged that the allyl thiosulfonates would be converted into the corresponding unprecedented allyl disulfanes. In a continuation of our interest in organosulfur chemistry,15 we decided to study MBH bromide with sodium thiosulfonate for direct C–S bond construction, which represents an interesting and challenging endeavour in organic synthesis. In this context, we disclose our findings and report the scope for the synthesis of unsymmetrical allyl thiosulfonates and allyl disulfanes with a variety of substitution patterns.
Results and discussion
At the outset, optimization studies were undertaken (see ESI†) for the nucleophilic substitution reaction of (Z)-methyl 2-(bromo-methyl)-3-phenylacrylate (1a) with sodium thiosulfonate (2a) as a model substrate. To our delight, an excellent yield (94%) of the desired allyl thiosulfonate (3aa) was achieved with 1.5 equiv. of 2a in CH3CN at room temperature within 2 h. Then we explored a broad range of allyl bromides (1a–t) with sodium thiosulfonates (2a–d) for the synthesis of allyl thiosulfonates 3aa–tb (Table 1). In addition to 3aa, the 4-bromo and 4-chloro-substituted allyl bromides (1b and 1c) readily reacted with 2a, giving the desired thiosulfonates (3ba–ca) in 89% and 84% yields, respectively. Various aryl-substituted MBH bromides (1a–h) smoothly reacted with sodium 4-methylbenzenesulfonothioate 2b to provide the desired products (3ab–hb) in 70–92% yield. The 2-naphthyl derived thiosulfonates (3ia and 3jb) were obtained in high yield under optimised reaction conditions. Heteroaryl derived allyl bromide (1k) also served as a good substrate, affording 3kb in 68% yield. Moreover, cinnamyl and isovaleraldehyde derived allyl bromides (1l–m) were also suitable substrates to produce the desired allyl thiosulfonates (3lb and 3mb) in high yields. The ethyl ester derived MBH bromides (1n and 1o) provided the corresponding products 3nb and 3ob in 78% and 72% yields, respectively. Disappointingly, the nitrostyrene derived bromide (1p) was sluggish to react with 2a. The substrate scope was further extended to acrylonitrile derived MBH bromides (1q–t). This successfully achieved the corresponding thiosulfonates (3qa/b, 3(r–t)b) in moderate to good yields with inseparable regioisomers (ESI†).16 Overall, these reactions were scalable to provide the desired allyl thiosulfonates with a little deviation in the outcome (ESI†).
Table 1 Substrate scope for synthesis of allyl thiosulfonatesa,b

|
All reactions performed on 0.4 mmol of 1a–t (1 eq.), sodium thiosulfonates 2a–d (1.5 eq.) in CH3CN (2 mL) at room temperature. Isolated yields. Reactions performed on a 4 mmol scale for synthesis of 3aa/b and 2 mmol scale for synthesis of 3ba, (3b–e, g–i, k, n, o, q)b and 3ad (see ESI). |
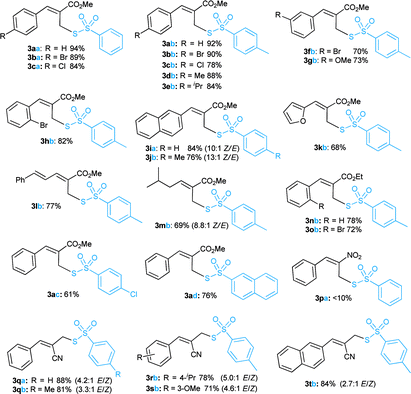 |
Next, we explored the synthetic utility of readily accessible allyl thiosulfonates in organic synthesis. We desired to synthesise methyl 2H-thiochromene-3-carboxylate (4)17 via the cyclization of 2-bromo derived thiosulfonate 3hb (Scheme 2). Unfortunately, we did not attain the anticipated cyclic product 4; however, other interesting allyl disulfanes were obtained under these conditions (Table 2). Hence, we directed our attention toward the synthesis of allyl disulfanes using allyl thiosulfonates.
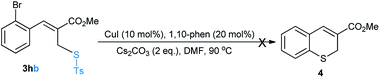 |
| Scheme 2 Synthesis of methyl 2H-thiochromene-3-carboxylate (4). | |
Table 2 Optimization for synthesis of allyl disulfanes from thiosulfonate 3aba

|
Entry |
Reaction conditions |
Time |
5ab |
6ab |
All reactions performed on a 0.4 mmol scale of 3ab in solvent (2 mL). Isolated yields. NR: no reaction. |
1 |
CuI (10 mol%), 1,10-phen (20 mol%), Cs2CO3 (2 eq.), DMF, 90 °C |
6 h |
20% |
14% |
2 |
CuBr (10 mol%), 1,10-phen (20 mol%), Cs2CO3 (2 eq.), DMF, 90 °C |
6 h |
29% |
20% |
3 |
CuBr (10 mol%), 1,10-phen (20 mol%), K2CO3 (2 eq.), DMF, 90 °C |
6 h |
NR |
NR |
4 |
CuBr (10 mol%), 1,10-phen (20 mol%), Cs2CO3 (2 eq.), toluene, 90 °C |
6 h |
34% |
11% |
5 |
CuBr (10 mol%), 1,10-phen (20 mol%), Cs2CO3 (2 eq.), THF, 60 °C |
4 h |
30% |
20% |
6 |
CuBr (10 mol%), Cs2CO3 (2 eq.), THF, 60 °C |
4 h |
35% |
22% |
7 |
CuBr (5 mol%), Cs2CO3 (2 eq.), THF, 60 °C |
4 h |
38% |
24% |
8 |
CuBr (10 mol%), THF, 60 °C |
6 h |
NR |
NR |
9 |
Cs2CO3 (2 eq.), THF, 60 °C |
2 h |
45% |
36% |
10 |
Cs2CO3 (1 eq.), THF, 60 °C |
2 h |
49% |
44% |
11 |
Cs2CO3 (0.5 eq.), THF, 60 °C |
4 h |
38% |
29% |
12 |
DBU (2 eq.), THF, 60 °C |
6 h |
20% |
20% |
13 |
DABCO (2 eq.), THF, 60 °C |
6 h |
NR |
NR |
14 |
K2CO3 (2 eq.), THF, 60 °C |
6 h |
NR |
NR |
15 |
Without base, THF, 60 °C |
6 h |
NR |
NR |
Encouraged by this result, we commenced the optimization of reaction conditions using thiosulfonates 3ab (Table 2). The use of CuI (10 mol%) and CuBr (10 mol%) with 1,10-phen (20 mol%) in the presence of Cs2CO3 (2 eq.) in DMF afforded the desired disulfanes (5a and 6a) in low yield (entries 1 and 2). There was no reaction in the presence of K2CO3 in DMF; however, substantial progress was seen in toluene and THF (entries 3–5). In the absence of a ligand (1,10-phen), the reaction proceeded with 5 mol% of CuBr, but there was no reaction with only CuBr and without Cs2CO3 (entries 6–8). To our surprise, Cs2CO3 alone afforded the desired disulfanes with improved yields (entries 9–11). A satisfactory yield of diallyl disulfane 5a (49%) and unsymmetrical disulfane 6a (44%) were obtained when the reaction was carried out using 1.0 eq. of Cs2CO3 in THF at 60 °C (entry 10). Low yields were obtained with DBU, DABCO, and K2CO3 (entries 12–14) and no reaction was found without a base (entry 15). It is worth noting that our attempts to produce as a single product either 5a or 6a were unsuccessful.
With the optimized conditions in hand, the scope of the reaction was further verified and the results are summarized in Table 3. Pleasingly, the allyl thiosulfonate substrates (3a, d, e, g, h, o/b and 3aa/b) bearing different groups at the para, meta or ortho position of the aromatic rings proceeded efficiently to afford the corresponding allyl disulfanes (5a–g and 6a–h) in good to high yields. The bulky 2-naphthalene derived thiosulfonates (3jb/3ad) were also well tolerated to provide the diallyl disulfanes (5h/a) and unsymmetrical disulfanes (6i/j) in good yields. The ethyl ester derived thiosulfonate (3nb) was also a good substrate to furnish allyl disulfane 5i and 6k in 40% and 35% yields, respectively. But an extension to other thiosulfonates 3kb and 3qb failed to provide the desired allyl disulfides.
Table 3 Substrate scope for synthesis of allyl disulfanesa,b

|
All reactions performed on 0.8 mmol of allyl thiosulfonates (1 eq.) and Cs2CO3 (1.0 eq.) in dry THF (4 mL) at 60 °C for 2 h. Isolated yields. |
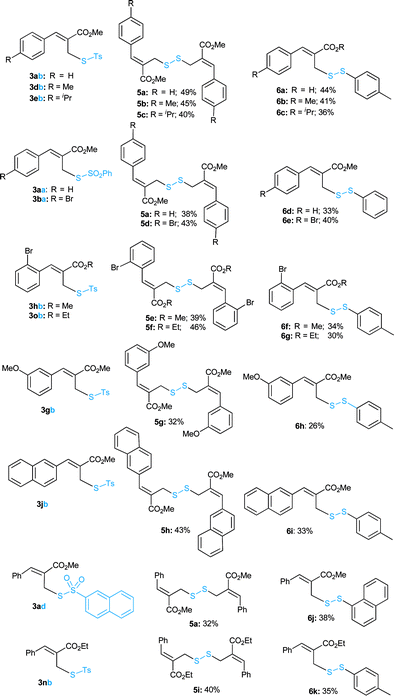 |
Recently, Jiang and co-workers18 demonstrated the potential application of S-acetyl disulfanes in oxidative coupling with arylboronic acids. In this direction, the allyl thiosulfonates 3(a/h)b were treated with potassium thioacetate in DMF and the anticipated products (7a/b) were achieved in 88% and 85% yields, respectively (Scheme 3). Sequential one-pot nucleophilic substitution reactions were performed using MBH bromide 1a with sodium thiosulfonate (2b) followed by potassium thioacetate (Scheme 3). The desired product 7a was obtained in 78% yield and this procedure has notable advantages, such as straightforwardness, step-economy and scalability in a one-pot operation (see ESI†).
 |
| Scheme 3 Reactions performed on 0.8 mmol of 3ab/3hb with KSAc (1.5 eq.). A gram scale reaction of allyl bromide 1a with 2b/KSAc for the synthesis of 7a. | |
To gain more insight, the S-aryl arenethiosulfonates (8a and 8b) were treated with Cs2CO3 under the same reaction conditions. Thus, it was found that no reaction representing the allyl moiety played a vital role in the formation of disulfanes. A radical-trapping experiment was also performed with 3ab using TEMPO (2 equiv.) as a radical scavenger under standard conditions. The disulfanes 5a and 6a were isolated in low yields, alongside the TEMPO derived product (9),4d as detected by HRMS (Scheme 4b). These experimental results indicate that the reaction may proceed via a radical pathway leading to the formation of allyl disulfanes 5 and 6.
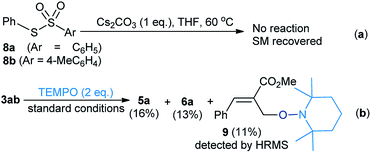 |
| Scheme 4 Control experiments. | |
Experimental
General procedure-1 (GP1) for synthesis of allyl thiosulfonates
A heat gun-dried Schlenk tube was charged with Morita–Baylis–Hillman allyl bromides 1a–t (0.4 mmol, 1.0 equiv.) and sodium thiosulfonates 2a–d (0.6 mmol, 1.5 equiv.) in CH3CN (2.0 mL). The reaction mixture was stirred at room temperature for 2 h and monitored by TLC until it was either complete or appeared to be making no further progress. The mixture was quenched by the addition of water (10 mL) followed by extraction with EtOAc (3 × 10 mL). The combined organic layers were washed with brine (2 × 10 mL), dried over anhydrous Na2SO4, and the solvent was removed under reduced pressure. The resulting residue was subjected to flash chromatography (silica gel, eluted with 20–30% ethyl acetate/petether) to afford the desired allyl thiosulfonates.
Allyl thiosulfonate 3aa
Prepared according to GP1 using 1a (101.2 mg, 0.4 mmol) with sodium benzenethiosulfonate 2a (117.6 mg, 0.6 mmol) to afford 3aa (130.6 mg, 94%) using 20% ethyl acetate/petether as a colorless solid. Mp: 95–97 °C; 1H NMR (400 MHz, CDCl3) δ 7.87–7.77 (m, 3H), 7.63 (t, J = 7.4 Hz, 1H), 7.51 (t, J = 7.8 Hz, 2H), 7.42–7.32 (m, 5H), 4.17 (s, 2H), 3.75 (s, 3H); 13C NMR (101 MHz, CDCl3) δ 166.8, 144.6, 144.3, 134.0, 133.7, 129.8, 129.7 (2C), 129.4 (2C), 129.0 (2C), 127.2 (2C), 124.4, 52.6, 33.4; HRMS (ESI) calculated for C17H16O4S2Na [M + Na]+: m/z 371.0388, found 371.0389. Prepared according to GP1 using 1a (1.02 g, 4.0 mmol) with sodium benzenethiosulfonate 2a (1.47 g, 6.0 mmol) in CH3CN (20 mL) to afford 3aa (1.1 g, 79%) as a colorless solid.
General procedure-2 (GP2) for synthesis of allyl disulfanes
A heat gun-dried Schlenk tube was charged with allyl thiosulfonates 3 (0.8 mmol, 1.0 equiv.) and cesium carbonate (260.6 mg, 0.8 mmol, 1.0 equiv.) in dry THF (4.0 mL). The reaction mixture was stirred at 60 °C for 2 h and monitored by TLC either until it was complete or appeared to be making no further progress. The mixture was allowed to cool to room temperature and quenched by the addition of water (20 mL) followed by extraction with EtOAc (3 × 30 mL). The combined organic layers were washed with brine (2 × 20 mL), dried over anhydrous Na2SO4, and the solvent was removed under reduced pressure. The resulting residue was subjected to flash chromatography (silica gel, eluted with 20–30% ethyl acetate/petether) to afford desired allyl disulfanes 5 and 6.
Diallyl disulfane 5a
Prepared according to GP2 using 3ab (289.6 mg, 0.8 mmol) with Cs2CO3 (260.6 mg, 0.8 mmol) to afford 5a (81.2 mg, 24.5% × 2 = 49%) using 20% ethyl acetate/petether as a colorless solid. Mp: 110–112 °C; 1H NMR (400 MHz, CDCl3) δ 7.82 (s, 1H), 7.51 (dd, J = 7.3, 1.0 Hz, 2H), 7.41–7.34 (m, 3H), 3.88 (s, 2H), 3.81 (s, 3H); 13C NMR (101 MHz, CDCl3) δ 167.6, 142.4, 134.9, 129.7 (2C), 129.2, 128.8 (2C), 128.0, 52.4, 36.7; HRMS (ESI) calculated for C22H22O4S2Na [M + Na]+: m/z 437.0857, found 437.0857.
Allyl disulfane 6a
Prepared according to GP2 using 3ab (289.6 mg, 0.8 mmol) with Cs2CO3 (260.6 mg, 0.8 mmol) to afford 6a (116.2 mg, 44%) using 30% ethyl acetate/petether as a colorless solid. Mp: 163–165 °C. 1H NMR (400 MHz, CDCl3) δ 7.93 (s, 1H), 7.71 (d, J = 8.3 Hz, 2H), 7.50–7.43 (m, 2H), 7.40–7.33 (m, 3H), 7.27 (d, J = 8.0 Hz, 2H), 4.47 (s, 2H), 3.61 (s, 3H), 2.42 (s, 3H); 13C NMR (101 MHz, CDCl3) δ 167.1, 146.3, 145.5, 144.9, 136.5, 133.8, 129.8 (2C), 129.3 (2C), 128.8 (2C), 128.7 (2C), 121.2, 55.3, 52.5, 21.7; HRMS (ESI) calculated for C18H18O4S2Na [M + Na]+: m/z 353.0823, found 353.0829.
Diallyl disulfane 5b
Prepared according to GP2 using 3db (300.6 mg, 0.8 mmol) with Cs2CO3 (260.6 mg, 0.8 mmol) to afford 5b (80.3 mg, 22.7% × 2 = 45%) using 20% ethyl acetate/petether as a pale-yellow solid. Mp: 197–199 °C; 1H NMR (400 MHz, CDCl3) δ 7.87–7.74 (m, 1H), 7.45 (d, J = 8.1 Hz, 2H), 7.19 (d, J = 8.0 Hz, 2H), 3.97 (s, 2H), 3.81 (s, 3H), 2.37 (s, 3H); 13C NMR (101 MHz, CDCl3) δ 167.7, 142.5, 139.6, 131.9, 129.9 (2C), 129.5 (2C), 126.9, 52.3, 37.1, 21.5; HRMS (ESI) calculated for C24H26O4S2Na [M + Na]+: m/z 465.1170, found 465.1172.
Allyl disulfane 6b
Prepared according to GP2 using 3db (300.6 mg, 0.8 mmol) with Cs2CO3 (260.6 mg, 0.8 mmol) to afford 6b (112.7 mg, 41%) using 30% ethyl acetate/petether as a pale-yellow solid. Mp: 212–214 °C; 1H NMR (400 MHz, CDCl3) δ 7.90 (s, 1H), 7.72 (d, J = 8.2 Hz, 2H), 7.41 (d, J = 8.0 Hz, 2H), 7.27 (d, J = 8.0 Hz, 2H), 7.18 (d, J = 8.0 Hz, 2H), 4.48 (s, 2H), 3.58 (s, 3H), 2.41 (s, 3H), 2.37 (s, 3H); 13C NMR (101 MHz, CDCl3) δ 167.2, 146.4, 144.8, 140.2, 136.5, 130.9, 129.6 (2C), 129.50 (2C), 129.48 (2C), 128.6 (2C), 120.0, 55.3, 52.3, 21.7, 21.5; HRMS (ESI) calculated for C19H20O2S2Na [M + Na]+: m/z 367.06980; found 367.06980.
Diallyl disulfane 5c
Prepared according to GP2 using 3eb (323.2 mg, 0.8 mmol) with Cs2CO3 (260.6 mg, 0.8 mmol) to afford 5c (79.7 mg, 20% × 2 = 40%) using 20% ethyl acetate/petether as a pale-yellow solid. Mp: 178–180 °C; 1H NMR (400 MHz, CDCl3) δ 7.80 (s, 1H), 7.50 (d, J = 8.1 Hz, 2H), 7.25 (d, J = 8.2 Hz, 2H), 3.96 (s, 2H), 3.81 (s, 3H), 2.91 (sept, J = 6.9 Hz, 1H), 1.25 (d, J = 6.9 Hz, 6H); 13C NMR (101 MHz, CDCl3) δ 167.8, 150.5, 142.6, 132.3, 130.1 (2C), 126.9 (2C), 126.87, 52.4, 37.1, 34.1, 23.9 (2C); HRMS (ESI) calculated for C28H35O4S2 [M + H]+: m/z 499.1957, found 499.1939.
Allyl disulfane 6c
Prepared according to GP2 using 3eb (323.2 mg, 0.8 mmol) with Cs2CO3 (260.6 mg, 0.8 mmol) to afford 6c (107.0 mg, 36%) using 30% ethyl acetate/petether as a colorless solid. Mp: 216–218 °C; 1H NMR (400 MHz, CDCl3) δ 7.94 (s, 1H), 7.75 (d, J = 8.3 Hz, 2H), 7.47 (d, J = 8.1 Hz, 2H), 7.29 (dd, J = 8.7, 2.3 Hz, 4H), 4.54 (s, 2H), 3.63 (s, 3H), 3.04–2.88 (m, 1H), 2.46 (s, 3H), 1.30 (d, J = 6.9 Hz, 6H); 13C NMR (101 MHz, CDCl3) δ 167.2, 151.1, 146.4, 144.7, 136.5, 131.3, 129.67 (2C), 129.66 (2C), 128.7 (2C), 126.9 (2C), 120.1, 55.4, 52.4, 34.1, 23.9 (2C), 21.7; HRMS (ESI) calculated for C21H24O2SNa [M + Na]+: m/z 395.1293, found 395.1293.
Diallyl disulfane 5a
Prepared according to GP2 using 3aa (278.4 mg, 0.8 mmol) with Cs2CO3 (260.6 mg, 0.8 mmol) to afford 5a (63.2 mg, 19% × 2 = 38%) using 20% ethyl acetate/petether as a colorless solid.
Allyl disulfane 6d
Prepared according to GP2 using 3aa (278.4 mg, 0.8 mmol) with Cs2CO3 (260.6 mg, 0.8 mmol) to afford 6d (83.5 mg, 33%) using 30% ethyl acetate/petether as a pale-yellow viscous liquid. 1H NMR (400 MHz, CDCl3) δ 7.94 (s, 1H), 7.84 (dd, J = 8.3, 1.2 Hz, 2H), 7.60 (t, J = 7.5 Hz, 1H), 7.51–7.45 (m, 4H), 7.40–7.34 (m, 3H), 4.49 (s, 2H), 3.58 (s, 3H); 13C NMR (101 MHz, CDCl3) δ 166.9, 146.4, 139.4, 133.8, 133.7, 129.8, 129.2 (2C), 129.1 (2C), 128.8 (2C), 128.5 (2C), 121.0, 55.2, 52.4; HRMS (ESI) calculated for C17H16O2S2Na [M + Na]+: m/z 339.0667, found 339.0665.
Diallyl disulfane 5d
Prepared according to GP2 using 3ba (341.6 mg, 0.8 mmol) with Cs2CO3 (260.6 mg, 0.8 mmol) to afford 5d (98.6 mg, 21.5% × 2 = 43%) using 20% ethyl acetate/petether as a pale-yellow gum. 1H NMR (400 MHz, CDCl3) δ 7.72 (s, 1H), 7.52 (d, J = 8.5 Hz, 2H), 7.37 (d, J = 8.3 Hz, 2H), 3.84 (s, 2H), 3.81 (s, 3H); 13C NMR (101 MHz, CDCl3) δ 167.3, 141.0, 133.7, 132.0 (2C), 131.2 (2C), 128.6, 123.7, 52.5, 36.6; HRMS (ESI) calculated for C22H21O4S2Br2 [M + H]+: m/z 570.9248, found 570.9259.
Allyl disulfane 6e
Prepared according to GP2 using 3ba (341.6 mg, 0.8 mmol) with Cs2CO3 (260.6 mg, 0.8 mmol) to afford 6e (126.3 mg, 40%) using 30% ethyl acetate/petether as a pale-yellow gum. 1H NMR (400 MHz, CDCl3) δ 7.87 (s, 1H), 7.85 (dd, J = 8.4, 1.2 Hz, 2H), 7.66–7.60 (m, 1H), 7.54–7.48 (m, 4H), 7.39 (d, J = 8.3 Hz, 2H), 4.43 (s, 2H), 3.57 (s, 3H); 13C NMR (101 MHz, CDCl3) δ 166.7, 145.2, 139.4, 134.0, 132.6, 132.2 (2C), 130.8 (2C), 129.2 (2C), 128.6 (2C), 124.4, 121.6, 55.2, 52.6; HRMS (ESI) calculated for C17H15O4S2BrNa [M + Na]+: m/z 416.9772, found 416.9776.
Diallyl disulfane 5e
Prepared according to GP2 using 3hb (341.6 mg, 0.8 mmol) with Cs2CO3 (260.6 mg, 0.8 mmol) to afford 5e (89.2 mg, 19.5% × 2 = 39%) using 20% ethyl acetate/petether as a pale-yellow viscous liquid. 1H NMR (400 MHz, CDCl3) δ 7.78 (s, 1H), 7.59 (dd, J = 8.0, 1.1 Hz, 1H), 7.45 (dd, J = 7.6, 1.3 Hz, 1H), 7.32 (td, J = 7.5, 0.8 Hz, 1H), 7.20 (td, J = 7.5, 1.3 Hz, 1H), 3.82 (s, 3H), 3.57 (s, 2H); 13C NMR (101 MHz, CDCl3) δ 167.0, 141.2, 135.4, 133.0, 130.6, 130.2, 129.8, 127.5, 124.5, 52.5, 35.9; HRMS (ESI) calculated for C22H20O4S2Br2Na [M + Na]+: m/z 592.9063, found 592.9055.
Allyl disulfane 6f
Prepared according to GP2 using 3hb (341.6 mg, 0.8 mmol) with Cs2CO3 (260.6 mg, 0.8 mmol) to afford 6f (112.1 mg, 34%) using 30% ethyl acetate/petether as a pale-yellow viscous liquid. 1H NMR (400 MHz, CDCl3) δ 7.94 (s, 1H), 7.70 (d, J = 8.3 Hz, 2H), 7.62 (dd, J = 7.7, 1.1 Hz, 1H), 7.56 (dd, J = 8.0, 1.1 Hz, 1H), 7.35 (td, J = 7.6, 1.6 Hz, 1H), 7.28 (d, J = 8.0 Hz, 2H), 7.22 (td, J = 7.6, 1.2 Hz, 1H), 4.35 (s, 2H), 3.64 (s, 3H), 2.43 (s, 3H); 13C NMR (101 MHz, CDCl3) δ 166.5, 145.1, 144.8, 136.4, 134.2, 133.0, 130.7, 130.2, 129.8 (2C), 128.5 (2C), 127.7, 124.2, 123.0, 55.0, 52.6, 21.7; HRMS (ESI) calculated for C18H17O2S2BrNa [M + Na]+: m/z 430.9751, found 430.9926.
Diallyl disulfane 5f
Prepared according to GP2 using 3ob (353.0 mg, 0.8 mmol) with Cs2CO3 (260.6 mg, 0.8 mmol) to afford 5f (108.4 mg, 23.0% × 2 = 46%) using 20% ethyl acetate/petether as a pale-yellow viscous liquid. 1H NMR (400 MHz, CDCl3) δ 7.77 (s, 1H), 7.58 (d, J = 8.0 Hz, 1H), 7.46 (d, J = 7.6 Hz, 1H), 7.31 (t, J = 7.6 Hz, 1H), 7.19 (t, J = 7.7 Hz, 1H), 4.28 (q, J = 7.1 Hz, 2H), 3.59 (s, 2H), 1.33 (t, J = 7.1 Hz, 3H); 13C NMR (101 MHz, CDCl3) δ 166.5, 140.9, 135.4, 132.9, 130.6, 130.2, 130.1, 127.4, 124.5, 61.5, 36.1, 14.4; HRMS (ESI) calculated for C24H24O4S2Br2Na [M + Na]+: m/z 620.9380, found 620.9373.
Allyl disulfane 6g
Prepared according to GP2 using 3ob (353.0 mg, 0.8 mmol) with Cs2CO3 (260.6 mg, 0.8 mmol) to afford 6g (101.3 mg, 30%) using 30% ethyl acetate/petether as a pale-yellow viscous liquid. 1H NMR (400 MHz, CDCl3) δ 7.94 (s, 1H), 7.71 (d, J = 8.3 Hz, 2H), 7.62 (dd, J = 7.7, 1.1 Hz, 1H), 7.56 (dd, J = 8.0, 1.1 Hz, 1H), 7.35 (td, J = 7.5, 0.9 Hz, 1H), 7.28 (d, J = 8.3 Hz, 2H), 7.22 (td, J = 7.6, 1.3 Hz, 1H), 4.35 (s, 2H), 4.10 (q, J = 7.1 Hz, 2H), 2.43 (s, 3H), 1.26 (t, J = 7.1 Hz, 3H); 13C NMR (101 MHz, CDCl3) δ 166.0, 144.78, 144.76, 136.5, 134.3, 132.9, 130.7, 130.2, 129.8 (2C), 128.5 (2C), 127.7, 124.2, 123.3, 61.8, 55.0, 21.7, 14.1; HRMS (ESI) calculated for C19H19O2S2BrNa [M + Na]+: m/z 445.0088, found 445.0082.
Diallyl disulfane 5g
Prepared according to GP2 using 3gb (313.6 mg, 0.8 mmol) with Cs2CO3 (260.6 mg, 0.8 mmol) to afford 5g (60.6 mg, 16% × 2 = 32%) as a pale-yellow solid. Mp: 192–194 °C; 1H NMR (400 MHz, CDCl3) δ 7.74 (s, 1H), 7.25–7.19 (m, 1H), 7.03 (d, J = 2.2 Hz, 2H), 6.85 (dd, J = 8.1, 1.9 Hz, 1H), 3.87 (s, 2H), 3.76 (s, 3H), 3.75 (s, 3H); 13C NMR (101 MHz, CDCl3) δ 167.6, 159.8, 142.5, 136.1, 129.8, 128.1, 122.1, 115.4, 114.6, 55.5, 52.4, 37.0; HRMS (ESI) calculated for C24H26O6S2Na [M + Na]+: m/z 497.1068, found 497.1069.
Allyl disulfane 6h
Prepared according to GP2 using 3gb (313.6 mg, 0.8 mmol) with Cs2CO3 (260.6 mg, 0.8 mmol) to afford 6h (74.8 mg, 26%) using 30% ethyl acetate/petether as a pale-yellow gum. 1H NMR (400 MHz, CDCl3) δ 7.91 (s, 1H), 7.72 (d, J = 8.2 Hz, 2H), 7.32–7.24 (m, 3H), 7.11 (s, 1H), 7.01 (d, J = 7.6 Hz, 1H), 6.92 (d, J = 8.0 Hz, 1H), 4.48 (s, 2H), 3.85 (s, 3H), 3.61 (s, 3H), 2.43 (s, 3H); 13C NMR (101 MHz, CDCl3) δ 167.0, 159.9, 146.3, 144.9, 136.5, 135.1, 129.84, 129.76 (2C), 128.7 (2C), 121.7, 121.4, 116.0, 114.1, 55.6, 55.4, 52.5, 21.7; HRMS (ESI) calculated for C19H20O5SNa [M + Na]+: m/z 383.0929, found 383.0937.
Diallyl disulfane 5h
Prepared according to GP2 using 3jb (330.0 mg, 0.8 mmol) with Cs2CO3 (260.6 mg, 0.8 mmol) to afford 5h (89.3 mg, 21.7% × 2 = 43%) using 20% ethyl acetate/petether as a yellow solid. Mp: 187–198 °C; 1H NMR (400 MHz, CDCl3) δ 8.02 (s, 1H), 7.95 (s, 1H), 7.79 (dd, J = 8.7, 5.3 Hz, 3H), 7.57 (dd, J = 8.5, 1.5 Hz, 1H), 7.49 (ddd, J = 8.0, 6.7, 1.4 Hz, 2H), 4.03 (s, 2H), 3.81 (s, 3H); 13C NMR (101 MHz, CDCl3) δ 167.6, 142.4, 133.4, 133.2, 132.3, 129.8, 128.7, 128.4, 128.1, 127.8, 127.2, 126.8, 126.7, 52.4, 37.2; HRMS (ESI) calculated for C30H26O4S2Na [M + Na]+: m/z 537.1165, found 537.1170.
Allyl disulfane 6i
Prepared according to GP2 using 3jb (330.0 mg, 0.8 mmol) with Cs2CO3 (260.6 mg, 0.8 mmol) to afford 6i (99.6 mg, 33%) using 30% ethyl acetate/petether as yellow solid. Mp: 195–197 °C; 1H NMR (400 MHz, CDCl3) δ 8.07 (s, 1H), 7.97 (s, 1H), 7.88–7.78 (m, 3H), 7.70 (d, J = 8.3 Hz, 2H), 7.55–7.47 (m, 3H), 7.18 (d, J = 8.0 Hz, 2H), 4.57 (s, 2H), 3.67 (s, 3H), 2.33 (s, 3H); 13C NMR (101 MHz, CDCl3) δ 167.2, 146.2, 144.9, 136.5, 133.6, 133.2, 131.3, 129.7 (2C), 129.6, 128.8, 128.7 (2C), 128.5, 127.8, 127.5, 126.8, 126.1, 121.4, 55.4, 52.6, 21.7; HRMS (ESI) calculated for C22H20O2S2Na [M + Na]+: m/z 403.0980, found 403.0983.
Diallyl disulfane 5a
Prepared according to GP2 using 3ad (318.8 mg, 0.8 mmol) with Cs2CO3 (260.6 mg, 0.8 mmol) to afford 5a (52.6 mg, 15.8% × 2 = 32%) using 20% ethyl acetate/petether as a colorless solid.
Allyl disulfane 6j
Prepared according to GP2 using 3ad (318.8 mg, 0.8 mmol) with Cs2CO3 (260.6 mg, 0.8 mmol) to afford 6j (110.6 mg, 38%) using 30% ethyl acetate/petether as a pale-yellow solid. Mp: 227–229 °C; 1H NMR (400 MHz, CDCl3) δ 8.38 (s, 1H), 7.97–7.87 (m, 4H), 7.79 (dd, J = 8.7, 1.8 Hz, 1H), 7.69–7.57 (m, 2H), 7.37 (dd, J = 7.4, 1.6 Hz, 2H), 7.27–7.22 (m, 3H), 4.57 (s, 2H), 3.45 (s, 3H); 13C NMR (101 MHz, CDCl3) δ 166.9, 146.3, 136.2, 135.4, 133.6, 132.1, 130.5, 129.6, 129.5, 129.4, 129.3, 129.0 (2C), 128.7 (2C), 128.0, 127.7, 123.2, 121.1, 55.1, 52.4; HRMS (ESI) calculated for C21H19O4S2 [M + H]+: m/z 367.1004, found 367.1005.
Diallyl disulfane 5i
Prepared according to GP2 using 3nb (300.8 mg, 0.8 mmol) with Cs2CO3 (260.6 mg, 0.8 mmol) to afford 5i (70.7 mg, 20% × 2 = 40%) using 20% ethyl acetate/petether as a pale-yellow liquid. 1H NMR (400 MHz, CDCl3) δ 7.81 (s, 1H), 7.50 (d, J = 6.9 Hz, 2H), 7.40–7.33 (m, 3H), 4.27 (q, J = 7.1 Hz, 2H), 3.89 (s, 2H), 1.32 (t, J = 7.1 Hz, 3H); 13C NMR (101 MHz, CDCl3) δ 167.1, 142.1, 134.9, 129.7, 129.1 (2C), 128.8, 128.3 (2C), 61.4, 36.8, 14.4; HRMS (ESI) calculated for C24H27O4S2 [M + H]+: m/z 443.1334, found 443.1351.
Allyl disulfane 6k
Prepared according to GP2 using 3nb (300.8 mg, 0.8 mmol) with Cs2CO3 (260.6 mg, 0.8 mmol) to afford 6k (96.5 mg, 35%) using 30% ethyl acetate/petether as a pale-yellow liquid. 1H NMR (400 MHz, CDCl3) δ 7.91 (s, 1H), 7.71 (d, J = 8.3 Hz, 2H), 7.50–7.43 (m, 2H), 7.39–7.33 (m, 3H), 7.26 (d, J = 8.0 Hz, 2H), 4.48 (s, 2H), 4.07 (q, J = 7.1 Hz, 2H), 2.41 (s, 3H), 1.24 (t, J = 7.1 Hz, 3H); 13C NMR (101 MHz, CDCl3) δ 166.7, 146.0, 144.8, 136.5, 133.9, 129.7 (2C), 129.6, 129.3 (2C), 128.8 (2C), 128.7 (2C), 121.6, 61.7, 55.2, 21.7, 14.2; HRMS (ESI) calculated for C19H20O2S2Na [M + Na]+: m/z 367.0980, found 367.0981.
Conclusions
We have successfully developed a convenient protocol for the synthesis of allyl thiosulfonates. A range of aryl/heteroaryl/aliphatic allyl bromides and sodium arylthiosulfonates were readily assembled to furnish allyl thiosulfonates. Cs2CO3 serves as an efficient reagent for the synthesis of diallyl disulfanes and allyl disulfanes in moderate to high yields under mild reaction conditions. The present methodologies are operationally simple, tolerate a broad range of functional groups and are also reliable in a gram scale reaction for the synthesis of thiosulfonates.
Conflicts of interest
There are no conflicts to declare.
Acknowledgements
We thank SERB-ECR (File No. ECR/2015/000053), New Delhi for financial assistance. Our special thanks to OUDST-PURSE Programme for generous funding. R. J. R. thanks to UGC for faculty position under Faculty Recharge Programme. M. W. and J. J. K. thanks to UGC and DST Inspire, New Delhi, respectively for their research fellowship. We also thank OUCFRD for NMR facility.
Notes and references
- Leading reviews:
(a) C. Ni, M. Hu and J. Hu, Chem. Rev., 2015, 115, 765 CrossRef CAS PubMed
;
(b) J. E. M. D. McReynolds, J. M. Dougherty and P. R. Hanson, Chem. Rev., 2004, 104, 2239 CrossRef
;
(c) Organosulfur Chemistry I & II, ed. P. C. B. Page, Springer, Berlin, 1999 Search PubMed
. - Review articles:
(a) C.-F. Lee, R. S. Basha and S. S. Badsara, Top. Curr. Chem., 2018, 376, 25 CrossRef
;
(b) K. L. Dunbar, D. H. Scharf, A. Litomska and C. Hertweck, Chem. Rev., 2017, 117, 5521 CrossRef CAS PubMed
;
(c) X.-H. Xu, K. Matsuzaki and N. Shibata, Chem. Rev., 2015, 115, 731 CrossRef CAS PubMed
;
(d) C.-F. Lee, Y.-C. Liu and S. S. Badsara, Chem.–Asian J., 2014, 9, 706 CrossRef CAS
;
(e) H. Liu and X. Jiang, Chem.–Asian J., 2013, 8, 2546 CrossRef CAS PubMed
. -
(a) P. K. Shyam and H.-Y. Jang, J. Org. Chem., 2017, 82, 1761 CrossRef CAS PubMed
;
(b) K. Kanemoto, Y. Sugimura, S. Shimizu, S. Yoshida and T. Hosoya, Chem. Commun., 2017, 53, 10640 RSC
;
(c) W. Wang, X. Peng, F. Wei, C.-H. Tung and Z. Xu, Angew. Chem., Int. Ed., 2016, 55, 649 CrossRef CAS PubMed
;
(d) S. Yoshida, Y. Sugimura, Y. Hazama, Y. Nishiyama, T. Yano, S. Shimizu and T. Hosoya, Chem. Commun., 2015, 51, 16613 RSC
;
(e) P. Mampuys, Y. Zhu, T. Vlaar, E. Ruijter, R. V. A. Orru and B. U. W. Maes, Angew. Chem., Int. Ed., 2014, 53, 12849 CrossRef CAS PubMed
. - Recent examples:
(a) Y. Yu, Y. Zhou, Z. Song and G. Liang, Org. Biomol. Chem., 2018, 16, 4958 RSC
;
(b) F. Xiao, D. Wang, S. Yuan, H. Huang and G.-J. Deng, RSC Adv., 2018, 8, 23319 RSC
;
(c) Y. Siddaraju and K. R. Prabhu, Org. Biomol. Chem., 2017, 15, 5191 RSC
;
(d) P. Singh, R. Bai, R. Choudhary, M. C. Sharma and S. S. Badsara, RSC Adv., 2017, 7, 30594 RSC
;
(e) J. Sun, D. Zhang-Negrerie and Y. Du, Adv. Synth. Catal., 2016, 358, 2035 CrossRef CAS
;
(f) S.-r. Guo, Y.-q. Yuan and J.-n. Xiang, Org. Lett., 2013, 15, 4654 CrossRef CAS PubMed
. -
(a) N. S. Zefirof, N. V. Zyk, E. K. Beloglazkina and A. G. Kutateladze, Sulfur Rep., 1993, 14, 223 CrossRef
;
(b) J. P. Weidner and S. S. Block, J. Med. Chem., 1964, 7, 671 CrossRef CAS PubMed
. - Synthesis of thiosulfonates from disulfides and thiols oxidation, see:
(a) P. K. Shyam, Y. K. Kim, C. Lee and H.-Y. Jang, Adv. Synth. Catal., 2016, 358, 56 CrossRef CAS
;
(b) P. Natarajan, Tetrahedron Lett., 2015, 56, 4131 CrossRef CAS
;
(c) T. X. T. Luu, T.-T. T. Nguyen, T. N. Le, J. Spanget-Larsen and F. Duus, J. Sulfur Chem., 2015, 36, 340 CrossRef CAS
;
(d) M. Kirihara, S. Naito, Y. Nishimura, Y. Ishizuka, T. Iwai, H. Takeuchi, T. Ogata, H. Hanai, Y. Kinoshita, M. Kishida, K. Yamazaki, T. Noguchi and S. Yamashoji, Tetrahedron, 2014, 70, 2464 CrossRef CAS
. - Recent reports for thiosulfonates from sulfinate salts, see:
(a) N. Taniguchi, J. Org. Chem., 2015, 80, 1764 CrossRef CAS PubMed
;
(b) N. Taniguchi, Eur. J. Org. Chem., 2014, 5691 CrossRef CAS
;
(c) G. Liang, M. Liu, J. Chen, J. Ding, W. Gao and H. Wu, Chin. J. Chem., 2012, 30, 1611 CrossRef CAS
;
(d) G. Liang, J. Chen, J. Chen, W. Li, J. Chen and H. Wu, Tetrahedron Lett., 2012, 53, 6768 CrossRef CAS
. - Recent examples using sulfonyl chlorides and hydrazines, see:
(a) Z. Peng, X. Zheng, Y. Zhang, D. An and W. Dong, Green Chem., 2018, 20, 1760 RSC
;
(b) Q. Chen, Y. Huang, X. Wang, J. Wu and G. Yu, Org. Biomol. Chem., 2018, 16, 1713 RSC
;
(c) G.-Y. Zhang, S.-S. Lv, A. Shoberu and J.-P. Zou, J. Org. Chem., 2017, 82, 9801 CrossRef CAS PubMed
;
(d) X. Zhao, T.-X. Liu and G. Zhang, Asian J. Org. Chem., 2017, 6, 677 CrossRef CAS
;
(e) Y. Zheng, F.-L. Qing, Y. Huang and X.-H. Xu, Adv. Synth. Catal., 2016, 358, 3377 Search PubMed
. -
(a) M. Musiejuk, J. Doroszuk and D. Witt, RSC Adv., 2018, 8, 9718 RSC
;
(b) M. Salvadl, B. Amgarten, S. Castillln, G. J. L. Bernardes and O. Boutureira, Org. Lett., 2015, 17, 2836 CrossRef PubMed
;
(c) D. P. Gamblin, P. Garnier, S. J. Ward, N. J. Oldham, A. J. Fairbanks and B. G. Davis, Org. Biomol. Chem., 2003, 1, 3642 RSC
;
(d) S. Takano, K. Hiroya and K. Ogasawara, Chem. Lett., 1983, 255 CrossRef CAS
;
(e) A. P. Kozikowskif, A. Ames and H. Wetter, J. Organomet. Chem., 1979, 164, C33 CrossRef
and also see ref. 3b.. -
(a) E. Block, Angew. Chem., Int. Ed., 1992, 31, 1135 CrossRef
;
(b) S. Wang and H. Kohn, J. Med. Chem., 1999, 42, 788 CrossRef CAS
;
(c) T. T. Conway, E. G. DeMaster, D. J. W. Goon, F. N. Shirota and H. T. Nagasawa, J. Med. Chem., 1999, 42, 4016 CrossRef CAS PubMed
;
(d) C. Wright and R. D. Moore, Am. J. Med., 1990, 88, 647 CrossRef CAS PubMed
;
(e) S. A. Caldarelli, M. Hamel, J.-F. Duckert, M. Ouattara, M. Calas, M. Maynadier, S. Wein, C. Périgaud, A. Pellet, H. J. Vial and S. Peyrottes, J. Med. Chem., 2012, 55, 4619 CrossRef CAS PubMed
. -
(a) M. Song, J.-S. Kim, L. Liu, M. Husain and A. Vázquez-Torres, Cell Rep., 2016, 14, 2901 CrossRef CAS PubMed
;
(b) T. Ilani, A. Alon, I. Grossman, B. Horowitz, E. Kartvelishvily, S. R. Cohen and D. Fass, Science, 2013, 341, 74 CrossRef CAS PubMed
;
(c) J. Alegre-Cebollada, P. Kosuri, J. A. Rivas-Pardo and J. M. Fernández, Nat. Chem., 2011, 3, 882 CrossRef CAS PubMed
;
(d) M. Góngora-Benítez, J. Tulla-Puche and F. Albericio, Chem. Rev., 2014, 114, 901 CrossRef PubMed
. -
(a) B. Mandal and B. Basu, RSC Adv., 2014, 4, 13854 RSC
and references herein;
(b) T. M. Postma and F. Albericio, Eur. J. Org. Chem., 2014, 3519 CrossRef CAS
. - Selected reviews on MBH reaction, see:
(a) K. Kaur and I. N. N. Namboothiri, Chimia, 2012, 66, 913 CrossRef CAS PubMed
;
(b) D. Basavaiah, B. S. Reddy and B. S. Badsara, Chem. Rev., 2010, 110, 5447 CrossRef CAS PubMed
;
(c) V. Declerck, J. Martinez and F. Lamaty, Chem. Rev., 2009, 109, 1 CrossRef CAS PubMed
;
(d) V. Singh and S. Batra, Tetrahedron, 2008, 64, 4511 CrossRef CAS
;
(e) D. Basavaiah, K. V. Rao and R. J. Reddy, Chem. Soc. Rev., 2007, 36, 1581 RSC
. - Synthesis of sodium thiosulfonates,
(a) R. Sato, T. Goto, Y. Takikawa and S. Takizawa, Synthesis, 1980, 615 CrossRef CAS
;
(b) A. J Fischmanna and L. Spiccia, Dalton Trans., 2011, 40, 12310 RSC
. -
(a) R. J. Reddy, Md. Waheed, T. Karthik and A. Shankar, New J. Chem., 2018, 42, 980 RSC
;
(b) R. J. Reddy, A. Shankar, M. Waheed and J. B. Nanubolu, Tetrahedron Lett., 2018, 59, 2014 CrossRef CAS
. - The regioselectivities of thiosulfonates based on the corresponding MBH allyl bromides (as a mixture of E and Z isomers), see: D. Basavaiah, K. R. Reddy and N. Kumaragurubaran, Nat. Protoc., 2007, 2, 2665 CrossRef CAS PubMed
. -
(a) M. J. Cha, Y. S. Song, E.-G. Han and K.-J. Lee, J. Heterocycl. Chem., 2008, 45, 235 CrossRef CAS
;
(b) M. J. Cha, Y. S. Song and K.-J. Lee, Bull. Korean Chem. Soc., 2006, 27, 1900 CrossRef CAS
. - X. Xiao, M. Feng and X. Jiang, Angew. Chem., Int. Ed., 2016, 55, 14121 CrossRef CAS PubMed
.
Footnotes |
† Electronic supplementary information (ESI) available: General experimental procedures, spectroscopic data and NMR spectra for the new compounds. See DOI: 10.1039/c8ra06938g |
‡ These authors contributed equally. |
|
This journal is © The Royal Society of Chemistry 2018 |
Click here to see how this site uses Cookies. View our privacy policy here.