Visible-light induced decarboxylative C2-alkylation of benzothiazoles with carboxylic acids under metal-free conditions†
Received
5th October 2018
, Accepted 30th November 2018
First published on 30th November 2018
Abstract
An effective protocol of photoredox catalyzed C2-alkylation of benzothiazoles with aliphatic carboxylic acids was disclosed. In the presence of an acridinium salt as a photocatalyst and air as an oxidant, a wide range of secondary or tertiary aliphatic carboxylic acids were employed as alkylation reagents, providing the desired products in good to excellent yields under mild reaction conditions with a broad substrate scope.
Introduction
Benzothiazoles are widespread in various natural products from agriculture and pharmaceutical agents to materials sciences.1 Synthetic methods which enable the selective functionalization of the C–H bonds of benzothiazoles could greatly facilitate their applications in these areas. Recently, transition-metal-catalyzed direct introduction of secondary and tertiary alkyl groups into benzothiazoles offered a powerful tool for the synthesis of alkyl-substituted benzothiazoles.2 For example, Miura and co-workers reported an efficient alkylation protocol of benzothiazoles with N-tosylhydrazones bearing unactivated alkyl groups using nickel and cobalt as catalysts.2a In 2014, Zhao's group developed an Ag-catalyzed alkylation of benzothiazoles with carboxylic acids through a direct decarboxylative cross-coupling reaction.2b Nevertheless, most of the strategies were carried out by utilizing precious and/or toxic metal complexes as catalysts or oxidants, leading to the difficult purification of the desired products from residual metal catalysts. Furthermore, some groups also described examples of direct C–H bond functionalization via cross dehydrogenative coupling processes without the use of a transition metal catalyst, yet these reactions often rely on the additives in the system to promote the reactions, and are limited in the substrate scope to cyclic and benzyl frameworks (cycloalkanes and toluene derivatives).3 Therefore, the development of efficient methods for the straightforward synthesis of alkylated benzothiazoles with various functional groups under metal-free conditions remains highly desirable.
Carboxylic acids are abundant, non-toxic and renewable feedstocks in organic synthesis, and are regarded as convenient radical precursors.4 Moreover, radicals are produced upon decarboxylation, and the elimination of CO2, as a traceless by-product, does not have an impact on their reactivity. Decarboxylative radical generation dated back to the classical studies of Hunsdiecker, who found that aliphatic, unsaturated, and aromatic carboxylic acids could efficiently undergo radical halogenative decarboxylation in the presence of silver salts.5 Subsequently, radical decarboxylative functionalization was widely used in organic synthesis, but an excess amount of strong oxidant and UV-light irradiation were usually applied as radical initiation conditions.6 In the past few years, visible-light photoredox catalysis has emerged as a valuable platform for the design of a variety of radical reactions under mild reaction conditions and low-energy irradiation.7 Recently, the application of this strategy to radical decarboxylation reactions has gathered much attention, and remarkable achievements have already been demonstrated.8 Among these, several examples of the visible-light-induced decarboxylation of aliphatic acids have been reported for cross-coupling reactions to date.9 However, the reactions often involve the use of Ir- and Ru-complexes as photosensitizers and hypervalent iodine reagents as stoichiometric sacrificial oxidants in most of the cases. Furthermore, molecular oxygen (O2), as an inexpensive, available and environmentally friendly oxidant, has scarcely been investigated in the visible-light-induced decarboxylative cross-coupling reactions under metal-free conditions.
Herein, we wish to report an effective photoredox decarboxylative method for the direct C2-alkylation of benzothiazoles with readily available aliphatic carboxylic acids using 9-mesityl-10-methylacridinium perchlorate ([Acr+-Mes]ClO4) as the photocatalyst and air as the oxidant under blue LED irradiation at room temperature, providing the desired products in good to excellent yields with a broad substrate scope (Scheme 1).
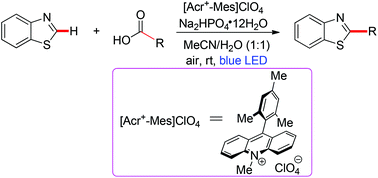 |
| Scheme 1 Decarboxylative C2-alkylation of benzothiazoles with carboxylic acids. | |
Results and discussion
Initially, a model reaction of benzothiazole (1a) and pivalic acid (2a) was chosen to optimize the reaction conditions, and the results are shown in Table 1. In the presence of 9-mesityl-10-methyl acridinium perchlorate ([Acr+-Mes]ClO4, 3 mol%) as a photocatalyst and Na2HPO4·12H2O as a base, this alkylation reaction could occur in water at room temperature by irradiation with a blue light emitting diode (LED, 410–415 nm, 1.5 W), generating the desired product 3a in 35% yield (Table 1, entry 1). By using CH3CN instead of H2O as a solvent, 58% yield of 3a was obtained (Table 1, entry 2). Much to our pleasure, an isolated yield of 83% was achieved when a mixture of acetonitrile and H2O (1
:
1 volume ratio) was used as the solvent (Table 1, entry 3). Other generally used photocatalysts, such as Ru(bpy)3Cl2, fac-Ir(ppy)3, eosin Y, acridine red or methylene blue was employed as the photocatalyst instead of [Acr+-Mes]ClO4 in the model reaction, but no desired product 3a was observed (Table 1, entries 4–9). The effect of solvent was also investigated in detail. The mixed solvent of H2O with DMSO (dimethyl sulfoxide), DMF (N,N-dimethylformamide), acetone, ethanol, THF (tetrahydrofuran) or 1,4-dioxane in the 1
:
1 volume ratio showed poor performance to the model reaction, providing product 3a in 24–55% yields (Table 1, entries 10–15). Further exploration of a variety of commercially available bases indicated that Na2HPO4·12H2O was superior to the others, including Na2CO3, K2CO3, Et3N and DIPEA (Table 1, entries 16–19). Inorganic bases Na2CO3 and K2CO3 were not suitable ones and the model reaction was prohibited completely. Organic bases Et3N and DIPEA gave inferior results and led to the formation of 3a in 56% and 39% yields, respectively. Furthermore, the role of atmospheric oxygen was also observed as an essential parameter in the formation of 3a because there was no reaction under the N2 atmosphere under the given conditions (Table 1, entries 20 and 21). Under the irradiation of a 450–455 nm LED, 68% yield of 3a was obtained (Table 1, entry 22). Similarly, the reaction also did not proceed under irradiation with a 1.5 W green LED (530 ± 5 nm) (Table 1, entry 23). It should be noted that no product was detected in the absence of a photocatalyst, visible light or a base (Table 1, entries 24–26). All these features demonstrate that this radical reaction is a photochemical process. In addition, the reactions were carried out in an open air tubed vessel in our experiments. The internal temperature of the reaction mixture was measured and found to be 30 ± 1 °C.
Table 1 Optimization of the reaction conditionsa
With the optimized reaction conditions in hand, a series of substituted benzothiazoles were examined to establish the substrate scope. As can be seen from Scheme 2, benzothiazoles substituted at the C6-position with electron-donating groups (Me, MeO and AcNH) provided the desired products 3b–3d in moderate to good yields. It is important to note that this reaction was compatible with electron-withdrawing substituents including Br and Cl on the aromatic rings, furnishing 76–90% yields of products 3e–3i, which can undergo further transformation for the synthesis of more complex molecules. Notably, benzothiazoles bearing a trifluoromethyl (CF3) group, which is a useful structural motif in biologically active molecules,10 reacted with 2a to generate the corresponding product 3j in 75% yield. Furthermore, 5-phenyl benzothiazole was also successful in this transformation and 70% yield of the corresponding product 3k was isolated.
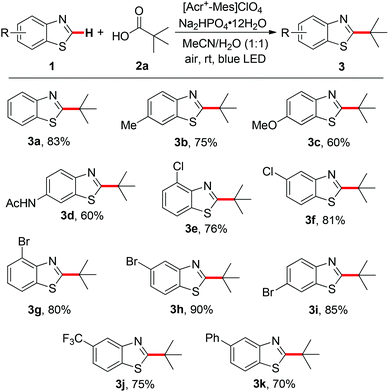 |
| Scheme 2 The scope of benzothiazoles [reaction conditions: benzothiazole (1, 0.5 mmol), pivalic acid (2a, 1.5 mmol), 9-mesityl-10-methyl acridinium perchlorate ([Acr+-Mes]ClO4, 3 mol%), Na2HPO4·12H2O (3.0 equiv.) and CH3CN/H2O (1 : 1, 3 mL) at room temperature under 1.5 W blue LED (410–415 nm) irradiation for 36 h in air; isolated yield of the product]. | |
Next, the scope of the readily available aliphatic carboxylic acids was investigated. The reaction of tertiary alkyl carboxylic acids proceeded smoothly with the construction of a quaternary carbon center to generate the corresponding products 3l–3v in good to excellent yields. It should be noted that adamantane-1-carboxylic acid is an excellent reaction partner, and 2-adamantan-1-yl-benzothiazoles (3o–3v) were obtained in 78–92% yields under the present reaction conditions. Due to the steric bulkiness of adamantane, radical chemistry is very convenient for its transformations and this moiety has been quintessential for the development of new drugs and catalysts.12 Furthermore, tolerance to a variety of functionalities such as Br, Cl and ether is particularly significant. In addition, secondary radicals also reacted well to form 3w–3ab in moderate to good yields. For example, secondary alkyl groups including 2-butyl, 2-pentyl, 3-pentyl, 2-hexyl and cyclohexyl were successfully incorporated into benzothiazoles, forming the desired products 3w–3ab. When a primary carboxylic acid, such as n-butyric acid was used in the reaction, no desired product (3ac) was isolated. These results demonstrate that the present photocatalytic system enables the selective generation of various alkyl radicals from the corresponding aliphatic carboxylic acids except for the primary carboxylic acid.
To clarify the reaction mechanism, 2,2,6,6-tetramethyl-1-piperidinyloxy (TEMPO), as a radical-trapping reagent, was added into the reaction system to capture the intermediate under the optimized conditions (Scheme 3). No desired product 3a was found upon the addition of TEMPO, whereas the tert-butyl radical of pivalic acid formed in situ was trapped by TEMPO to form a radical adduct (4), which was determined by HPLC-HRMS analysis (Scheme 4). The result implied that a radical process was involved in this transformation.
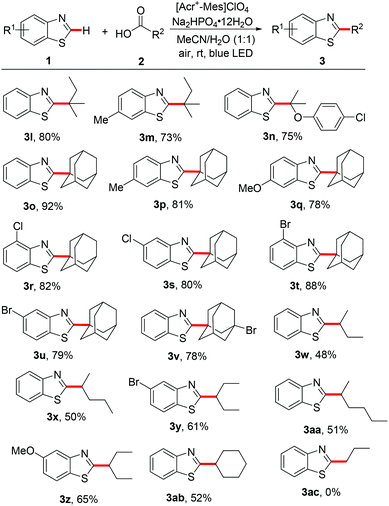 |
| Scheme 3 The scope of carboxylic acids [reaction conditions: benzothiazole (1, 0.5 mmol), carboxylic acid (2, 1.5 mmol), 9-mesityl-10-methyl acridinium perchlorate ([Acr+-Mes]ClO4, 3 mol%), Na2HPO4·12H2O (3.0 equiv.) and CH3CN/H2O (1 : 1, 3 mL) at room temperature under 1.5 W blue LED (410–415 nm) irradiation for 36 h in air; isolated yield of the product]. | |
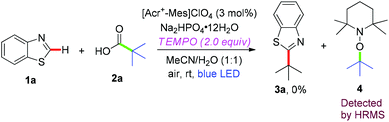 |
| Scheme 4 The radical-trapping experiment. | |
On the basis of the above results, fluorescence quenching experiments and previous reports,13 a plausible mechanism for this reaction is proposed in Scheme 5. Initially, a single electron oxidation of aliphatic carboxylic acid (2) by an excited-state [Acr+-Mes]* formed under blue LED irradiation and sequential decarboxylation occur to give the alkyl radical (A) and [Acr+-Mes]˙, which was then oxidized by O2 to complete the photocatalytic cycle along with the generation of O2˙−. Subsequently, the obtained A was added to the 2-position of the excited state 1a* which was from the reaction of 1a and [Acr+-Mes]* via energy transfer, providing intermediate B. B underwent an aromatization through an oxidation in the presence of O2˙− to generate the desired product 3via intermediate C. Notably, alkyl radical (A) was trapped by TEMPO to form a radical adduct (4), determined by HPLC-HRMS analysis. After the reaction was complete, the reaction solution was detected with a starch potassium iodide test paper and the test paper changed into blue colour, indicating that H2O2 was formed in the reaction.
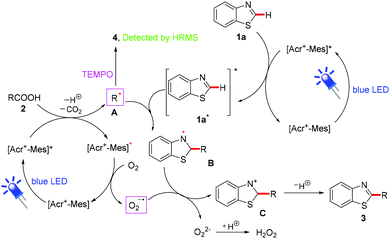 |
| Scheme 5 Proposed mechanism. | |
Conclusions
In summary, a novel and efficient photoredox induced decarboxylative C2-alkylation of benzothiazoles was developed by using a catalytic amount of 9-mesityl-10-methyl acridinium perchlorate (3 mol%) as a photocatalyst and air as an oxidant with the irradiation of a blue LED under transition-metal free conditions at room temperature. A variety of secondary and tertiary alkyl groups could be efficiently incorporated into benzothiazoles at the C2-position in good yields. The approach has advantages in terms of experimental simplicity, mild reaction conditions and easy work-up. Further expansion of this method to a broader spectrum of substrates is underway.
Experimental section
General remarks
All 1H NMR and 13C NMR spectra were recorded on a 400 MHz Bruker FT-NMR spectrometer (400 MHz or 100 MHz, respectively). All chemical shifts are given as δ values (ppm) with reference to tetramethylsilane (TMS) as an internal standard. The peak patterns are indicated as follows: s, singlet; d, doublet; t, triplet; m, multiplet; q, quartet. The coupling constants, J, are reported in hertz (Hz). High resolution mass spectroscopy data of the products were collected on an Agilent Technologies 6540 UHD Accurate-Mass Q-TOF LC/MS (ESI).
All reactions were set up in air. Methyl, bromo- and chloro-substituted benzothiazoles were prepared from the corresponding substituted 2-aminobenzothiazoles.11 All other chemicals were purchased commercially and used without further purification. Products were purified by flash chromatography on 200–300 mesh silica gels, SiO2. Melting points were determined on a digital melting point apparatus and temperatures were uncorrected.
Typical procedure for the photoredox induced decarboxylative C2-alkylation of benzothiazoles
A tubed vessel containing a stir bar was charged with benzothiazole (0.50 mmol), carboxylic acid (1.5 mmol), [Acr+-Mes]ClO4 (3 mol%), Na2HPO4·12H2O (1.5 mmol) and CH3CN/H2O (1
:
1, 3.0 mL). The reaction vessel was exposed to a blue LED (410–415 nm, 1.5 W) at room temperature for 36 h. Upon completion of the reaction, the mixture was diluted with water (3 mL) and extracted with ethyl acetate (10 mL × 3). The organic layer was combined, dried over anhydrous Na2SO4, and filtered. The solvent was removed via a rotary evaporator under reduced pressure and the residue was purified by flash chromatography (silica gel, a gradient eluent of petroleum ether/ethyl acetate) to yield the desired product.
Characterization data for the products
2-(tert-Butyl)benzo[d]thiazole (3a).
Yellow liquid (79.4 mg, 83%). 1H NMR (CDCl3, 400 MHz) δ: 7.99 (d, J = 8.2 Hz, 1H), 7.84 (d, J = 7.8 Hz, 1H), 7.43 (td, J = 7.7, 1.1 Hz, 1H), 7.32 (td, J = 7.6, 1.1 Hz, 1H), 1.52 (s, 9H); 13C NMR (CDCl3, 100 MHz) δ: 181.8, 153.3, 135.0, 125.7, 124.5, 122.7, 121.4, 38.3, 30.7. HRMS (ESI) [M + H]+ calcd for C11H14NS: 192.0841, found: 192.0841.
2-(tert-Butyl)-6-methylbenzo[d]thiazole (3b).
Yellow liquid (77.1 mg, 75%). 1H NMR (CDCl3, 400 MHz) δ: 7.86 (d, J = 8.3 Hz, 1H), 7.61 (s, 1H), 7.23 (dd, J = 8.3, 1.1 Hz, 1H), 2.45 (s, 3H), 1.50 (s, 9H); 13C NMR (CDCl3, 100 MHz) δ: 180.6, 151.3, 135.1, 134.4, 127.2, 122.1, 121.1, 38.2, 30.7, 21.4. HRMS (ESI) [M + H]+ calcd for C12H16NS: 206.0998, found: 206.0999.
2-(tert-Butyl)-6-methoxybenzo[d]thiazole (3c).
Yellow liquid (66.4 mg, 60%). 1H NMR (CDCl3, 400 MHz) δ: 7.86 (d, J = 8.9 Hz, 1H), 7.30 (d, J = 2.5 Hz, 1H), 7.03 (dd, J = 8.9, 2.5 Hz, 1H), 3.85 (s, 3H), 1.50 (s, 9H); 13C NMR (CDCl3, 100 MHz) δ: 179.2, 157.2, 147.7, 136.2, 123.1, 114.8, 104.2, 55.7, 38.1, 30.7. HRMS (ESI) [M + H]+ calcd for C12H16NOS: 222.0947, found: 222.0942.
N-(2-(tert-Butyl)benzo[d]thiazol-6-yl)acetamide (3d).
Yellow solid (74.4 mg, 60%). M.p. 114–115 °C. 1H NMR (CDCl3, 400 MHz) δ: 8.39 (d, J = 1.9 Hz, 1H), 8.37 (s, 1H), 7.83 (d, J = 8.7 Hz, 1H), 7.28 (dd, J = 8.7, 2.1 Hz, 1H), 2.18 (s, 3H), 1.49 (s, 9H); 13C NMR (CDCl3, 100 MHz) δ: 181.5, 168.8, 149.7, 135.7, 134.9, 122.3, 118.6, 112.6, 38.2, 30.6, 24.4. HRMS (ESI) [M + H]+ calcd for C13H17N2OS: 249.1056, found: 249.1056.
2-(tert-Butyl)-4-chlorobenzo[d]thiazole (3e).
Yellow liquid (85.5 mg, 76%). 1H NMR (CDCl3, 400 MHz) δ: 7.72 (d, J = 8.0 Hz, 1H), 7.44 (dd, J = 7.8, 0.6 Hz, 1H), 7.23 (t, J = 7.9 Hz, 1H), 1.53 (s, 9H); 13C NMR (CDCl3, 100 MHz) δ: 182.7, 150.2, 136.5, 127.5, 126.0, 125.0, 120.0, 38.6, 30.7. HRMS (ESI) [M + H]+ calcd for C11H13ClNS: 226.0452, found: 226.0451.
2-(tert-Butyl)-5-chlorobenzo[d]thiazole (3f).
Colorless oil (91.5 mg, 81%). 1H NMR (CDCl3, 400 MHz) δ: 7.97 (d, J = 2.0 Hz, 1H), 7.74 (d, J = 8.5 Hz, 1H), 7.30 (dd, J = 8.5, 2.0 Hz, 1H), 1.51 (s, 9H); 13C NMR (CDCl3, 100 MHz) δ: 183.9, 154.1, 133.2, 131.7, 125.0, 122.6, 122.1, 38.5, 30.6. HRMS (ESI) [M + H]+ calcd for C11H13ClNS: 226.0452, found: 226.0446.
4-Bromo-2-(tert-butyl)benzo[d]thiazole (3g).
Colorless oil (107.9 mg, 80%). 1H NMR (CDCl3, 400 MHz) δ: 7.76 (dd, J = 7.9, 0.9 Hz, 1H), 7.62 (dd, J = 7.8, 0.9 Hz, 1H), 7.16 (t, J = 7.9 Hz, 1H), 1.53 (s, 9H); 13C NMR (CDCl3, 100 MHz) δ: 182.3, 151.4, 136.0, 129.2, 125.3, 120.6, 116.3, 38.7, 30.7. HRMS (ESI) [M + H]+ calcd for C11H13BrNS: 269.9947, found: 269.9942.
5-Bromo-2-(tert-butyl)benzo[d]thiazole (3h).
White solid (121.2 mg, 90%). M.p. 88.5–90.0 °C. 1H NMR (CDCl3, 400 MHz) δ: 8.14 (d, J = 1.9 Hz, 1H), 7.69 (d, J = 8.5 Hz, 1H), 7.43 (dd, J = 8.5, 1.9 Hz, 1H), 1.51 (s, 9H); 13C NMR (CDCl3, 100 MHz) δ: 183.7, 154.5, 133.8, 127.6, 125.6, 122.5, 119.2, 38.5, 30.6. HRMS (ESI) [M + H]+ calcd for C11H13BrNS: 269.9947, found 269.9942.
6-Bromo-2-(tert-butyl)benzo[d]thiazole (3i).
Colorless oil (114.4 mg, 85%). 1H NMR (CDCl3, 400 MHz) δ: 7.95 (d, J = 1.7 Hz, 1H), 7.82 (d, J = 8.7 Hz, 1H), 7.52 (dd, J = 8.7, 1.9 Hz, 1H), 1.50 (s, 9H); 13C NMR (CDCl3, 100 MHz) δ: 182.3, 152.1, 136.6, 129.1, 123.9, 123.7, 117.9, 38.3, 30.6. HRMS (ESI) [M + H]+ calcd for C11H13BrNS: 269.9947, found: 269.9947.
2-(tert-Butyl)-5-(trifluoromethyl)benzo[d]thiazole (3j).
Colorless oil (97.1 mg, 75%). 1H NMR (CDCl3, 400 MHz) δ: 8.27 (s, 1H), 7.94 (d, J = 8.4 Hz, 1H), 7.57 (dd, J = 8.4, 1.0 Hz, 1H), 1.53 (s, 9H); 13C NMR (CDCl3, 100 MHz) δ: 184.1, 152.9, 138.5, 128.4 (q, J = 32.3 Hz), 124.3 (q, J = 270.5 Hz), 122.0, 120.9 (q, J = 3.5 Hz), 119.9 (q, J = 4.1 Hz), 38.6, 30.6. HRMS (ESI) [M + H]+ calcd for C12H13F3NS: 260.0715, found: 260.0710.
2-(tert-Butyl)-5-phenylbenzo[d]thiazole (3k).
Yellow liquid (93.8 mg, 70%). 1H NMR (CDCl3, 400 MHz) δ: 8.23 (d, J = 0.8 Hz, 1H), 7.85 (d, J = 8.3 Hz, 1H), 7.65 (d, J = 7.4 Hz, 2H), 7.56 (dd, J = 8.3, 1.6 Hz, 1H), 7.44 (t, J = 7.6 Hz, 2H), 7.34 (t, J = 7.3 Hz, 1H), 1.53 (s, 9H); 13C NMR (CDCl3, 100 MHz) δ: 182.6, 154.0, 140.9, 139.3, 133.9, 128.8, 127.3, 123.9, 121.5, 121.0, 38.4, 30.7. HRMS (ESI) [M + H]+ calcd for C17H18NS: 268.1154, found: 268.1139.
2-(tert-Pentyl)benzo[d]thiazole (3l).
Yellow liquid (82.1 mg, 80%). 1H NMR (CDCl3, 400 MHz) δ: 8.00 (d, J = 8.1 Hz, 1H), 7.85 (d, J = 7.9 Hz, 1H), 7.44 (td, J = 7.7, 1.1 Hz, 1H), 7.33 (td, J = 7.6, 1.0 Hz, 1H), 1.89–1.83 (m, 2H), 1.48 (s, 6H), 0.86 (t, J = 7.5 Hz, 3H); 13C NMR (CDCl3, 100 MHz) δ: 181.2, 153.2, 135.0, 125.6, 124.4, 122.7, 121.4, 41.7, 36.7, 28.0, 9.0. HRMS (ESI) [M + H]+ calcd for C12H16NS: 206.0998, found: 206.0999.
6-Methyl-2-(tert-pentyl)benzo[d]thiazole (3m).
Yellow liquid (80.2 mg, 73%). 1H NMR (CDCl3, 400 MHz) δ: 7.87 (d, J = 8.3 Hz, 1H), 7.62 (s, 1H), 7.23 (d, J = 8.5 Hz, 1H), 2.45 (s, 3H), 1.86–1.81 (m, 2H), 1.46 (s, 6H), 0.85 (t, J = 7.4 Hz, 3H); 13C NMR (CDCl3, 100 MHz) δ: 180.0, 151.3, 135.1, 134.4, 127.1, 122.1, 121.1, 41.6, 36.7, 28.0, 21.4, 9.0. HRMS (ESI) [M + H]+ calcd for C13H18NS: 220.1154, found: 220.1147.
2-(2-(4-Chlorophenoxy)propan-2-yl)benzo[d]thiazole (3n).
Yellow liquid (113.5 mg, 75%). 1H NMR (CDCl3, 400 MHz) δ: 8.03 (d, J = 8.2 Hz, 1H), 7.88 (d, J = 7.9 Hz, 1H), 7.49 (td, J = 7.7, 1.0 Hz, 1H), 7.39 (td, J = 7.6, 0.8 Hz, 1H), 7.14 (d, J = 8.9 Hz, 2H), 6.79 (d, J = 8.9 Hz, 2H), 1.85 (s, 6H); 13C NMR (CDCl3, 100 MHz) δ: 177.5, 153.5, 152.9, 135.7, 129.1, 128.1, 126.0, 125.2, 123.3, 122.4, 121.8, 81.5, 28.3. HRMS (ESI) [M + H]+ calcd for C16H15ClNOS: 304.0559, found: 304.0557.
2-(Adamantan-1-yl)benzo[d]thiazole (3o).
Yellow solid (123.5 mg, 92%). M.p. 77.1–77.8 °C. 1H NMR (CDCl3, 400 MHz) δ: 7.99 (d, J = 8.1 Hz, 1H), 7.85 (d, J = 7.8 Hz, 1H), 7.43 (td, J = 7.7, 1.2 Hz, 1H), 7.32 (td, J = 7.6, 1.0 Hz, 1H), 2.15 (s, 9H), 1.82 (s, 6H); 13C NMR (CDCl3, 100 MHz) δ: 182.1, 153.2, 134.4, 125.6, 124.4, 122.6, 121.5, 43.0, 40.2, 36.5, 28.6; HRMS (ESI) [M + H]+ calcd for C17H20NS: 270.1311, found: 270.1314.
2-(Adamantan-1-yl)-6-methylbenzo[d]thiazole (3p).
Yellow solid (114.6 mg, 81%). M.p. 134.4–134.8 °C. 1H NMR (CDCl3, 400 MHz) δ: 7.86 (d, J = 8.3 Hz, 1H), 7.61 (s, 1H), 7.22 (dd, J = 8.3, 1.2 Hz, 1H), 2.44 (s, 3H), 2.13 (s, 9H), 1.79 (s, 6H); 13C NMR (CDCl3, 100 MHz) δ: 180.9, 151.2, 134.4, 134.2, 127.1, 122.1, 121.2, 42.9, 40.0, 36.5, 28.5, 21.3. HRMS (ESI) [M + H]+ calcd for C18H22NS: 284.1467, found: 284.1460.
2-(Adamantan-1-yl)-6-methoxybenzo[d]thiazole (3q).
Yellow solid (116.8 mg, 78%). M.p. 119.2–119.9 °C. 1H NMR (CDCl3, 400 MHz) δ: 7.87 (d, J = 8.8 Hz, 1H), 7.30 (s, 1H), 7.03 (d, J = 8.8 Hz, 1H), 3.83 (s, 3H), 2.12 (s, 9H), 1.80 (s, 6H); 13C NMR (CDCl3, 100 MHz) δ: 179.5, 157.1, 147.6, 135.5, 123.0, 114.7, 104.2, 55.7, 42.9, 39.9, 36.4, 28.5. HRMS (ESI) [M + H]+ calcd for C18H22NOS: 300.1417, found: 300.1412.
2-(Adamantan-1-yl)-4-chlorobenzo[d]thiazole (3r).
White solid (124.2 mg, 82%). M.p. 123.9–124.1 °C. 1H NMR (CDCl3, 400 MHz) δ: 7.71 (dd, J = 8.0, 1.0 Hz, 1H), 7.42 (dd, J = 7.8, 1.0 Hz, 1H), 7.21 (t, J = 7.9 Hz, 1H), 2.14 (s, 9H), 1.80 (s, 6H); 13C NMR (CDCl3, 100 MHz) δ: 182.8, 150.1, 136.0, 127.5, 125.8, 124.8, 120.0, 42.9, 40.5, 36.4, 28.5. HRMS (ESI) [M + H]+ calcd for C17H19ClNS: 304.0921, found: 304.0916.
2-(Adamantan-1-yl)-5-chlorobenzo[d]thiazole (3s).
White solid (121.3 mg, 80%). M.p. 117.2–117.4 °C. 1H NMR (CDCl3, 400 MHz) δ: 7.98 (d, J = 1.9 Hz, 1H), 7.75 (d, J = 8.5 Hz, 1H), 7.30 (dd, J = 8.5, 2.0 Hz, 1H), 2.13 (s, 9H), 1.81 (s, 6H); 13C NMR (CDCl3, 100 MHz) δ: 184.2, 154.1, 132.7, 131.6, 124.8, 122.5, 122.2, 42.9, 40.3, 36.4, 28.5. HRMS (ESI) [M + H]+ calcd for C17H19ClNS: 304.0921, found: 304.0917.
2-(Adamantan-1-yl)-4-bromobenzo[d]thiazole (3t).
White solid (152.9 mg, 88%). M.p. 118.2–119 °C. 1H NMR (CDCl3, 400 MHz) δ: 7.76 (d, J = 8.0 Hz, 1H), 7.60 (dd, J = 7.8, 0.6 Hz, 1H), 7.14 (t, J = 7.8 Hz, 1H), 2.14 (s, 9H), 1.80 (s, 6H); 13C NMR (CDCl3, 100 MHz) δ: 182.5, 151.3, 135.4, 129.0, 125.1, 120.6, 116.3, 42.9, 40.5, 36.4, 28.5. HRMS (ESI) [M + H]+ calcd for C17H19BrNS: 348.0416, found: 348.0411.
2-(Adamantan-1-yl)-5-bromobenzo[d]thiazole (3u).
White solid (137.6 mg, 79%). M.p. 104.5–105 °C. 1H NMR (CDCl3, 400 MHz) δ: 8.14 (d, J = 1.7 Hz, 1H), 7.68 (d, J = 8.4 Hz, 1H), 7.42 (dd, J = 8.4, 1.7 Hz, 1H), 2.12 (s, 9H), 1.80 (s, 6H); 13C NMR (CDCl3, 100 MHz) δ: 184.0, 154.4, 133.2, 127.4, 125.5, 122.5, 119.2, 42.8, 40.3, 36.4, 28.5. HRMS (ESI) [M + H]+ calcd for C17H19BrNS: 348.0416, found: 348.0414.
2-(3-Bromoadamantan-1-yl)benzo[d]thiazole (3v).
White solid (135.3 mg, 78%). M.p. 125.8–126.2 °C. 1H NMR (CDCl3, 400 MHz) δ: 8.00 (d, J = 8.2 Hz, 1H), 7.87 (d, J = 7.9 Hz, 1H), 7.46 (td, J = 7.7, 1.1 Hz, 1H), 7.35 (td, J = 7.6, 1.0 Hz, 1H), 2.74 (s, 2H), 2.42 (d, J = 2.8 Hz, 4H), 2.33 (s, 2H), 2.20–2.12 (m, 4H), 1.80 (s, 2H); 13C NMR (CDCl3, 100 MHz) δ: 178.9, 153.0, 134.2, 126.0, 124.7, 122.8, 121.6, 63.5, 53.3, 48.1, 44.2, 41.1, 34.5, 32.2. HRMS (ESI) [M + H]+ calcd for C17H19BrNS: 348.0416, found: 348.0411.
2-(sec-Butyl)benzo[d]thiazole (3w).
Yellow liquid (45.9 mg, 48%). 1H NMR (CDCl3, 400 MHz) δ: 7.98 (d, J = 8.2 Hz, 1H), 7.85 (dd, J = 7.0, 0.4 Hz, 1H), 7.45 (td, J = 7.7, 1.2 Hz, 1H), 7.34 (td, J = 7.6, 1.1 Hz, 1H), 3.26–3.17 (m, 1H), 1.97–1.73 (m, 2H), 1.45 (d, J = 6.9 Hz, 3H), 0.98 (t, J = 7.4 Hz, 3H); 13C NMR (CDCl3, 100 MHz) δ: 177.9, 153.1, 134.7, 125.8, 124.5, 122.6, 121.5, 41.1, 30.6, 20.7, 11.8. HRMS (ESI) [M + H]+ calcd for C11H14NS: 192.0841, found: 192.0842.
2-(Pentan-2-yl)benzo[d]thiazole (3x).
Yellow liquid (51.2 mg, 50%). 1H NMR (CDCl3, 400 MHz) δ: 7.98 (d, J = 8.1 Hz, 1H), 7.85 (dd, J = 8.0, 0.4 Hz, 1H), 7.44 (td, J = 7.7, 1.2 Hz, 1H), 7.34 (td, J = 7.6, 1.1 Hz, 1H), 3.35–3.26 (m, 1H), 1.91–1.68 (m, 2H), 1.42–1.35 (m, 2H), 0.93 (t, J = 7.3 Hz, 3H); 13C NMR (CDCl3, 100 MHz) δ: 178.1, 153.1, 134.7, 125.8, 124.5, 122.6, 121.5, 39.8, 39.3, 21.1, 20.5, 13.9. HRMS (ESI) [M + H]+ calcd for C12H16NS: 206.0998, found: 206.0999.
5-Bromo-2-(pentan-3-yl)benzo[d]thiazole (3y).
Yellow liquid (86.4 mg, 61%). 1H NMR (CDCl3, 400 MHz) δ: 8.13 (d, J = 1.8 Hz, 1H), 7.69 (d, J = 8.4 Hz, 1H), 7.44 (dd, J = 8.5, 1.9 Hz, 1H), 3.02–2.95 (m, 1H), 1.87–1.78 (m, 4H), 0.91 (t, J = 7.4 Hz, 6H); 13C NMR (CDCl3, 100 MHz) δ: 178.6, 154.3, 133.5, 127.6, 125.5, 122.5, 119.3, 48.7, 48.6, 28.8, 11.8. HRMS (ESI) [M + H]+ calcd for C12H15BrNS: 284.0103, found: 284.0106.
5-Methoxy-2-(pentan-3-yl)benzo[d]thiazole (3z).
Yellow liquid (76.3 mg, 65%). 1H NMR (CDCl3, 400 MHz) δ: 7.86 (d, J = 8.9 Hz, 1H), 7.31 (d, J = 2.5 Hz, 1H), 7.04 (dd, J = 8.9, 2.5 Hz, 1H), 3.86 (s, 3H), 2.98–2.91 (m, 1H), 1.86–1.76 (m, 4H), 0.92 (t, J = 7.4 Hz, 6H); 13C NMR (CDCl3, 100 MHz) δ: 174.2, 157.3, 147.5, 135.9, 123.0, 114.8, 104.4, 55.8, 48.6, 28.9, 11.9. HRMS (ESI) [M + H]+ calcd for C13H18NOS: 236.1104, found: 236.1099.
2-(Hexan-2-yl)benzo[d]thiazole (3aa).
Orange liquid (56.0 mg, 51%). 1H NMR (CDCl3, 400 MHz) δ: 7.98 (d, J = 8.1 Hz, 1H), 7.84 (d, J = 7.8 Hz, 1H), 7.44 (td, J = 7.7, 1.2 Hz, 1H), 7.33 (td, J = 7.6, 1.0 Hz, 1H), 3.32–3.24 (m, 1H), 1.92–1.68 (m, 2H), 1.45 (d, J = 6.9 Hz, 3H), 1.38–1.29 (m, 4H), 0.88 (t, J = 7.0 Hz, 3H); 13C NMR (CDCl3, 100 MHz) δ: 178.1, 153.1, 134.6, 125.7, 124.5, 122.6, 121.5, 39.5, 37.4, 29.5, 22.6, 21.6, 13.9. HRMS (ESI) [M + H]+ calcd for C13H18NS: 220.1154, found: 220.1157.
2-Cyclohexylbenzo[d]thiazole (3ab).
Yellow liquid (56.7 mg, 52%). 1H NMR (CDCl3, 400 MHz) δ: 7.97 (d, J = 8.1 Hz, 1H), 7.84 (d, J = 8.0 Hz, 1H), 7.43 (td, J = 7.7, 1.1 Hz, 1H), 7.33 (td, J = 7.6, 1.0 Hz, 1H), 3.14–3.10 (m, 1H), 2.20 (dd, J = 13.5, 2.0 Hz, 2H), 1.91–1.86 (m, 2H), 1.79–1.74 (m, 1H), 1.69–1.59 (m, 2H), 1.50–1.39 (m, 2H), 1.37–1.26 (m, 1H); 13C NMR (CDCl3, 100 MHz) δ: 177.5, 153.1, 134.5, 125.7, 124.5, 122.5, 121.5, 43.4, 33.4, 26.0, 25.8. HRMS (ESI) [M + H]+ calcd for C13H15NS: 218.0998, found: 218.1000.
Conflicts of interest
There are no conflicts to declare.
Acknowledgements
We gratefully acknowledge the National Natural Science Foundation of China (21772062, 21602072, and 21572078).
Notes and references
-
(a) É. Bokor, S. Kun, T. Docsa, P. Gergely and L. Somsák, ACS Med. Chem. Lett., 2015, 6, 1215 CrossRef PubMed
;
(b) A. L. Kantsadi, É. Bokor, S. Kun, G. A. Stravodimos, D. S. M. Chatzileontiadou, D. D. Leonidas, A. Szakács, G. Batta, T. Docsa, P. Gergely and L. Somsák, Eur. J. Med. Chem., 2016, 123, 737 CrossRef CAS PubMed
;
(c) R. A. Hughes and C. J. Moody, Angew. Chem., Int. Ed., 2007, 46, 7930 CrossRef CAS PubMed
.
-
(a) T. Yao, K. Hirano, T. Satoh and M. Miura, Angew. Chem., Int. Ed., 2012, 51, 775 CrossRef CAS PubMed
;
(b) W.-M. Zhao, X.-L. Chen, J.-W. Yuan, L.-B. Qu, L.-K. Duan and Y.-F. Zhao, Chem. Commun., 2014, 50, 2018 RSC
;
(c) P. Y. Xin, H. Y. Niu, G. R. Qu, R. F. Ding and H. M. Guo, Chem. Commun., 2012, 48, 6717 RSC
;
(d) T. Mukai, K. Hirano, T. Satoh and M. Miura, Org. Lett., 2010, 12, 1360 CrossRef CAS PubMed
;
(e) G. A. Molander, V. Colombel and V. A. Braz, Org. Lett., 2011, 13, 1852 CrossRef CAS PubMed
;
(f) P. Ren, I. Salihu, R. Scopelliti and X. L. Hu, Org. Lett., 2012, 14, 1748 CrossRef CAS PubMed
;
(g) Z. Xie, Y. Cai, H. Hu, C. Lin, J. Jiang, Z. Chen, L. Wang and Y. Pan, Org. Lett., 2013, 15, 4600 CrossRef CAS PubMed
.
-
(a) R. Xia, H.-Y. Niu, G.-R. Qu and H.-M. Guo, Org. Lett., 2012, 14, 5546 CrossRef CAS PubMed
;
(b) Z. Li, L. Jin and C. Cai, Org. Chem. Front., 2017, 4, 2039 RSC
.
-
(a) N. Rodrguez and L. J. Goossen, Chem. Soc. Rev., 2011, 40, 5030 RSC
;
(b) P. Gallezot, Chem. Soc. Rev., 2012, 41, 1538 RSC
;
(c) A. J. J. Straathof, Chem. Rev., 2014, 114, 1871 CrossRef CAS PubMed
.
-
(a) H. Hunsdiecker and C. Hunsdiecker, Chem. Ber., 1942, 75, 291 CrossRef
;
(b) H. Kolbe, Liebigs Ann. Chem., 1849, 69, 257 CrossRef
;
(c) H. Kolbe, Liebigs Ann. Chem., 1848, 64, 339 CrossRef
.
-
(a) R. G. Johnson and R. K. Ingham, Chem. Rev., 1956, 56, 219 CrossRef CAS
;
(b) D. Budac and P. J. Wan, Photochem. Photobiol., A, 1992, 67, 135 CrossRef CAS
;
(c) A. G. Griesbeck, W. Kramer and M. Oelgemoller, Synlett, 1999, 1169 CrossRef CAS
.
- For selected reviews, see:
(a) T. P. Yoon, M. A. Ischay and J. Du, Nat. Chem., 2010, 2, 527 CrossRef CAS PubMed
;
(b) J. M. R. Narayanam and C. R. J. Stephenson, Chem. Soc. Rev., 2011, 40, 102 RSC
;
(c) J. Xuan and W.-J. Xiao, Angew. Chem., Int. Ed., 2012, 51, 6828 CrossRef CAS PubMed
;
(d) C. K. Prier, D. A. Rankic and D. W. C. MacMillan, Chem. Rev., 2013, 113, 5322 CrossRef CAS PubMed
;
(e) M. L. Marin, L. Santos-Juanes, A. Arques, A. M. Amat and M. A. Miranda, Chem. Rev., 2012, 112, 1710 CrossRef CAS PubMed
;
(f) D. Ravelli, M. Fagnoni and A. Albini, Chem. Soc. Rev., 2013, 42, 97 RSC
;
(g) J. K. Matsui, S. B. Lang, D. R. Heitz and G. A. Molander, ACS Catal., 2017, 7, 2563 CrossRef CAS PubMed
;
(h) W.-M. Zhang, J.-J. Dai, J. Xu and H.-J. Xu, J. Org. Chem., 2017, 82, 2059 CrossRef CAS PubMed
.
-
(a) H. Huang, K. Jia and Y. Chen, ACS Catal., 2016, 6, 4983 CrossRef CAS
;
(b) J. Xuan, Z.-G. Zhang and W.-J. Xiao, Angew. Chem., Int. Ed., 2015, 54, 15632 CrossRef CAS PubMed
;
(c) Y. Yoshimi, M. Masuda, T. Mizunashi, K. Nishikawa, K. Maeda, N. Koshida, T. Itou, T. Morita and M. Hatanaka, Org. Lett., 2009, 11, 4652 CrossRef CAS PubMed
;
(d) H. Tan, H. Li, W. Ji and L. Wang, Angew. Chem., Int. Ed., 2015, 54, 8374 CrossRef CAS PubMed
;
(e) J. Liu, Q. Liu, H. Yi, C. Qin, R. P. Bai, X. T. Qi, Y. Lan and A. W. Lei, Angew. Chem., Int. Ed., 2014, 53, 502 CrossRef CAS PubMed
.
-
(a) T. Chinzei, K. Miyazawa, Y. Yasu, T. Koike and M. Akita, RSC Adv., 2015, 5, 21297 RSC
;
(b) J. Wang, G.-X. Li, G. He and G. Chen, Asian J. Org. Chem., 2018, 7, 1307 CrossRef CAS
;
(c) J. Genovino, Y. Lian, Y. Zhang, T. O. Hope, A. Juneau, Y. Gagné, G. Ingle and M. Frenette, Org. Lett., 2018, 20, 3229 CrossRef CAS PubMed
;
(d) L. Chu, C. Ohta, Z. Zuo and D. W. C. MacMillan, J. Am. Chem. Soc., 2014, 136, 10886 CrossRef CAS PubMed
;
(e) J. Xie, P. Xu, H. Li, Q. Xue, H. Jin, Y. Cheng and C. Zhu, Chem. Commun., 2013, 49, 5672 RSC
;
(f) Y. Miyake, K. Nakajima and Y. Nishibayashi, Chem. Commun., 2013, 49, 7854 RSC
.
-
(a) K. Müller, C. Faeh and F. Diederich, Science, 2007, 317, 1881 CrossRef PubMed
;
(b) S. Purser, P. R. Moore, S. Swallow and V. Gouverneur, Chem. Soc. Rev., 2008, 37, 320 RSC
;
(c) W. K. Hagmann, J. Med. Chem., 2008, 51, 4359 CrossRef CAS PubMed
.
- A. R. Katritzky, B. V. Rogovoy, C. Chassaing, V. Vvedensky, B. Forood, B. Flatt and H. Nakai, J. Heterocycl. Chem., 2000, 37, 1655 CrossRef CAS
.
-
(a) E. C. N. Wong, T. A. Reekie, E. L. Werry, J. O'Brien-Brown, S. L. Bowyer and M. Kassiou, Bioorg. Med. Chem. Lett., 2017, 27, 2439 CrossRef CAS PubMed
;
(b) V. Burmistrov, C. Morisseau, D. Pitushkin, D. Karlov, R. R. Fayzullin, G. M. Butov and B. D. Hammock, Bioorg. Med. Chem. Lett., 2018, 28, 2302 CrossRef CAS PubMed
.
- For a selected review, see:
(a) J. Xuan, Z.-G. Zhang and W.-J. Xiao, Angew. Chem., Int. Ed., 2015, 54, 15632 CrossRef CAS PubMed
. For selected examples, see:
(b) C. Cassani, G. Bergonzini and C.-J. Wallentin, Org.
Lett., 2014, 16, 4228 CrossRef CAS PubMed
;
(c) N. P. Ramirez and J. C. Gonzalez-Gomez, Eur. J. Org. Chem., 2017, 2154 CrossRef CAS
;
(d) J. Genovino, Y. Lian, Y. Zhang, T. O. Hope, A. Juneau, Y. Gagné, G. Ingle and M. Frenette, Org. Lett., 2018, 20, 4686 CrossRef PubMed
;
(e) N. Xu, P. Li, Z. Xie and L. Wang, Chem. – Eur. J., 2016, 22, 2236 CrossRef CAS PubMed
.
Footnote |
† Electronic supplementary information (ESI) available. See DOI: 10.1039/c8ob02476f |
|
This journal is © The Royal Society of Chemistry 2019 |
Click here to see how this site uses Cookies. View our privacy policy here.