EMA-amplicon-based taxonomic characterisation of the viable bacterial community present in untreated and SODIS treated roof-harvested rainwater†
Received
4th September 2018
, Accepted 17th November 2018
First published on 22nd November 2018
Abstract
Illumina next generation sequencing coupled with the viability dye ethidium monoazide bromide (EMA) was utilised to characterise and compare the viable bacterial community present in roof-harvested rainwater pre- and post-treatment. The diversity and relative abundance of the viable bacterial community present in roof-harvested rainwater before (tank 1) and after solar disinfection treatment (SODIS-CPC-1) for eight h, was also determined. Taxonomic assignments were made using the Ribosomal Database Project whereafter alpha- and beta-diversity indices were calculated to investigate the effect of SODIS treatment on the viable bacterial population present in roof-harvested rainwater. Alpha-diversity indices, including species richness and Shannon diversity were significantly (p < 0.05) lower in the SODIS-CPC-1 treated rainwater samples in comparison to tank 1 rainwater samples, indicating a significant difference in the species richness after treatment. The tank 1 rainwater samples were dominated by the families Nocardiaceae (16.5%) and Pseudomonadaceae (8.9%), while the SODIS-CPC-1 rainwater samples were dominated by Nocardiaceae (44.0%) and Micrococcaceae (31.7%). On the genus level, Rhodococcus (17.1%) and Pseudomonas (9.2%) dominated in the tank 1 rainwater samples, while Rhodococcus (48.0%) and Arthrobacter (35.2%) were the most abundant in the SODIS-CPC-1 rainwater samples. While, viable opportunistic pathogenic bacteria such as Pseudomonas and Clostridium XI were detected in both the tank 1 and SODIS-CPC-1 rainwater samples, beta-diversity analysis, using the Bray-Curtis distance metric system, indicated that there was a significant shift (PERMANOVA, p < 0.05) in the viable bacterial community after SODIS treatment. Based on the results obtained, EMA-amplicon-based taxonomic analysis could be utilised as an alternative culture-independent method for the initial monitoring and profiling of the viable and viable-but-non-culturable whole bacterial community in water sources.
Water impact
Ethidium monoazide bromide (EMA)-amplicon-based taxonomic analysis can be used as a culture-independent technique for the initial monitoring of the viable whole bacterial community in water samples. A profile of the phylogenetic diversity before and after the application of water treatment methods is also obtained.
|
1. Introduction
Virulent Escherichia coli (E. coli), Legionella spp., Pseudomonas spp., Yersinia spp., adenovirus and Giardia spp., amongst others, have been detected in stored rainwater. As the presence of Legionella, Mycobacterium avium complex (MAC) and other pathogens and opportunistic pathogens in roof-harvested rainwater may pose a significant health risk to the end-users when used for potable and non-potable purposes, it is recommended that the water source be treated prior to utilisation.1–4 While various water treatment technologies such as chlorination, filtration, solar pasteurization (SOPAS) and solar disinfection (SODIS) have been employed to reduce the level of particularly microbial contamination in rainwater, SODIS is considered more cost-effective and consistently yields good treatment efficiencies. Solar disinfection is also promoted by the World Health Organisation (WHO) as a water treatment method and literature has indicated that the bactericidal effect of SODIS is based on the synergistic effect of direct ultraviolet (UV-A and UV-B) radiation and solar mild-heat to inactivate microorganisms.5 Various research groups have successfully employed SODIS strategies to reduce the level of particularly indicator microorganisms (such as E. coli and total coliforms) in harvested rainwater.6 However, viable opportunistic pathogens such as Legionella and Pseudomonas have been detected using ethidium monoazide bromide quantitative polymerase chain reaction (EMA-qPCR) analysis.7 Limited information is then available on the abundance and diversity of the overall bacterial population present in roof-harvested rainwater pre- and post-treatment.8
Next generation sequencing, such as Illumina sequencing of the 16S ribosomal ribonucleic acid (rRNA) gene, has been utilised to investigate and study complex, whole bacterial community structures in sewage, biosolids, urban water and rainwater.8,9 Information on the detailed community structure as well as the dominant bacterial taxa and the less common pathogens, which are generally present at low concentrations (<1% proportion of the sample), is obtained with a high taxonomic resolution.8,10 Chidamba and Korsten used pyrosequencing to sequence the V3 and V4 hypervariable region of the 16S rRNA gene in order to assess the diversity of the microbial community present in seven rainwater and two river water samples.9 Results showed that there were significant similarities in the community structure between rainwater samples, which differed from the community structure profiled for the river water samples. Furthermore, the authors detected low level signatures (few sequence reads, one in most cases) of potential pathogens (e.g. Pseudomonas, Clostridium, Yersinia and Legionella) in the rainwater. They hypothesised that the low level signature of potential pathogens could possibly be attributed to the low number of reads (10
956) which was obtained from the pyrosequencing platform.9
In a recent study conducted by Ahmed et al., Illumina next generation sequencing was utilised to characterise the overall bacterial community present in rainwater and analyse the distribution of genera in 88 rainwater tanks located in the Brisbane and Currumbin regions of Australia.8 The authors were able to sequence and analyse a high number of reads (2
795
320) which further enabled them to detect and identify the dominant opportunistic pathogens such as Clostridium, Mycobacterium, Legionella and Pseudomonas, amongst others, present in the rainwater. It should however, be noted that a limitation of both the Chidamba and Korsten9 and the Ahmed et al.8 studies is that no information on the viability of these species was obtained as Illumina next generation sequencing does not elucidate the viability status of the bacteria.
Previous studies have used qPCR assays in conjunction with nucleic acid binding dyes such as EMA and propidium monoazide (PMA), to quantify the viable portion of a specific species present in a sample.11,12 In a recent study conducted by Reyneke et al., various concentrations of EMA-, PMA- and DNase were compared and utilised in conjunction with qPCR assays for the determination of microbial cell viability.13 Based on the results obtained, it was concluded that EMA-qPCR assays (with an EMA concentration of 6 μM) were the most suitable for the identification of various opportunistic pathogens such as Legionella pneumophila, Pseudomonas aeruginosa (P. aeruginosa) and Salmonella typhimurium.13
A comprehensive literature search indicated that nucleic acid binding dyes have not previously been used in combination with next generation sequencing techniques such as Illumina sequencing. As both qPCR and Illumina next generation sequencing are amplicon-based screening assays, rainwater samples in the current study were EMA pre-treated prior to analysis by Illumina next generation sequencing in order to screen for the overall viable bacterial community present, pre- and post-treatment. As outlined in Strauss et al., a SODIS-compound parabolic collector (SODIS-CPC-1) was designed and connected to a rainwater harvesting tank (tank 1), for the treatment of roof-harvested rainwater. The results indicated that overall the E. coli counts were reduced to below the detection limit (1 CFU/100 mL) after SODIS treatment, while the heterotrophic bacteria and total coliform counts were significantly reduced by 2.60 and 3.17 logs, respectively. However, using EMA-qPCR, viable Legionella and Pseudomonas spp. were still detected in SODIS treated rainwater.7 The question therefore arose as to which other bacterial genera and species are persisting in rainwater following SODIS treatment. The primary aim of the current study was thus (i) to determine the diversity and abundance of the viable bacterial community present in the untreated rainwater (tank 1) and the SODIS treated (for eight h) rainwater (SODIS-CPC-1), using Illumina next generation sequencing coupled with EMA, (ii) to determine whether the viable bacterial community differs after SODIS treatment (untreated rainwater collected from tank 1 vs. SODIS-CPC-1) and lastly, (iii) to detect and identify the primary viable pathogenic and opportunistic pathogenic genera persisting after SODIS treatment in roof-harvested rainwater.
2. Materials and methods
2.1. SODIS-CPC system
Detailed information on the design, construction and installation of the SODIS-CPC-1 system and description of the sampling site at Welgevallen Experimental Farm, Stellenbosch University, South Africa (GPS co-ordinates: 33° 56′ 36.19′′S, 18° 52′ 6.08′′E) is outlined in Strauss et al.7
2.2. Sample collection
As outlined in Strauss et al., on the morning of a sampling event, rainwater was collected directly from the harvesting tank (tank 1) for analysis (sample representing untreated rainwater).7 Additionally, the 10.6 L reactor (borosilicate glass cylinder) of the SODIS-CPC-1 system was filled with harvested rainwater from tank 1. The rainwater in the SODIS-CPC-1 system was then exposed to direct sunlight for 8 h,7 whereafter the treated sample was collected from the SODIS-CPC-1 system. Samples were collected in 5 L sterile bottles and stored at 4 °C until they were processed (within 24 h). A total of seven sampling sessions were conducted from March to April 2017, resulting in 14 samples (n = 14) collected in total for the Strauss et al. study.7
Four control samples (EMA vs. non-EMA) were also included by combining 400 mL of each sample (n = 5) collected from tank 1 resulting in a 2 L composite tank 1 rainwater sample, while 400 mL of each sample (n = 5) collected from SODIS-CPC-1 was also combined resulting in a 2 L composite SODIS-CPC-1 rainwater sample. Each respective composite sample was then divided into two aliquots of 1 L. One litre of the composite tank 1 sample was not EMA-treated [control 1 – designated tank 1 (C)], while the remaining 1 L was EMA pre-treated resulting in control 2 [designated tank 1 (C-EMA)]. Similarly, 1 L of the composite SODIS-CPC-1 sample was not EMA-treated [control 3 – designated SODIS-CPC-1 (C)], while the remaining 1 L was EMA pre-treated resulting in control 4 [designated SODIS-CPC-1 (C-EMA)].
Results for the general rainwater quality [chemical (physicochemical, anions and cations) and microbial (indicator organism analyses and monitoring Legionella and Pseudomonas removal)] and system treatment efficiency were reported in Strauss et al.7 However, as the current follow-up study aimed to further elucidate which microbial genera remain viable following SODIS treatment using next generation sequencing coupled with EMA viability dye pre-treatment, only representative samples (n = 10) were used in the current analysis based on the maximum direct normal irradiance (DNI) (≥918.26 W m−2), ambient UV-A (≥19 W m−2) and UV-B (≥3.00 W m−2) radiation and the water temperature of the samples after SODIS (≥51 °C) as reported in Strauss et al.7 For the presentation of results in the figures, untreated samples for the sampling sessions one to five are designated as T1_1 (tank 1 sampling session one), T1_2 (tank 1 sampling session two), etc., while treated samples for the corresponding sampling sessions one to five are designated as S1_1 (SODIS-CPC-1 sampling session one), S1_2 (SODIS-CPC-1 sampling session two), etc. (Table S1†).
2.3. Rainwater concentration, EMA treatment, DNA extraction and quality control assessment
For each sampling session, 1 L of the untreated (tank 1) and treated (SODIS-CPC-1), as well as the four control samples were concentrated (flocculation and filtration). Briefly, 2 mL CaCl2 (1 M; Saarchem, Durban, South Africa) and 2 mL Na2HPO4 (1 M; Saarchem) was added to a 1 L water sample and the sample was stirred for 5 min to allow for flocculation, whereafter the sample was filtered through a 0.45 μm pore size non-charged mixed-ester membrane filter (Whatman GmbH, Dassel, Germany). Each filter was washed with 0.3 M citrate buffer and the resulting suspension of all the concentrated rainwater samples, with the exception of controls tank 1 (C) and SODIS-CPC-1 (C), were treated with 2.5 μg mL−1 (6 μM) EMA (Biotium, Hayward, USA). Following the addition of EMA to the concentrated rainwater sample, the sample was incubated on ice for 10 min followed by a 15 min halogen light exposure (keeping the sample on ice). The EMA treated sample was washed with 1 mL NaCl (0.85%; Saarchem) followed by centrifugation (16
000 × g for 5 min), whereafter deoxyribonucleic acid (DNA) was extracted using the Soil Microbe DNA MiniPrep™ Kit (Zymo Research, Irvine, USA) as per manufacturer's instructions.7
After DNA was extracted, quality control assessment was performed in order to determine the quality (purity), quantity and integrity of the 14 genomic DNA (gDNA) samples. The NanoDrop® ND-1000 (Nanodrop Technologies Inc., Wilmington, Delaware, USA) and Qubit™ fluorometer (Invitrogen Corporation, Carlsbad, CA, USA) (in conjunction with the Qubit™ dsDNA HS Assay Kit) were used to determine the UV absorbance ratios of 260 nm to 280 nm (A260/A280) and 230 nm (A260/A230) and the concentration of double-stranded DNA (dsDNA), respectively, of each sample in triplicate. In addition, the integrity of each sample was assessed by gel electrophoresis using a 1% agarose gel containing ethidium bromide (2% v/v) which was visualised using the Uvitec Alliance 2.7 chemiluminescent imager (Uvitec, Cambridge, UK).
2.4. 16S rRNA metagenomic sequencing
Deoxyribonucleic acid extracted from the 10 rainwater and 4 control samples were sent for 16S metagenomic sequencing library construction and sequencing at the Centre for Proteomic and Genomic Research (CPGR), Cape Town, South Africa, as previously described by Erol et al.14 Briefly, the sequencing library was prepared by amplifying a ∼464 bp region located in the hypervariable V3 and V4 region of the 16S rRNA prokaryotic gene using region of interest-specific primers with overhang Illumina adapter sequences.15 The forward primer 5′-TCGTCGGCAGCGTCAGATGTGTATAAGAGACAG![[C with combining low line]](https://www.rsc.org/images/entities/char_0043_0332.gif)
![[C with combining low line]](https://www.rsc.org/images/entities/char_0043_0332.gif)
![[T with combining low line]](https://www.rsc.org/images/entities/char_0054_0332.gif)
![[A with combining low line]](https://www.rsc.org/images/entities/char_0041_0332.gif)
![[C with combining low line]](https://www.rsc.org/images/entities/char_0043_0332.gif)
![[G with combining low line]](https://www.rsc.org/images/entities/char_0047_0332.gif)
![[G with combining low line]](https://www.rsc.org/images/entities/char_0047_0332.gif)
![[G with combining low line]](https://www.rsc.org/images/entities/char_0047_0332.gif)
![[N with combining low line]](https://www.rsc.org/images/entities/char_004e_0332.gif)
![[G with combining low line]](https://www.rsc.org/images/entities/char_0047_0332.gif)
![[G with combining low line]](https://www.rsc.org/images/entities/char_0047_0332.gif)
![[C with combining low line]](https://www.rsc.org/images/entities/char_0043_0332.gif)
![[W with combining low line]](https://www.rsc.org/images/entities/char_0057_0332.gif)
![[G with combining low line]](https://www.rsc.org/images/entities/char_0047_0332.gif)
![[C with combining low line]](https://www.rsc.org/images/entities/char_0043_0332.gif)
![[A with combining low line]](https://www.rsc.org/images/entities/char_0041_0332.gif)
-3′ and the reverse primer 5′-GTCTCGTGGGCTCGGAGATGTGTATAAGAGACAG![[G with combining low line]](https://www.rsc.org/images/entities/char_0047_0332.gif)
![[A with combining low line]](https://www.rsc.org/images/entities/char_0041_0332.gif)
![[C with combining low line]](https://www.rsc.org/images/entities/char_0043_0332.gif)
![[T with combining low line]](https://www.rsc.org/images/entities/char_0054_0332.gif)
![[A with combining low line]](https://www.rsc.org/images/entities/char_0041_0332.gif)
![[C with combining low line]](https://www.rsc.org/images/entities/char_0043_0332.gif)
![[H with combining low line]](https://www.rsc.org/images/entities/char_0048_0332.gif)
![[V with combining low line]](https://www.rsc.org/images/entities/char_0056_0332.gif)
![[G with combining low line]](https://www.rsc.org/images/entities/char_0047_0332.gif)
![[G with combining low line]](https://www.rsc.org/images/entities/char_0047_0332.gif)
![[G with combining low line]](https://www.rsc.org/images/entities/char_0047_0332.gif)
![[T with combining low line]](https://www.rsc.org/images/entities/char_0054_0332.gif)
![[A with combining low line]](https://www.rsc.org/images/entities/char_0041_0332.gif)
![[T with combining low line]](https://www.rsc.org/images/entities/char_0054_0332.gif)
![[C with combining low line]](https://www.rsc.org/images/entities/char_0043_0332.gif)
![[T with combining low line]](https://www.rsc.org/images/entities/char_0054_0332.gif)
![[A with combining low line]](https://www.rsc.org/images/entities/char_0041_0332.gif)
![[A with combining low line]](https://www.rsc.org/images/entities/char_0041_0332.gif)
![[T with combining low line]](https://www.rsc.org/images/entities/char_0054_0332.gif)
![[C with combining low line]](https://www.rsc.org/images/entities/char_0043_0332.gif)
-3′ were used where the underlined sequences refer to the 16S locus interest-specific sequence of the primer. The amplicons were purified from primer dimers and free primers using the AMPure XP clean-up kit (Beckman Coulter Genomics, Danvers, MA, USA) followed by an Index PCR using the Nextera XT Index kit (Illumina, San Diego, CA, USA) to attach dual indices and Illumina sequencing adapters to the original amplicon. A final amplicon purification step was performed using the AMPure XP clean-up kit (Beckman Coulter Genomics). Libraries were then normalised and pooled and sequenced on the MiSeq system using the MiSeq Reagent Kit v3 (Illumina).
2.5. Sequence data analysis
The open source bioinformatics program Mothur (version 1.39.5) was used to process the sequence reads,16 while the standard operating procedure described by Kozich et al. was followed with a few modifications (https://mothur.org/wiki/MiSeq_SOP).17 Contigs were created with the sequence paired-end reads and trimmed to 470 nucleotides. Sequences with homopolymers of 8 nucleotides or ambiguous bases were removed and high-quality sequences were aligned using the SILVA (v.128) reference database.18 A 1% pre-cluster error and chimera removal was conducted using VSEARCH in order to quality trim the sequences. Assignment of operational taxonomic units (OTUs) was completed at a 97% identity using the opticlust algorithm. Taxonomic assignments were made against the Ribosomal Database Project (version 16).19 The method described by Wang et al. was used for the classification (bootstrap cut-off of 80%).20 The sequences from individual samples were rarefied to equal sample size based on the sample with fewest sequences which equalled 116
122.
2.6. Statistical analysis
Mothur (version 1.39.5) was used to calculate sample coverage, which serves as an indication of the number of different species detected as a fraction of the cumulative number of species observed per study site. The total number of species detected with all methods combined per localisation are thus presented as a percentage.21 Furthermore, the number of OTUs observed was calculated as well as alpha (α)-diversity indices including species richness, the Shannon diversity index (indicates the abundance and evenness of the species based on the species present in the sample) and the abundance-based coverage estimate (ACE; statistical estimation of the total number of species present in a sample, based on the actual number of species detected). Differences in bacterial community composition [beta (β)-diversity] was evaluated through permutational multivariate analysis of variance (PERMANOVA) and visualised using non-metric multidimensional scaling (NMDS) which was performed using the computer software RStudio (version 1.0.153).22 This analysis provides information on the differences in composition of the viable bacterial populations between the untreated (tank 1) and treated (SODIS-CPC-1) rainwater samples. Furthermore, relative abundances on family and genus level was also calculated using RStudio and was visualised using the computer software Microsoft® Excel (version 15.39).
3. Results
3.1. Bacterial α-diversity analysis of untreated and SODIS treated rainwater samples
The four control samples were included in the Illumina next generation sequencing analysis to determine whether EMA treatment, prior to the sequencing analysis, reduced the species richness and the number of OTUs in the samples and was therefore able to reduce the detection of non-viable bacteria. The results for the control samples indicated that a mean sample coverage of 99.1% was obtained, indicating that sufficient sequence data was generated to obtain a nearly complete characterisation of the bacterial communities in the control samples (results not shown). Alpha diversity indices then demonstrated that the species richness in the rainwater tank 1 non-EMA treated sample was 1951, compared to 1723 recorded for the corresponding EMA-treated sample (results not shown). The recorded species richness then also decreased from 1205 for the SODIS-CPC-1 non-EMA treated sample to 1006 in the corresponding EMA-treated sample. The Shannon diversity index was the same for the non-EMA treated and EMA treated rainwater tank 1 control samples (3.1), while the diversity score in the non-EMA treated SODIS-CPC-1 sample (1.0) was comparable to the EMA-treated SODIS-CPC-1 sample (0.9) (results not shown). These results then confirmed that the signal from the non-viable bacterial community was reduced with the addition of EMA prior to sequencing analysis.
The amplicon sequencing analysis of the experimental untreated and SODIS treated rainwater samples yielded a total of 1
179
075 and 1
196
969 sequences which were further clustered into a total of 5874 and 4020 OTUs for the tank 1 (n = 5) and SODIS-CPC-1 (n = 5) rainwater samples at a similarity of 97%, respectively. Overall, the sequencing analysis resulted in a 99.1 ± 0.001% and 99.3 ± 0.001% mean sample coverage for the tank 1 and SODIS-CPC-1 rainwater samples. (Table 1).
Table 1 Mean and standard deviation of α-diversity indices, including percentage sample coverage, Shannon diversity index, species richness and ACE, observed for tank 1 (untreated) and SODIS-CPC-1 (treated) rainwater samples. The significance (p value) for the respective indices between tank 1 and SODIS-CPC-1 rainwater sample are also indicated
Sample |
% coverage |
Shannon |
Species richnessa |
ACE |
The number of different species observed.
|
Tank 1 |
99.1 ± 0.001 |
3.8 ± 0.9 |
1865.0 ± 129.4 |
4997.7 ± 445.7 |
SODIS-CPC-1 |
99.3 ± 0.001 |
1.0 ± 0.6 |
1014.4 ± 216.7 |
5091.9 ± 1668.5 |
p value |
>0.05 |
<0.05 |
<0.05 |
>0.05 |
Various α-diversity indices were further used to investigate the species diversity and abundance within each sample. For the mean species richness, a significant difference (p < 0.05) was observed for the tank 1 rainwater samples (1865.0 ± 129.4) versus the SODIS-CPC-1 rainwater samples (1014.4 ± 216.7) (Table 1). Similarly, a significant difference (p < 0.05) was observed in the mean Shannon diversity indices for the tank 1 rainwater samples (3.8 ± 0.9) versus the SODIS-CPC-1 rainwater samples (1.0 ± 0.6) (Table 1). The significantly higher mean species richness and Shannon diversity index recorded in the tank 1 rainwater samples, compared to the SODIS-CPC-1 rainwater samples, suggest a greater abundance and evenness of the viable bacterial community in the tank 1 rainwater samples.
In contrast, for the mean ACE, no significant difference (p > 0.05) was observed for the tank 1 rainwater samples (4997.7 ± 445.7) versus the SODIS-CPC-1 rainwater samples (5091.9 ± 1668.5) (Table 1). Rarefaction curves for the OTUs observed (species richness) for each sample collected from tank 1 and SODIS-CPC-1 are indicated in Fig. S1† and depicts the dispersion of the number of different OTUs observed per sample.
3.2. Taxonomic diversity and characterisation of bacterial families and genera in rainwater samples
In order to investigate the β-diversity, the whole community structure (based on OTUs), between the tank 1 and the SODIS-CPC-1 rainwater samples were compared. Taxonomic diversity analysis revealed that 312
233 and 660
200 of the sequences were classified into 133 and 77 unique families for the tank 1 and SODIS-CPC-1 rainwater samples, respectively. Based on the relative abundance, the overall viable bacterial population in the tank 1 rainwater samples was predominantly comprised of the families Nocardiaceae (16.5%) followed by Pseudomonadaceae (8.9%), Sphingomonadaceae (6.0%), Planctomycetaceae (4.2%), Chitinophagaceae (3.1%) and Oxalobacteraceae (2.9%) (Fig. 1; Table S2†). However, it should be noted that for the tank 1 rainwater samples, a number of OTUs (mean of 47.2%) could not be classified into any known bacterial family, nevertheless this number significantly decreased (p = 0.010) in the SODIS-CPC-1 rainwater samples as a mean of only 12.2% of the number of OTUs could not be classified. Based on the relative abundance observed for the SODIS-CPC-1 rainwater samples, the viable bacterial population for these samples was predominantly comprised of the families Nocardiaceae (44.0%) followed by Micrococcaceae (31.7%), Oxalobacteraceae (5.0%), Xanthomonadaceae (1.9%), Rhizobiaceae (1.2%) and Chitinophagaceae (0.9%) (Fig. 1; Table S2†).
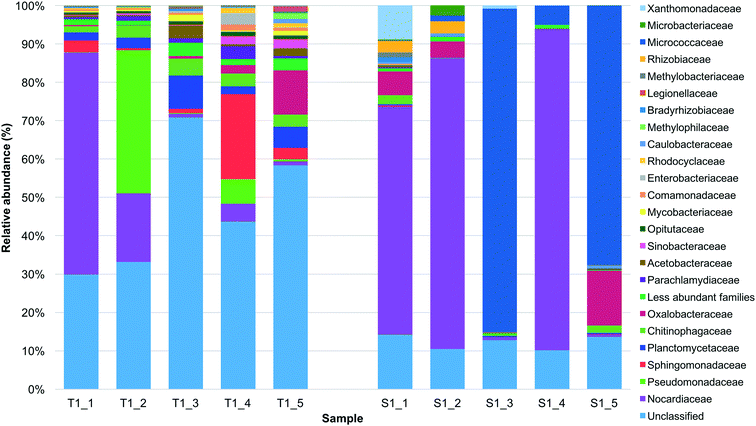 |
| Fig. 1 Relative abundance of the primary families detected in the before (tank 1: T1_1, T1_2, T1_3, T1_4 and T1_5) and after SODIS treated (SODIS-CPC-1: S1_1, S1_2, S1_3, S1_4 and S1_5) rainwater samples collected during the five sampling sessions. Sequences with a homology ≥80% were used. | |
On the genus level, 263
794 and 551
455 sequences were classified into 14 and 8 unique genera for the tank 1 and SODIS-CPC-1 rainwater samples, respectively. Based on the relative abundance for the genera detected, the overall viable bacterial population in the tank 1 rainwater samples was predominantly comprised of the genera Rhodococcus (17.1%) followed by Pseudomonas (9.2%), Sphingobium (4.6%), Undibacterium (2.5%), Sediminibacterium (1.4%) and Nevskia (1.1%). The overall viable bacterial population in the SODIS-CPC-1 rainwater samples were predominantly comprised of the genera Rhodococcus (48.0%) followed by Arthrobacter (35.2%), Oxalicibacterium (5.3%), Stenotrophomonas (1.9%), Kaistia (1.3%) and Sediminibacterium (1.0%). Additionally, 5.0% of the OTUs in the SODIS-CPC-1 rainwater samples could not be classified into any known genera, which was significantly lower (p = 0.0033) than the 54.6% unclassified OTUs for the tank 1 rainwater samples (Fig. 2; Table S3†).
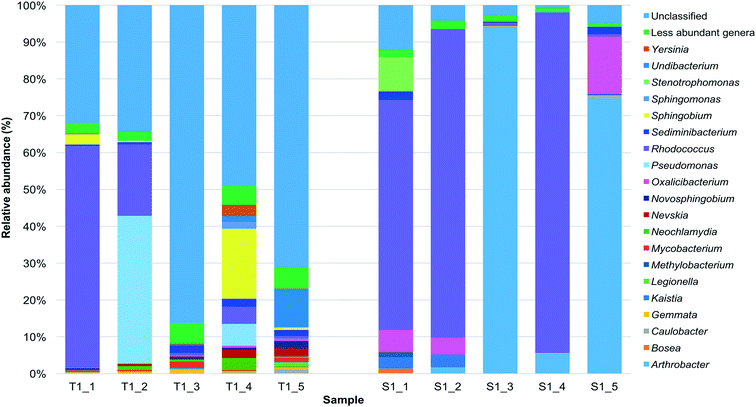 |
| Fig. 2 Relative abundance of the primary genera detected in the before (tank 1: T1_1, T1_2, T1_3, T1_4 and T1_5) and after SODIS treated (SODIS-CPC-1: S1_1, S1_2, S1_3, S1_4 and S1_5) rainwater samples collected during the five sampling sessions. Sequences with a homology ≥80% were used. | |
Further contributing to the higher species diversity (α-diversity indices) recorded in the tank 1 rainwater samples was the increased percentage of low abundance families (2.2%) and genera (4.3%) observed in these samples, in comparison to the low abundance families (0.7%) and genera (1.5%) recorded after SODIS treatment.
To subsequently investigate the β-diversity, which is the variation in species diversity between samples (tank 1 vs. SODIS-CPC-1), the Bray–Curtis distance metric was used whereafter PERMANOVA was performed. Subsequently, a significant difference (PERMANOVA; p < 0.05) was observed between the β-diversity composition of the untreated (tank 1) and SODIS treated (SODIS-CPC-1) rainwater samples as two distinct clusters were observed on the NMDS ordination plot (Fig. 3).
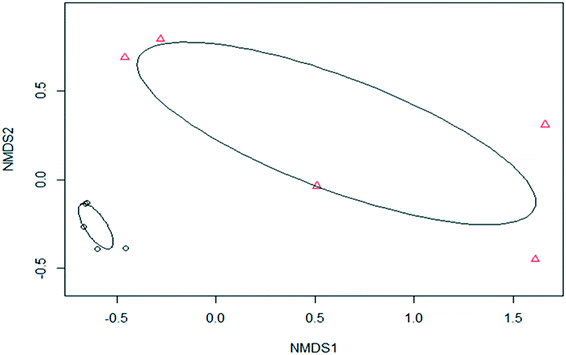 |
| Fig. 3 Non-metric multidimensional scaling ordination plot showing the relative differences in OTU community composition. The Bray–Curtis distance metric was used to quantify the similarity between the observed patterns. Ellipses indicate a 95% confidence interval for replicates. Two distinct clusters are observed (PERMANOVA, p < 0.05) where tank 1 rainwater samples are represented by red triangles, while SODIS-CPC-1 rainwater samples are represented by black circles. | |
3.3. Signatures of potential opportunistic pathogenic and pathogenic genera present in rainwater samples
In order to determine whether viable opportunistic pathogens and pathogenic genera were present in the tank 1 and SODIS-CPC-1 samples, taxonomic assignments were made using the SILVA and RDP databases. Based on the data analysis, 14 viable opportunistic pathogens and pathogenic genera were identified in either the untreated (tank 1) or the SODIS treated (SODIS-CPC-1) rainwater samples or in both sample groups (Table 2). All the tank 1 rainwater samples (100%) were positive for the presence of Legionella spp., Mycobacterium spp., Pseudomonas spp., Yersinia spp., Clostridium III and Clostridium sensu stricto. Similarly, Pseudomonas spp. and Clostridium sensu stricto were detected in all the SODIS-CPC-1 rainwater samples (100%; Table 2). After SODIS treatment, the frequency of detection for the remaining dominant genera which were present in all the tank 1 samples i.e. Clostridium III, Legionella spp., Mycobacterium spp. and Yersinia spp., however, decreased to 20%, 60%, 60% and 60%, respectively. In addition, Acinetobacter spp. and Aeromonas spp. were detected at 80% and 60% in both the tank 1 and SODIS-CPC-1 rainwater samples, respectively. In contrast, Clostridium XI (60%) and Brevibacterium spp. (20%) were detected in the tank 1 rainwater samples, however these genera were not detected after SODIS treatment in the SODIS-CPC-1 rainwater samples. Similarly, Staphylococcus spp. (40%), Campylobacter spp. (20%) and Burkholderia spp. (20%) were only present in the SODIS-CPC-1 samples. Corynebacterium spp. were also detected in 60% of the tank 1 water samples, while the frequency of detection decreased to 20% in the SODIS-CPC-1 rainwater samples.
Table 2 Percentage rainwater samples (tank1 and SODIS-CPC-1) that tested positive for opportunistic pathogens
Genera |
Tank 1 (n = 5) |
SODIS-CPC-1 (n = 5) |
ND – not detected.
|
Acinetobacter
|
80 |
80 |
Aeromonas
|
60 |
60 |
Brevibacterium
|
20 |
ND |
Burkholderia
|
ND |
20 |
Campylobacter
|
ND |
20 |
Clostridium III
|
100 |
20 |
Clostridium sensu stricto
|
100 |
100 |
Clostridium XI
|
60 |
ND |
Corynebacterium
|
60 |
20 |
Legionella
|
100 |
60 |
Mycobacterium
|
100 |
60 |
Pseudomonas
|
100 |
100 |
Staphylococcus
|
ND |
40 |
Yersinia
|
100 |
60 |
4. Discussion
Based on an extensive literature search, to date high throughput next generation sequencing has only been used twice to characterise the bacterial community present in roof-harvested rainwater.8,9 However, in the current study a viability dye, namely EMA, was utilised in conjunction with the Illumina next generation sequencing platform to monitor the total viable bacterial community shift in rainwater pre- and post-treatment. Overall, the sequencing analysis resulted in a 99.1 and 99.3% mean sample coverage for the tank 1 and SODIS-CPC-1 rainwater samples (Table 1), which indicates that an expansive analysis of the bacterial communities present in the samples was obtained.
In addition, the results obtained in the current study indicated a clear community shift (families and genera) in the viable bacterial population between the untreated and the SODIS-CPC treated rainwater samples (Fig. 1 and 2). For the tank 1 rainwater samples, Nocardiaceae, Pseudomonadaceae and Sphingomonadaceae were the dominant families identified, while the families Nocardiaceae and Micrococcaceae exhibited a high relative abundance in the SODIS-CPC-1 rainwater samples. The dominance of the Nocardiaceae family in both sample groups is however, not surprising as genera associated with this family inhabits a wide range of aquatic and terrestrial environments.23 In addition, they are saprophytes which live on decaying organic matter such as leaves, debris, etc.24 Moreover, while there are no overhanging tree branches over the catchment area, the sampling site is surrounded by trees and gravel roads run adjacent to the sampling site.7 Leaves, decaying organic matter and dust are thus present on the catchment area and in the gutters and could have washed into the rainwater harvesting tank during a rain event.
The prevalence of the family Nocardiaceae correlates to the taxonomic analysis of the genus classification, where Rhodococcus spp. was the dominant genus present in the tank 1 rainwater samples as well as in the SODIS-CPC-1 rainwater samples. In addition, it is well known from literature that Rhodococcus spp. are classified as extremophiles and are resistant to environmental threats such as UV and osmotic stress.25 This is possibly due to its large bacterial chromosome (9.7 Mb) and the presence of three large linear plasmids.26,27 This genus further possesses high-fidelity DNA repair systems which render them genetically stable.28
The second most abundant family in the untreated tank 1 rainwater samples was Pseudomonadaceae. The genus Pseudomonas, which falls under the Pseudomonadaceae family, was also the second most abundant genus in the tank 1 rainwater samples (9.2%). Ahmed et al. established a significant positive correlation between the presence of Pseudomonas and bird faeces and attributed the presence of this genus in untreated rainwater to bird faecal matter contamination.8 Research has also indicated that Pseudomonas are able to persist during unfavourable environmental conditions such as high temperature and UV radiation, the primary disinfection strategies employed in SODIS, through the overexpression of the sigma factor algT.29 Moreover, Pseudomonas spp. are able to form associations with protozoa as a survival mechanism, where they exist as intracellular parasites.30
In contrast, the second most abundant family in the SODIS-CPC-1 treated rainwater samples was recorded as Micrococcaceae (31.7%). The genus Arthrobacter, which forms part of the Micrococcaceae family, accounted for 35.2% of the overall viable bacterial genera in the SODIS-CPC-1 rainwater samples. Arthrobacter is commonly found in soil environments and Kuhlman et al. isolated various bacteria, such as Arthrobacter, Cellulomona, Curtobacterium and Geodermatophilus, from rock varnish in the Whipple mountains, USA and stated that these bacteria are radiation-resistant as they were resistant to UV-C exposure.31 This was corroborated by Osman et al. who reported that Arthrobacter spp. exhibited elevated resistance to UV radiation.32
It is well-established that the microbiological quality of harvested rainwater is compromised as various research groups have detected bacterial, viral and protozoan pathogens in rainwater using molecular analysis.2,3,33 However, in order to accurately assess the health risk associated with using treated rainwater for potable or domestic purposes the viability of the pathogens detected by molecular presence/absence methods needs to be confirmed. The SILVA and RDP [as recommended by Liu et al.34] taxonomic classification databases were thus used in the current study to identify possible signatures of viable disease causing organisms present at genus level in the tank 1 and SODIS-CPC treated rainwater samples. In total, 14 opportunistic pathogens and pathogenic genera were subsequently identified in the tank 1 and/or SODIS-CPC-1 rainwater samples. It is important to note that Staphylococcus spp. (40%), Campylobacter spp. (20%) and Burkholderia spp. (20%) were only present in the SODIS-CPC-1 samples and were not detected in the tank 1 samples. It is hypothesised that this is possibly because the relative abundance of these genera were too low and therefore a higher sequencing depth is required for their detection.
Pseudomonas and Clostridium sensu stricto were detected in all (100%) the untreated and SODIS treated rainwater samples. As previously indicated, Pseudomonas was one of the dominant genera detected in the tank 1 rainwater samples and while present in all the SODIS-CPC-1 samples, a low relative abundance of this genus was detected. A study conducted in Sweden by Bagge et al. investigated the prevalence of Clostridium,35 which is well-known to persist in the gut of warm-blooded mammals, and reported that C. butyricum [which falls under the Clostridium sensu stricto group36] was isolated from cattle faeces. A dairy farm is located directly adjacent to the sampling site used in the current study and it is hypothesised that the dispersion of cattle faeces due to anthropogenic activity (vehicles that drive past etc.) could have contributed to the presence of Clostridium on the catchment area, which then washed into the tank during a rain event. Previous studies have also indicated that Clostridium are resistant to treatment by chemicals, moist heat and UV radiation, through the production of α/β-type small, acid-soluble proteins such as DacB and Spm.37
In the current study, Acinetobacter spp. were also detected in 80% of the untreated and SODIS treated rainwater samples. In 2017, the World Health Organisation (WHO) published a global priority list of antibiotic resistant pathogens and Acinetobacter baumannii and P. aeruginosa were included in the critical priority 1 group which included multidrug-resistant (MDR) Gram-negative bacteria.38Acinetobacter baumannii and P. aeruginosa are known to cause infection, such as bacteraemia, endocarditis and urinary tract infections, in debilitated patients in hospital environments, however they are also widely distributed in soil and water environments.39 Hörtnagl et al. investigated the effect of UV radiation on the growth efficiency of various bacteria, including Acinetobacter, Sphingomonas and Acidovorax, amongst others. They showed that the growth efficiency of Acinetobacter lwoffii increased significantly during UV radiation and it was concluded that Acinetobacter spp. are highly tolerant to UV radiation.40 In addition, Zenoff et al. reported that Acinetobacter johnsonii are able to endure as well as rapidly recover after UV radiation.41
Globally, rainwater harvesting plays an integral role in sustainable water management and is predominantly utilised to supplement rapidly depleting water resources.42 The presence of viable opportunistic pathogens and pathogenic genera in rainwater samples is thus a matter of serious concern and has predominantly been attributed to various environmental factors (farming, anthropogenic activity, industries, etc.) as well as to the period of dry days preceding a rain event.3,8 Moreover, signatures of potential pathogenic genera such as Mycobacterium, Clostridium, Leptospira, Campylobacter, Acinetobacter, Aeromonas and Enterobacter have previously been detected in bird and bat faecal samples.2 It is thus hypothesised that the presence of these viable opportunistic pathogenic genera in the treated and SODIS-CPC treated rainwater in the current study may predominantly be ascribed to bird faecal matter [Pseudomonas2 and Campylobacter43]; soil and dust on the catchment area [Acinetobacter39 and Brevibacterium44]; while Clostridium and Mycobacterium have previously been associated with cattle faecal matter.35,45
5. Conclusions
Using EMA in conjunction with the Illumina next generation sequencing platform, it was shown that the treatment of roof-harvested rainwater by solar radiation (UV light) and solar mild-heat decreased the species diversity significantly after SODIS and resulted in a community shift in the viable bacterial population. The variations in community structure in the before and after SODIS treatment samples were further supported by results obtained from the Bray–Curtis distance metric analysis as two separate clusters were observed for the tank 1 and SODIS-CPC-1 rainwater samples. There was also a significant difference (PERMANOVA; p < 0.05) between the community structures, which subsequently indicates that there was a significant shift in the viable bacterial community after SODIS treatment. The results obtained in this preliminary study thus shows that EMA-linked next generation sequencing may be used to determine the abundance and diversity of dominant viable opportunistic and pathogenic bacteria in rainwater samples.
However, various viable opportunistic pathogens and pathogenic bacteria were identified in roof-harvested rainwater after eight h of SODIS treatment. These bacterial genera have also been detected in dirt, debris, dust, organic matter and bird faecal matter present on the rainwater catchment area. Based on the results obtained for the EMA-linked next generation sequencing, viability-qPCR could subsequently be utilised to quantitatively and specifically investigate the abundance of the dominant pathogenic genera in rainwater (pre- or post-treatment) and identify the primary bacterial species present. Once the absolute species numbers are determined, quantitative microbial risk assessment studies should be performed in order to determine the risk of the dominant bacteria detected to human health. An educated decision on the primary uses of the SODIS treated roof-harvested rainwater can then be formulated.
Funding
This project has received funding from the European Union's Horizon 2020 Research and Innovation Program under grant agreement no. 688928. The financial assistance of the National Research Foundation (NRF, 90320) of South Africa towards the student scholarship is hereby also acknowledged. Opinions expressed and conclusions arrived at, are those of the authors and are not necessarily to be attributed to the NRF.
Conflicts of interest
The authors have no conflicts of interest to declare.
Acknowledgements
The authors would like to thank Dr. Ferdinand Postma for assistance with the sequence data and statistical analysis.
References
- E. Sazakli, A. Alexopoulos and M. Leotsinidis, Rainwater harvesting, quality assessment and utilization in Kefalonia Island, Greece, Water Res., 2007, 41, 2039–2047 CrossRef CAS PubMed.
- W. Ahmed, F. Huygens, A. Goonetilleke and T. Gardner, Real-time PCR detection of pathogenic microorganisms in roof-harvested rainwater in Southeast Queensland, Australia, Appl. Environ. Microbiol., 2008, 74, 5490–5496 CrossRef CAS PubMed.
- P. H. Dobrowsky, M. De Kwaadsteniet, T. E. Cloete and W. Khan, Distribution of indigenous bacterial pathogens and potential pathogens associated with roof-harvested rainwater, Appl. Environ. Microbiol., 2014, 80, 2307–2316 CrossRef CAS PubMed.
- K. A. Hamilton, W. Ahmed, S. Toze and C. N. Haas, Human health risks for Legionella and Mycobacterium avium complex (MAC) from potable and non-potable uses of roof-harvested rainwater, Water Res., 2017, 119, 288–303 CrossRef CAS PubMed.
- K. G. McGuigan, R. M. Conroy, H. Mosler, M. Du Preez, E. Ubomba-Jaswa and P. Fernández-Ibáñez, Solar water disinfection (SODIS): a review from bench- top to roof-top, J. Hazard. Mater., 2012, 235, 29–46 CrossRef PubMed.
- R. Nalwanga, C. K. Muyanja, K. G. McGuigan and B. Quilty, A study of the bacteriological quality of roof-harvested rainwater and an evaluation of SODIS as a suitable treatment technology in rural Sub-Saharan Africa, J. Environ. Chem. Eng., 2018, 6, 3648–3655 CrossRef CAS.
- A. Strauss, T. Ndlovu, B. Reyneke, M. Waso and W. Khan, Compound parabolic collector solar disinfection system for the treatment of harvested rainwater, Environ. Sci.: Water Res. Technol., 2018, 4, 976–991 RSC.
- W. Ahmed, C. Staley, K. A. Hamilton, D. J. Beale, M. J. Sadowsky, S. Toze and C. N. Haas, Amplicon-based taxonomic characterization of bacteria in urban and peri-urban roof-harvested rainwater stored in tanks, Sci. Total Environ., 2017, 576, 326–334 CrossRef CAS PubMed.
- L. Chidamba and L. Korsten, Pyrosequencing analysis of roof-harvested rainwater and river water used for domestic purposes in Luthengele village in the Eastern Cape province of South Africa, Environ. Monit. Assess., 2015, 187, 41 CrossRef PubMed.
- C. Staley, T. J. Gould, P. Wang, J. Phillips, J. B. Cotner and M. J. Sadowsky, Species sorting and seasonal dynamics primarily shape bacterial communities in the upper Mississippi River, Sci. Total Environ., 2015, 505, 435–445 CrossRef CAS PubMed.
- M. Leifels, L. Jurzik, M. Wilhelm and I. A. Hamza, Use of ethidium monoazide and propidium monoazide to determine viral infectivity upon inactivation by heat, UV-exposure and chlorine, Int. J. Hyg. Environ. Health, 2015, 218, 686–693 CrossRef CAS PubMed.
- B. Reyneke, P. H. Dobrowsky, T. Ndlovu, S. Khan and W. Khan, EMA-qPCR to monitor the efficiency of a closed-coupled solar pasteurization system in reducing Legionella contamination of roof-harvested rainwater, Sci. Total Environ., 2016, 553, 662–670 CrossRef CAS PubMed.
- B. Reyneke, T. Ndlovu, S. Khan and W. Khan, Comparison of EMA-, PMA-and DNase qPCR for the determination of microbial cell viability, Appl. Microbiol. Biotechnol., 2017, 101, 7371–7383 CrossRef CAS PubMed.
- O. Erol, I. W. C. E. Arends and G. Muyzer, Discovery of putative halogenases in environmental samples using metagenomics, Curr. Biotechnol., 2017, 6, 17–25 CrossRef CAS.
- A. Klindworth, E. Pruesse, T. Schweer, J. Peplies, C. Quast, M. Horn and F. O. Glöckner, Evaluation of general 16S ribosomal RNA gene PCR primers for classical and next-generation sequencing-based diversity studies, Nucleic Acids Res., 2013, 41, e1, DOI:10.1093/nar/gks808.
- P. D. Schloss, S. L. Westcott, T. Ryabin, J. R. Hall, M. Hartmann, E. B. Hollister, R. A. Lesniewski, B. B. Oakley, D. H. Parks, C. J. Robinson and J. W. Sahl, Introducing mothur: open-source, platform-independent, community-supported software for describing and comparing microbial communities, Appl. Environ. Microbiol., 2009, 75, 7537–7541 CrossRef CAS PubMed.
- J. J. Kozich, S. L. Westcott, N. T. Baxter, S. K. Highlander and P. D. Schloss, Development of a dual-index sequencing strategy and curation pipeline for analyzing amplicon sequence data on the MiSeq Illumina sequencing platform, Appl. Environ. Microbiol., 2013, 79, 5112–5120 CrossRef CAS PubMed.
- E. Pruesse, C. Quast, K. Knittel, B. M. Fuchs, W. Ludwig, J. Peplies and F. O. Glöckner, SILVA: a comprehensive online resource for quality checked and aligned ribosomal RNA sequence data compatible with ARB, Nucleic Acids Res., 2007, 35, 7188–7196 CrossRef CAS PubMed.
- J. R. Cole, Q. Wang, E. Cardenas, J. Fish, B. Chai, R. J. Farris, A. S. Kulam-Syed-Mohideen, D. M. McGarrell, T. Marsh, G. M. Garrity and J. M. Tiedje, The Ribosomal Database Project: improved alignments and new tools for rRNA analysis, Nucleic Acids Res., 2008, 37, D141–D145 CrossRef PubMed.
- J. Wang, P. A. McLenachan, P. J. Biggs, L. H. Winder, B. I. Schoenfeld, V. V. Narayan, B. J. Phiri and P. J. Lockhart, Environmental bio-monitoring with high-throughput sequencing, Briefings Bioinf., 2013, 14, 575–588 CrossRef PubMed.
- C. Westphal, R. Bommarco, G. Carre, E. Lamborn, N. Morison, T. Petanidou, S. G. Pottis, S. P. M. Roberts, H. Szentgyorgyi, T. Tscheulin, B. E. Vaissiere, M. Woyciechowski, J. C. Biesmeijer, W. E. Kunin, J. Settele and I. Stefan-Dewenter, Measuring bee diversity in different european habitats and biogeographical regions, Ecol. Monogr., 2008, 78, 653–671 CrossRef.
-
J. Oksanen, F. G. Blanchet, R. Kindt, P. Legendre, P. R. Minchin, R. B. O'Hara, G. L. Simpson, P. Solymos, M. Henry, H. Stevens, E. Szoecs and H. Wagner, Vegan: Community Ecology Package. R package version 2.3–0, http://CRAN.R-project.org/package=vegan (accessed September 2017) Search PubMed.
- N. Niyomvong, W. Pathom-aree, A. Thamchaipenet and K. Duangmal, Actinomycetes from tropical limestone caves, Chiang Mai J. Sci., 2012, 39, 373–388 Search PubMed.
-
M. Goodfellow, The family Nocardiaceae in The Prokaryotes: Actinobacteria, ed. E. Rosenberg, E. F. DeLong, S. Lory, E. Stacebrandt and F. Thompson, Springer, UK, 4th edn, 2014, pp. 595–650 Search PubMed.
- S. B. Urbano, V. H. Albarracín, O. F. Ordoñez, M. E. Farías and H. M. Alvarez, Lipid storage in high-altitude Andean Lakes extremophiles and its mobilization under stress conditions in Rhodococcus sp. A5, a UV-resistant actinobacterium, Extremophiles, 2013, 17, 217–227 CrossRef PubMed.
- M. P. McLeod, R. L. Warren, W. W. Hsiao, N. Araki, M. Myhre, C. Fernandes, D. Miyazawa, W. Wong, A. L. Lillquist, D. Wang and M. Dosanjh, The complete genome of Rhodococcus sp. RHA1 provides insights into a catabolic powerhouse, Proc. Natl. Acad. Sci. U. S. A., 2006, 103, 15582–15587 CrossRef PubMed.
- R. Van Der Geize and L. Dijkhuizen, Harnessing the catabolic diversity of rhodococci for environmental and biotechnological applications, Curr. Opin. Microbiol., 2004, 7, 255–261 CrossRef CAS PubMed.
- S. C. Santos, D. S. Alviano, C. S. Alviano, F. R. Goulart, M. De Pádula, Á. C. Leitão, O. B. Martins, C. M. Ribeiro, M. Y. Sassaki, C. P. Matta and J. Bevilaqua, Comparative studies of phenotypic and genetic characteristics between two desulfurizing isolates of Rhodococcus erythropolis and the well-characterized R. erythropolis strain IGTS8, J. Ind. Microbiol. Biotechnol., 2007, 34, 423–431 CrossRef CAS PubMed.
- S. Srivastava, A. Yadav, K. Seem, S. Mishra, V. Chaudhary and C. S. Nautiyal, Effect of high temperature on Pseudomonas putida NBRI0987 biofilm formation and expression of stress sigma factor RpoS, Curr. Microbiol., 2008, 56, 453–457 CrossRef CAS PubMed.
- V. Thomas, G. McDonnell, S. P. Denyer and J. Y. Maillard, Free-living amoebae and their intracellular pathogenic microorganisms: risks for water quality, FEMS Microbiol. Rev., 2010, 34, 231–259 CrossRef CAS PubMed.
- K. R. Kuhlman, L. B. Allenbach, C. L. Ball, W. G. Fusco, M. T. La Duc, G. M. Kuhlman, R. C. Anderson, T. Stuecker, I. K. Erickson, J. Benardini and R. L. Crawford, Enumeration, isolation and characterization of ultraviolet (UV-C) resistant bacteria from rock varnish in the Whipple Mountains, California, Icarus, 2005, 174, 585–595 CrossRef CAS.
- S. Osman, Z. Peeters, M. T. La Duc, R. Mancinelli, P. Ehrenfreund and K. Venkateswaran, Effect of shadowing on survival of bacteria under conditions simulating the Martian atmosphere and UV radiation, Appl. Environ. Microbiol., 2008, 74, 959–970 CrossRef CAS PubMed.
-
M. Lombard, M. De Kwaadsteniet, S. Khan and W. Khan, Comparison of treatment methods for the removal of Adeno- and Rotavirus from rain- and river water sources, B.Sc. Hons. Thesis, University of Stellenbosch, 2013 Search PubMed.
- Z. Liu, T. Z. DeSantis, G. L. Andersen and R. Knight, Accurate taxonomy assignments from 16S rRNA sequences produced by highly parallel pyrosequencers, Nucleic Acids Res., 2008, 36, e120, DOI:10.1093/nar/gkn491.
- E. Bagge, M. Persson and K. E. Johansson, Diversity of spore-forming bacteria in cattle manure, slaughterhouse waste and samples from biogas plants, J. Appl. Microbiol., 2010, 109, 1549–1565 CAS.
- P. A. Lawson and F. A. Rainey, Proposal to restrict the genus Clostridium Prazmowski to Clostridium butyricum and related species, Int. J. Syst. Evol. Microbiol., 2016, 66, 1009–1016 CrossRef CAS PubMed.
- D. Paredes-Sabja, N. Sarker, B. Setlow, P. Setlow and M. R. Sarker, Roles of DacB and Spm proteins in Clostridium perfringens spore resistance to moist heat, chemicals, and UV radiation, Appl. Environ. Microbiol., 2008, 74, 3730–3738 CrossRef CAS PubMed.
- World Health Organization, Global priority list of antibiotic-resistant bacteria to guide research, discovery, and development of new antibiotics, http://www.who.int/medicines/publications/global-priority-list-antibiotic-resistant-bacteria/en/ (accessed November 2017).
- H. J. Doughari, P. A. Ndakidemi, I. S. Human and S. Benade, The ecology, biology and pathogenesis of Acinetobacter spp.: an overview, Microbes Environ., 2011, 26, 101–112 CrossRef PubMed.
- P. Hörtnagl, M. T. Pérez and R. Sommaruga, Contrasting effects of ultraviolet radiation on the growth efficiency of freshwater bacteria, Aquat. Ecol., 2011, 45, 125–136 CrossRef PubMed.
- V. F. Zenoff, F. Siñeriz and M. E. Farías, Diverse responses to UV-B radiation and repair mechanisms of bacteria isolated from high-altitude aquatic environments, Appl. Environ. Microbiol., 2006, 72, 7857–7863 CrossRef CAS PubMed.
- M. De Kwaadsteniet, P. H. Dobrowsky, A. Van Deventer, W. Khan and T. E. Cloete, Domestic rainwater harvesting: microbial and chemical water quality and point-of-use treatment systems, Water, Air, Soil Pollut., 2013, 224, 1629 CrossRef.
- N. Abdollahpour, B. Zendehbad, A. Alipour and J. Khayatzadeh, Wild-bird feces as a source of Campylobacter jejuni in children's playgrounds in Iran, Food Control, 2015, 50, 378–381 CrossRef.
-
J. Fischer, Habitat: Brevibacterium linens, http://bioweb.uwlax.edu/bio203/s2012/fischer_jaco/habitat.htm (accessed November 2017) Search PubMed.
- S. J. Wells, M. T. Collins, K. S. Faaberg, C. Wees, S. Tavornpanich, K. R. Petrini, J. E. Collins, N. Cernicchiaro and R. H. Whitlock, Evaluation of a rapid fecal PCR test for detection of Mycobacterium avium subsp. paratuberculosis in dairy cattle, Clin. Vaccine Immunol., 2006, 13, 1125–1130 CrossRef CAS PubMed.
Footnote |
† Electronic supplementary information (ESI) available: Results for the rarefaction curves of the observed OTUs (Fig. S1), nomenclature of the tank 1 and SODIC-CPC-1 samples as used to present results (Table S1) and relative abundance percentages of the primary families (Table S2) and genera (Table S3), detected before and after treatment. See DOI: 10.1039/c8ew00613j |
|
This journal is © The Royal Society of Chemistry 2019 |
Click here to see how this site uses Cookies. View our privacy policy here.