DOI:
10.1039/C8RA07349J
(Paper)
RSC Adv., 2018,
8, 36167-36171
Efficient production of high-molecular-weight hyaluronic acid with a two-stage fermentation
Received
3rd September 2018
, Accepted 17th October 2018
First published on 24th October 2018
Abstract
In this paper, the production pattern of hyaluronic acid (HA) was revealed: the chain growth of HA mainly occurred in the first half of fermentation while the product accumulated throughout the fermentation period. Attempts were made to develop a two-stage fermentation process which provided high-level synthesis in both product titer and molecular weight. The pH was kept at 8.0 with a temperature of 31 °C in the first fermentation stage (0–10 h) to promote the growth of weight-average molecular weight (Mw), and the pH and temperature were maintained at 7.0 and 37 °C, respectively, in the following fermentation stage to facilitate HA accumulation. In addition, constant rates of aeration (1 vvm) and agitation (600 rpm) were adopted. The two-stage fermentation provided a balanced result in which a product titer of 4.75 g L−1 and a Mw of 2.36 × 106 Da were achieved under optimized conditions. The process introduces an effective way to produce HA considering the effect of segmented control strategy.
1 Introduction
As a common component of synovial fluid and extracellular matrices, hyaluronic acid (HA) is a natural polysaccharide with a linear high molar mass composed of alternating 1,4-linked units of 1,3-linked D-glucuronic acid and N-acetyl-glucosamine.1 HA is distributed in the extracellular matrix, the synovial fluid of joints and cartilage,2 and it is identified as a signaling molecule in cell motility, cell differentiation, cancer metastasis and wound healing.3 Due to a remarkable viscoelastic property, HA and its commercial crosslinked derivatives are key materials in joint lubrication.4 The immunoneutrality of HA contributes to its wide application in the production of biomaterials.5
Traditionally, HA is extracted from animal tissues such as the vitreous of eyes, umbilical cord and rooster combs. However, in order to avoid the risk of cross-species viral infection, HA from microbial sources is receiving increased attention in recent years.6 As a capsular biopolymer, HA is found in Lancefield group A and C Streptococci.7 HA deriving from different sources is chemically identical and polydisperse, and the molecular weight of HA ranges from 104 Da to 107 Da.8
Due to the functionality dependency of HA on molecular weight, the bacterial process presents the opportunity to provide high yield of HA with long chain by optimizing culture conditions. And medium composition, temperature, pH, aeration and agitation have been considered as influential factors in HA fermentation.6 Armstrong and Johns studied the effect of culture conditions on molecular weight property of HA, and the highest Mw was obtained at a high initial glucose concentration (40 g L−1) accompanied by moderate aeration (0.2 to 1 vvm).7 Vidhya et al. found that sucrose and lactose were better carbon sources than glucose for production of high molecular weight HA.9 Vázquez et al. reported the production of HA by Streptococcus zooepidemicus based on protein substrates deriving from Scyliorhinus canicula discards, and the highest HA titer reached 2.66 g L−1.10 Kim et al. found the optimized temperature (37 °C), pH (7.0), agitation speed (1200 rpm) and aeration rate (1.0 vvm) contributed to the increase of molecular weight.11 Johns et al. reported that high levels of agitation (600 rpm) and aeration (0.3 vvm) efficiently improved the HA titer.12 Liu et al. proposed a two-stage culture strategy in which S. zooepidemicus were cultured in a fed-batch mode with sucrose concentration maintained at 1.0 g L−1 during 0–8 h and then batch culture was performed during 8–20 h with an initial sucrose concentration of 15 g L−1. With such two-stage culture strategy, HA production increased significantly,13 and the developed culture model provided an alternative strategy for the fermentation processes. Furthermore, it has also been reported that a balanced flux of precursors towards HA biosynthesis was required to increase the molecular weight of HA.14,15 However, former studies focused on either HA yield or molecular weight, and the high-level synthesis in both product titer and molecular weight is rarely reported. In this study, we aimed to develop a two-stage fermentation process in which the culture conditions were segmentally controlled to achieve a balanced high result in product titer as well as molecular weight.
2 Methods
2.1 Materials, strains and culture media
BHI (Brain Heart Infusion), YE (yeast extract), tryptone and agar were purchased from Aobox Biotechnology Co., Ltd. (Beijing, China). All other chemicals used were of analytical grade and commercially available.
S. zooepidemicus HA-13-06 (screened by our research group and maintained at the Fermentation Engineering Technology Research Center of Hebei Province, Hebei University of Science and Technology, China) was used in all experiments.
The medium for agar slant contained 2 g L−1 glucose, 10 g L−1 BHI, 5 g L−1 YE, 5 g L−1 tryptone, 5 g L−1 NaCl and 1.8 g L−1 K2HPO4. The medium for inoculum preparation contained 20 g L−1 glucose, 10 g L−1 YE, 5 g L−1 tryptone, 0.4 g L−1 MgSO4·7H2O and 1.8 g L−1 K2HPO4. The fermentation medium used in a 10 L fermentor contained 70 g L−1 glucose, 10 g L−1 YE, 5 g L−1 tryptone, 0.4 g L−1 MgSO4·7H2O and 1.8 g L−1 K2HPO4. The pH of all culture media was adjusted to 7.2 before sterilization which was performed at 116 °C for 25 min.
2.2 Culture conditions
Stock cultures of strain HA-13-06 were maintained at 4 °C. Inoculum preparation was carried out in 500 ml conical flasks with a working volume of 100 ml, which were incubated at 37 °C and 250 rpm for 24 h on a rotary shaker. The seed culture was then inoculated into 7 L fermentation medium in a 10 L fermentor (SGB-10L, Changzhou Sungod Bio-technology & Engineering Equipment Co., Ltd., Jiangsu, China) with an inoculation rate of 5% (v/v). The temperature, aeration rate and agitation speed were 37 °C, 1.0 vvm and 600 rpm, respectively, and 4 mol L−1 NaOH was added as a neutralizer to keep the pH at 7.0.
2.3 Optimization of fermentation conditions
In the optimization of fermentation conditions, the effects of main influential factors (pH, temperature, aeration and agitation) on HA fermentation was studied. And different levels of pH (no control, 6.0, 6.5, 7.0, 7.5, 8.0, 8.5 and 9.0), temperature (31 °C, 34 °C, 37 °C and 40 °C), aeration rate (0, 0.25 vvm, 0.5 vvm, 1 vvm and 1.5 vvm) and agitation speed (300 rpm, 450 rpm, 600 rpm and 750 rpm) were adopted. Particularly, all experiments were conducted based on batch fermentation process, and other parameters were the same to the basic culture conditions in Section 2.2.
2.4 Analytical methods
The samples were drawn from bioreactor at regular intervals to determine the concentrations of biomass, substrate and HA. Glucose were measured by SBA-40C biosensor analyzer (Institute of Biology, Shandong Province Academy of Sciences, Shandong, China), and the fermentation broth was properly diluted before determination.16 The cell density was measured by a spectrophotometer (UV-1100, Beijing Eternal Cause Instrument Co., Ltd, China) at 700 nm, and the concentration of dry cell weight (DCW) was obtained according to the correlative relationship between absorbance and DCW concentration.17 The carbazole method was adopted to determine the concentration of HA, and the optical density was measured at 525 nm using D-glucuronic acid as a standard.18 Mw of HA was determined according to the viscosity method developed by Laurent.19
3 Results and discussion
3.1 Effects of aeration and agitation on HA fermentation
In order to investigate the effect of aeration on HA fermentation, different aeration rates were adopted as shown in Fig. 1. In aerobic fermentation, the aeration rate has a significant influence on dissolved oxygen level which furtherly affects microbial metabolism. Chong et al. reported that glucose uptake and growth rates increased under aerobic conditions, and the altered metabolism translated into a 50% increase in HA productivity and more than a doubling in molecular weight from 1.07 to 2.4 × 106 Da.20 In this study, as the growth of aeration rate, the DCW concentration first increased up to a maximum then decreased, and the tendency of HA and Mw were similar to that of DCW concentration (Fig. 1). The top values of DCW concentration, HA and Mw reached 9.22 g L−1, 4.71 g L−1 and 1.63 × 106 Da, respectively. Armstrong et al. found the aerobic fermentation contributed to the increase of HA titer and Mw comparing with anaerobic fermentation, and the final HA concentration increased by 20%.21 Johns et al. also reported the promoting effect of aerobic fermentation on HA titer and yield.12 As microbial metabolic efficiency is higher at high dissolved oxygen concentration than that in anaerobic condition, the DCW concentration, HA titer and Mw increased with the growth of aeration rate ranging from 0 vvm to 1 vvm. However, when aeration rate increased from 1.0 vvm to 1.5 vvm, the DCW concent-ration declined from 9.22 g L−1 to 8.94 g L−1, and HA titer and Mw decreased by 12.1% and 24.5%, respectively. It is speculated that the oxygen radical generation in the presence of a large excess of oxygen might have a negative impact on the metabolism of strain HA-13-06, and the degradation of HA polymer chain might further occur.22
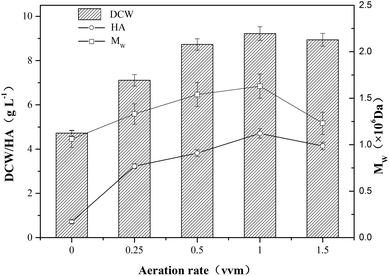 |
| Fig. 1 The fermentation of HA at different aeration rates ranging from 0 vvm to 1.5 vvm. The temperature and agitation speed were maintained at 37 °C and 600 rpm, respectively, and the pH was kept at 7.0 using 4 mol L−1 NaOH as a neutralizer. A 10 L fermentor was used, and the error bars in the figure indicate the standard deviations of three parallel replicates. | |
The influence of agitation on the yield and the molecular weight of HA is complex.23 The agitation speed is closely related to the transfer of mass and heat in the HA fermentation broth which is a non-newtonian fluid with high viscosity, and Johns et al. found agitation rate had a profound effect on both the titer and yield of HA produced in anaerobic fermentations.12 When the agitation speed rised from 300 rpm to 600 rpm, the DCW concentration increased from 6.51 g L−1 to 9.20 g L−1, however, the DCW concentration decreased 13.9% when the agitation speed further increased to 750 rpm. The tendency of HA titer and Mw were similar to that of DCW concentration (Fig. 2), and the top values of HA titer and Mw were 4.71 g L−1 and 1.63 × 106 Da, respectively. As shown in Fig. 2, DCW concentration, HA titer and Mw had a positive correlation with agitation speed ranging from 300 rpm to 600 rpm. However, the results indicated that the negative impact exceeded the positive impact of higher agitation speed (750 rpm) on HA production. The shear stress deriving from high agitation speed might damage HA polymer chain and microbial cell, and the oxygen radical generated by the excess of oxygen might cause the degradation of HA.24
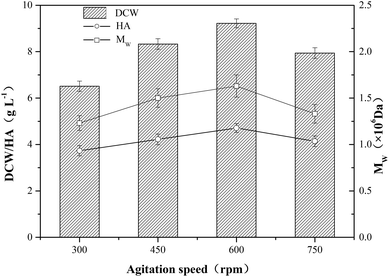 |
| Fig. 2 The fermentation of HA at different agitation speeds ranging from 300 rpm to 750 rpm. The temperature and aeration rate were maintained at 37 °C and 1.0 vvm, respectively, and the pH was kept at 7.0 using 4 mol L−1 NaOH as a neutralizer. A 10 L fermentor was used, and the error bars in the figure indicate the standard deviations of three parallel replicates. | |
3.2 Effect of pH on HA fermentation
In HA fermentation using S. zooepidemicus as producer, a sharp drop of pH was caused by the accumulation of carboxylic acid metabolites mainly composing of lactic acid, and the production of microbial biomass and HA was extremely inhibited by low pH, thus, the adjustment of pH provided a promoting effect on HA fermentation.25,26 As shown in Fig. 3, a significant increase in DCW concentration, HA titer and Mw was observed in experiments with pH control comparing with no-control condition.
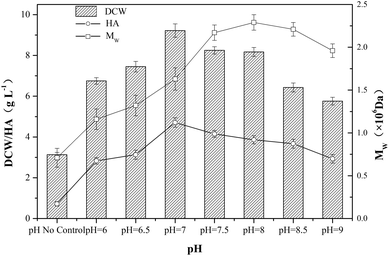 |
| Fig. 3 The fermentation of HA at different pH levels (no control, pH 6.0, 6.5, 7.0, 7.5, 8.0, 8.5 and 9.0). The temperature and aeration rate were maintained at 37 °C and 1.0 vvm, respectively, and the agitation speed was kept at 600 rpm. A 10 L fermentor was used, and the error bars in the figure indicate the standard deviations of three parallel replicates. | |
In order to investigate the effect of pH on HA fermentation, different pH levels ranging from 6.0 to 9.0 were adopted. With the increase of pH, both DCW concentration and HA titer first increased up to a maximum then decreased, and the top values of DCW concentration and HA titer reached 9.22 g L−1 and 4.71 g L−1, respectively, when the pH was kept at 7.0. In addition, the tendency of Mw was similar to that of DCW concentration and HA titer, and the highest Mw (2.29 × 106 Da) was obtained at pH 8.0. The results indicated that the maximum of HA titer and Mw could not be achieved at the same pH level.
3.3 Effect of temperature on HA fermentation
The catalytic activity of intracellular enzymes in microorganisms is mainly determined by temperature, and the change of temperature has a significant influence on microbial growth and product synthesis.27,28 As shown in Fig. 4, the Mw under different temperatures of 31 °C, 34 °C, 37 °C and 40 °C were 2.34 × 106 Da, 2.18 × 106 Da, 1.63 × 106 Da and 1.38 × 106 Da, respectively. The results indicated that low temperature contributed to the increase of Mw. However, when the temperature increased from 31 °C to 40 °C, both DCW concentration and HA titer first increased up to a maximum then decreased, and the top values of DCW concentration (9.22 g L−1) and HA titer (4.71 g L−1) were achieved at 37 °C. The maximum of HA titer and Mw could not be achieved at the same temperature, which was similar to the results obtained in pH optimization.
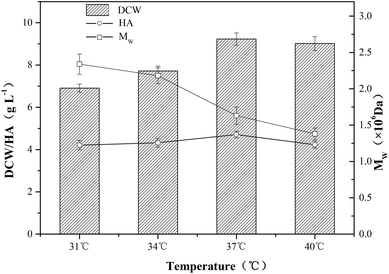 |
| Fig. 4 The fermentation of HA at different temperatures (31 °C, 34 °C, 37 °C and 40 °C). The agitation speed and aeration rate were maintained at 600 rpm and 1.0 vvm, respectively, and the pH was kept at 7.0 using 4 mol L−1 NaOH as a neutralizer. A 10 L fermentor was used, and the error bars in the figure indicate the standard deviations of three parallel replicates. | |
3.4 HA production in a two-stage fermentation
The results obtained in former experiments indicated the top values of HA titer and Mw could be obtained at the same level of aeration rate and agitation speed (1 vvm and 600 rpm). However, in respect to temperature and pH, the optimized condition for the HA accumulation was at 37 °C and pH 7.0, and the highest Mw could be obtained at 31 °C with a pH of 8.0. As shown in Fig. 5A, the glucose concentration continuously decreased from 70.22 g L−1 to 2.22 g L−1 within 24 h, and the final HA titer reached 4.71 g L−1 with a low Mw of 1.63 × 106 Da under the optimized condition for the HA accumulation (37 °C and pH 7.0). However, when the optimized condition (31 °C and pH 8.0) promoting Mw growth was adopted, a final Mw of 2.42 × 106 Da was achieved, and the HA titer was only 3.58 g L−1 (Fig. 5B), particularly, a residual glucose concentration was 5.13 g L−1 which was higher than that obtained under the optimized condition for the HA accumulation indicating a decreased product yield.
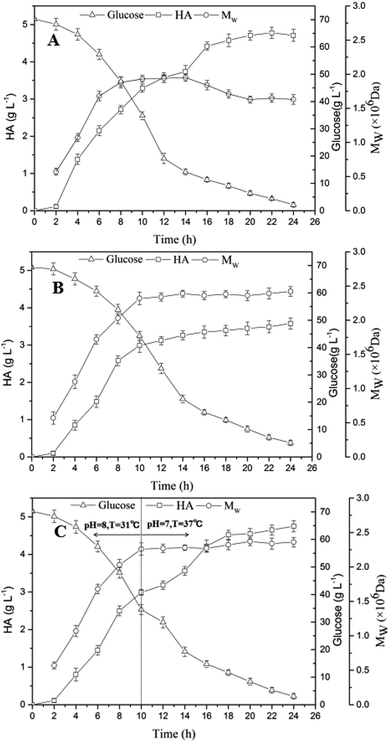 |
| Fig. 5 HA production under different conditions: (A) the optimized condition for the HA accumulation (37 °C and pH 7.0), (B) the optimized condition promoting Mw growth (31 °C and pH 8.0) and (C) segmented control containing condition (A) (10–24 h) and condition (B) (0–10 h). | |
By comparing Fig. 5A and B, the Mw mainly increased during the early stage of fermentation (0–10 h), however, the HA concentration accumulated continuously throughout the fermentation process. In order to obtain the maximum HA titer and highest Mw at the same time, a two-stage fermentation was further conducted. And it is worth to mention that the same aeration rate and agitation speed (1 vvm and 600 rpm) were maintained throughout the fermentation process. As shown in Fig. 5C, in the first stage of fermentation (0–10 h), the optimized condition for Mw (31 °C and pH 8.0) growth was adopted. Before 10 h, an accumulation of 2.99 g L−1 HA was obtained and the Mw reached 2.26 × 106 Da, while the glucose concentration decreased from 70.19 g L−1 to 34.54 g L−1. In the second stage (10–24 h) which was conducted under the condition (37 °C and pH 7.0) promoting HA accumulation, the HA concentration significantly increased from 2.99 g L−1 HA to 4.75 g L−1, and a slight increase of Mw (from 2.26 × 106 Da to 2.36 × 106 Da) was also observed, particularly, a relative low residual glucose concentration (3.09 g L−1) was obtained indicating the efficient conversion. Obviously, the segmented control strategy was effective to increase the efficiency of HA fermentation, and a balanced result containing high levels of both HA titer (4.75 g L−1) and Mw (2.36 × 106 Da) was eventually achieved.
4 Conclusions
An efficient way was introduced in this study to efficiently produce high-molecular-weight HA with a two-stage fermentation. The effect of main influential factors (pH, temperature, aeration and agitation) on HA fermentation was studied. The maximum of HA titer and Mw could be obtained at the condition of 1 vvm and 600 rpm, and the optimized condition for Mw was found to be 31 °C and pH 8.0, in addition, the top value of HA titer was obtained at 37 °C with a pH of 7.0. In the two-stage fermentation conducted with a segmented control strategy, a balanced result containing high levels of both HA titer and Mw was achieved, and the final HA concentration and Mw reached 4.75 g L−1 and 2.36 × 106 Da, respectively.
Conflicts of interest
There are no conflicts to declare.
Acknowledgements
We would like to acknowledge the establishment of the Fermentation Engineering Technology Research Center of Heibei Province. This work was supported by the Natural Science Foundation of Hebei Province (Grant No. C2016208052).
Notes and references
- G. Kogan, L. Šoltés, R. Stern and P. Gemeiner, Biotechnol. Lett., 2007, 29, 17 CrossRef CAS PubMed.
- T. C. Laurent, U. B. G. Laurent and J. R. E. Fraser, Ann. Rheum. Dis., 1995, 54, 429 CrossRef CAS PubMed.
- J. Entwistle, C. L. Hall and E. A. Turley, J. Cell. Biochem., 1996, 61, 569 CrossRef CAS PubMed.
- N. E. Larsen, E. Leshchiner and E. A. Balazs, Mater. Res. Soc. Symp. Proc., 1995, 394, 149 CrossRef CAS.
- L. E. Freed, G. Vunjak-Novakovic, R. J. Biron, D. B. Eagles, D. C. Lesnoy, S. K. Barlow and R. Langer, Biotechnology, 1994, 12, 689 CAS.
- W. C. Huang, S. J. Chen and T. L. Chen, Biochem. Eng. J., 2006, 32, 239 CrossRef CAS.
- D. C. Armstrong and M. R. Johns, Appl. Environ. Microbiol., 1997, 63, 2759 CAS.
- E. Shimada and G. Matsumura, J. Biochem., 1975, 78, 513 CrossRef CAS PubMed.
- R. Vidhya, S. Vyas, D. Jain, N. Vedapuri, V. Harshad and K. Arnab, EP2046969B1, 2009.
- J. A. Vázquez, L. Pastrana, C. Piñeiro, J. A. Teixeira, R. I. Pérez-Martín and I. R. Amado, Mar. Drugs, 2015, 13, 6537 CrossRef PubMed.
- J. H. Kim, S. J. Yoo, D. K. Oh, Y. G. Kweon, D. W. Park, C. H. Lee and G. H. Gil, Enzyme Microb. Technol., 1996, 19, 440 CrossRef CAS.
- M. R. Johns, L. T. Goh and A. OeggerU, Biotechnol. Lett., 1994, 16, 507 CrossRef CAS.
- L. Liu, G. Du, J. Chen, M. Wang and J. Sun, Bioresour. Technol., 2008, 99, 8532 CrossRef CAS PubMed.
- W. Y. Chen, E. Marcellin, J. A. Steen and L. K. Nielsen, Mol. Biotechnol., 2014, 56, 147 CrossRef CAS PubMed.
- M. V. Shah, S. S. Badle and K. B. Ramachandran, Biochem. Eng. J., 2013, 80, 53 CrossRef CAS.
- Y. Wang, D. Cai, M. He, Z. Wang, P. Qin and T. Tan, Bioresour. Technol., 2015, 198, 664 CrossRef CAS PubMed.
- I. R. Amado, J. A. Vázquez, L. Pastrana and J. A. Teixeira, Food Chem., 2016, 198, 54 CrossRef CAS PubMed.
- T. Bitter and H. M. Muir, Anal. Biochem., 1962, 4, 330 CrossRef CAS PubMed.
- T. C. Laurent, M. Ryan and A. Pietruszkiewicz, Biochim. Biophys. Acta, 1960, 42, 476 CrossRef CAS.
- B. F. Chong, L. M. Blank, R. Mclaughlin and L. K. Nielsen, Appl. Microbiol. Biotechnol., 2005, 66, 341 CrossRef CAS PubMed.
- D. C. Armstrong, M. J. Cooney and M. R. Johns, Appl. Microbiol. Biotechnol., 1997, 47, 309 CrossRef CAS.
- X. J. Duan, H. X. Niu, W. S. Tan and X. Zhang, J. Microbiol. Biotechnol., 2008, 19, 299 Search PubMed.
- C. G. Boeriu, J. Springer, F. K. Kooy, L. A. M. van den Broek and G. Eggink, Int. J. Carbohydr. Chem., 2013, 2013 Search PubMed.
- X. J. Duan, L. Yang and X. Zhang, J. Microbiol. Biotechnol., 2008, 18, 718 CAS.
- J. F. Lu, Y. Zhu, H. L. Sun, S. Liang, F. F. Leng and H. Y. Li, Lett. Appl. Microbiol., 2016, 62, 316 CrossRef CAS PubMed.
- L. Liu, Y. Liu, J. Li, G. Du and J. Chen, Microb. Cell Fact., 2011, 10, 99 CrossRef CAS PubMed.
- Y. Wang, C. Chen, D. Cai, Z. Wang, P. Qin and T. Tan, Bioresour. Technol., 2016, 218, 1098 CrossRef CAS PubMed.
- D. Vigetti, M. Viola, E. Karousou, G. De Luca and A. Passi, Matrix Biol., 2014, 35, 8 CrossRef CAS PubMed.
|
This journal is © The Royal Society of Chemistry 2018 |
Click here to see how this site uses Cookies. View our privacy policy here.