Visible light promoted coupling of alkynyl bromides and Hantzsch esters for the synthesis of internal alkynes†
Received
22nd November 2018
, Accepted 3rd December 2018
First published on 4th December 2018
Abstract
A metal-free visible light promoted C(sp3)–C(sp) coupling reaction of alkynyl bromides and Hantzsch esters was developed, giving internal alkynes with primary, secondary, tertiary alkyl or other functional groups in good to high yields.
Introduction
Alkynes are essential structural motifs and ubiquitous intermediates in synthetic transformations for building fine chemicals, pharmaceuticals and polymeric photo-/electronic materials.1 The past several decades have witnessed tremendous development of alkyne synthesis in organic chemistry. The general approaches via the C(sp3)–C(sp) bond formation rely on the addition reaction with organometallic reagents,2 and transition-metal-catalyzed coupling reactions using Pd,3 Cu,4 Co,5 Ni,6 Ag7 and Fe8 complexes (Scheme 1, reaction (a)). However, the tedious reaction conditions and the use of relatively expensive metals led chemists to explore alternative protocols under mild conditions in an environmentally benign manner.9
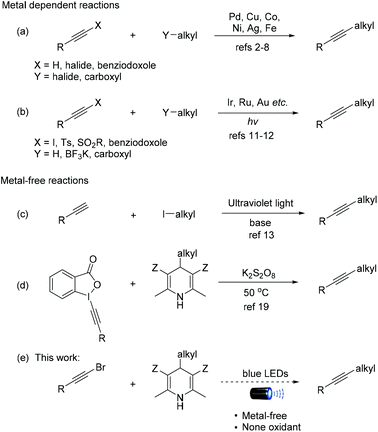 |
| Scheme 1 Synthesis of internal alkynes via C(sp3)–C(sp) coupling. | |
The visible-light-induced catalysis is becoming a fascinating methodology in modern organic synthesis,10 including those for C(sp3)–C(sp) coupling reactions (Scheme 1, reaction (b)). For example, in 2014, Chen et al. reported a visible-light-induced deboronative alkynylation reaction with [Ru(bpy)3](PF6)2/BI–OH.11 This reaction worked with alkyl trifluoroborates or boronic acids to generate substituted alkynes. In 2015, Hashmi et al. reported a gold-catalyzed radical C(sp3)–H alkynylation of tertiary aliphatic amines using readily available 1-iodoalkynes as a radical alkynylation reagent in the presence of [Au2(μ-dppm)2]2+ under photocatalysis conditions.12 The reaction shows excellent regioselectivity and good functional-group compatibility. However, these reactions require expensive transition metals/ligands and limitations of the substrate scope. In 2015, Li et al. reported a pioneering metal-free coupling of an alkyne and alkyl iodide under ultraviolet irradiation (Scheme 1, reaction (c)).13
Recently, Hantzsch esters (HEs)14 have been extensively used in transfer-hydrogenation reactions and transfer-alkylation reactions, especially in photoredox reactions.15 In 2011, Nishibayashi and coworkers reported the first visible-light-induced reaction of HEs via alkyl radicals.16 After that, the reactions of HEs have been successfully used for the C(sp3)–C(sp3)17 and C(sp3)–C(sp2)18 bond formation. Very recently, Cheng and co-workers reported the alkynylation of HEs with benziodoxole-activated alkynes under oxidative conditions (Scheme 1, reaction (d)).19 In this paper, we report a visible-light-induced radical C(sp3)–C(sp) coupling of alkynyl bromides and Hantzsch esters for the synthesis of internal alkynes (Scheme 1, reaction (e)). Our work provides a photocatalytic approach from readily available alkynyl bromides without any additional oxidant, which leads to good tolerance of functional groups.
Results and discussion
Initially, the reaction of 1-bromo-2-phenylacetylene (1a) with 4-cyclohexyl Hantzsch ester (2a) was investigated under photocatalysis (Table 1). We were encouraged to find that the desired cyclohexyl alkyne (3aa) was obtained in 19% yield when the reaction was carried out with 2 mol% of 4CzIPN (2,4,5,6-tetra(9H-carbazol-9-yl)isophthalonitrile) as the photocatalyst in dichloromethane at room temperature for 12 h under the irradiation of blue LEDs (entry 1). The yield was increased to 66% when the reaction time was prolonged to 36 h (entry 2). The addition of a silver salt showed no apparent improvement (entry 3). Screening of solvents and bases revealed that 1,2-dichloroethane is the best choice (entries 4–8), and K3PO4 performed best compared to other inorganic and organic bases (entries 8–12). Evaluation of photocatalysts showed that Ir- and Ru-based photocatalysts only afforded the product with a slightly increased yield (entries 13 and 14), while the organic photocatalyst of 10-methyl-9-mesitylacridinium gave only a trace amount of product (entry 15). Considering the environmental benignity of organic photocatalysts compared to transitional metals, the metal-free photocatalyst 4CzIPN was chosen for the reaction. A higher yield was achieved when the loading of Hantzsch ester 2a was increased to two equivalents (entry 16). It is worth noting that the reaction using alkynyl chloride (1a′) or sulfone (1a″) instead of bromide (1a) resulted in some loss of yield (entries 17 & 18).
Table 1 Optimization of the alkynylation reactiona
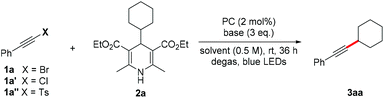
|
Entry |
1
|
PC |
Base |
Solvent |
Yieldb (%) |
Unless otherwise specified, 1a (0.2 mmol), 2a (0.3 mmol), photocatalyst (2 mol%), base (0.6 mmol), solvent (4 mL, [0.5 M]), rt.
Yields determined by 1H NMR (400 MHz) spectroscopy with CH2Br2 as the internal standard.
The reaction was terminated after 12 h.
AgNO3 (0.2 mmol) was added as an additive.
2a (0.4 mmol) was used.
Isolated yields in parentheses. DCE = 1,2-dichloroethane, 4CzIPN = 2,4,5,6-tetra(9H-carbazol-9-yl)isophthalonitrile, ppy = 2-phenylpyridine, Mes-Acr-Me = 10-methyl-9-mesitylacridinium.
|
1c |
1a
|
4CzIPN |
K3PO4 |
CH2Cl2 |
19 |
2 |
1a
|
4CzIPN |
K3PO4 |
CH2Cl2 |
66 |
3d |
1a
|
4CzIPN |
K3PO4 |
CH2Cl2 |
67 |
4 |
1a
|
4CzIPN |
K3PO4 |
DMF |
Trace |
5 |
1a
|
4CzIPN |
K3PO4 |
DMSO |
45 |
6 |
1a
|
4CzIPN |
K3PO4 |
MeCN |
55 |
7 |
1a
|
4CzIPN |
K3PO4 |
Acetone |
57 |
8 |
1a
|
4CzIPN |
K3PO4 |
DCE |
70 |
9 |
1a
|
4CzIPN |
Cs2CO3 |
DCE |
60 |
10 |
1a
|
4CzIPN |
NaOAc |
DCE |
51 |
11 |
1a
|
4CzIPN |
t-BuOK |
DCE |
NR |
12 |
1a
|
4CzIPN |
DBU |
DCE |
22 |
13 |
1a
|
fac-Ir(ppy)3 |
K3PO4 |
DCE |
71 |
14 |
1a
|
Ru(ppy)3Cl2 |
K3PO4 |
DCE |
76 |
15 |
1a
|
Mes-Acr-Me |
K3PO4 |
DCE |
3 |
16e |
1a
|
4CzIPN |
K3PO4 |
DCE |
83(75)f |
17e |
1a′
|
4CzIPN |
K3PO4 |
DCE |
(45)f |
18e |
1a″
|
4CzIPN |
K3PO4 |
DCE |
(21)f |
With the optimized reaction conditions in hand, we then investigated the substrate scope. A variety of arylethynyl bromides worked well for the reaction (Table 2). Both electron-donating groups (1b–1f) and electron-withdrawing groups (1g–1m) were tolerated at the ortho-, meta- or para-position, giving the internal alkynes 3aa–3ma in good to high yields (entries 1–13). All arylethynyl bromides with 4-phenyl or α-naphthyl and heteroarylethynylbromide derived from indole or thiophene worked as well (3na–3qa) (entries 14–17). Notably, the ester and ketone were also tolerable for the reaction to give the corresponding product (3ra) and estrone-derived alkyne (3sa) in good yields (entries 18 &19). The reaction of a series of 4-alkyl Hantzsch esters is shown in Table 3. It was found that benzyl, acyclic and cyclic secondary alkyl could be easily coupled with the alkynyl bromides to give the products (3db–3ld) in good to high yields (entries 1–4). More importantly, the tertiary alkyl, which is difficult to introduce by the classical nucleophilic substitution reaction, worked best due to the relative stability of their radicals (3ae–3qe) (entries 5–11). It should be noted that Meyer nitrile 2e
20 was used instead of Hantzsch esters due to the difficulty in their synthesis. Furthermore, the reaction of the piperidine-derived Hantzsch ester went smoothly to give product 3df in 65% yield (entry 12).
Table 2 Scope of alkynyl bromides
Table 3 Scope of 4-alkyl Hantzsch esters
The scale-up reaction using 1.05 g (5 mmol) of alkynyl bromide 1e gave product 3ea in 78% yield (Scheme 2).
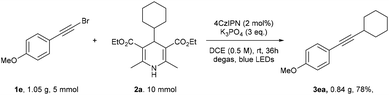 |
| Scheme 2 Gram-scale experiment. | |
The photocatalytic alkynyl–alkyl coupling reaction is believed to proceed via a radical intermediate.21 Control experiments revealed that the reaction was completely suppressed with no desired product detected when TEMPO (2,2,6,6-tetramethyl-1-oxylpiperdine) was added as the radical-trapping reagent (Scheme 3).
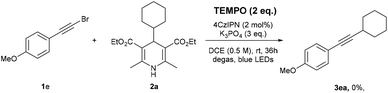 |
| Scheme 3 Radical-trapping reaction. | |
The plausible mechanism for the reaction is shown in Fig. 1. The readily available photocatalyst 4CzIPN is irradiated under blue LEDs to the photoexcited state (4CzIPN*), which features a high redox potential 
22 (PC = 4CzIPN) to promote the photooxidative reaction. The substituted Hantzsch esters (Ered1/2 = +1.08 V vs. SCE)17a were cleaved by the oxidation of photoexcited 4CzIPN* to generate the alkyl radical I and radical anion II. The addition of alkyl radical I to arylethynylbromide affords the bromoalkenyl radical III,23 which is collapsed to give alkylalkyne 3 and a bromine radical.24 The reduction of the bromine radical by radical anion species II
25 regenerates the photocatalyst and furnishes the catalytic cycle.
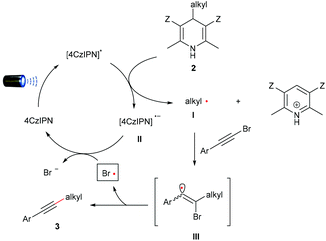 |
| Fig. 1 Plausible reaction mechanism. | |
Conclusions
In summary, the photocatalytic synthesis of internal alkynes via C(sp3)–C(sp) bond coupling of bromoalkynes and Hantzsch esters was developed. All the primary, secondary and tertiary alkyl radicals generated from the Hantzsch ester derivatives worked well for the reaction. The reaction features good tolerance of many functional groups, including esters and ketones, under metal-free conditions.
Experimental
Unless otherwise indicated, all reactions were carried out with N2 protection with magnetic stirring. Anhydrous CH2Cl2 was distilled from CaH2. Column chromatography was performed on silica gel (200–300 mesh). All 1H NMR (300 or 400 or 500 MHz) and 13C NMR (75 or 101 or 126 MHz) spectra were recorded on a Bruker-DMX 300 or 400 or 500 spectrometer in CDCl3, with CH2Br2 as an internal standard and reported in parts per million (ppm, δ). 1H NMR spectroscopy splitting patterns were designated as singlet (s), doublet (d), triplet (t), quartet (q). Splitting patterns that could not be interpreted or easily visualized were designated as multiplet (m) or broad (br). Infrared spectra were recorded on a Nicolet 6700 spectrophotometer and reported in wavenumbers (cm−1).
Visible light promoted coupling of alkynyl bromides and Hantzsch esters
General procedure.
To a solution of alkynyl bromides 1 (0.2 mmol) in a 10 mL reaction tube were added Hanzsch ester 2 (0.4 mmol, 2.0 equiv.), 4CzIPN (3.15 mg, 0.004 mmol, 2 mol%) and K3PO4 (127 mg, 0.6 mmol, 3.0 equiv.) under a nitrogen atmosphere at room temperature. The tube was sealed and 4 mL of DCE were injected. Then the solvent was degassed 3 times. The reaction mixture was stirred for 36 h at room temperature and irradiated by 24 W blue LEDs. After the completion of the reaction, the mixture was diluted with H2O and extracted with DCM 3 times. The combined organic layers were washed with brine, dried over Na2SO4, filtered and evaporated under reduced pressure. The residue was purified by chromatography on silica gel to give the desired coupling product 3.
Radical-trapping experiment
To a solution of alkynyl bromides 1e (42 mg, 0.2 mmol) in a 10 mL reaction tube were added Hanzsch ester 2a (134 mg, 0.4 mmol, 2.0 equiv.), 4CzIPN (3.15 mg, 0.004 mmol, 2 mol%), TEMPO (2,2,6,6-tetramethyl-1-oxylpiperidine, 62.5 mg, 0.4 mmol, 2.0 equiv.) and K3PO4 (127 mg, 0.6 mmol, 3.0 equiv.) under a nitrogen atmosphere at room temperature. The tube was sealed and 4 mL of DCE was injected. Then the solvent was degassed 3 times. The reaction mixture was stirred for 36 h at room temperature and irradiated by 24 W blue LEDs. No desired product was observed by TLC.
Conflicts of interest
There are no conflicts of interest to declare.
Acknowledgements
Financial support from the National Natural Science Foundation of China (no.: 21425207, 21521002, 21672216) is gratefully acknowledged.
Notes and references
-
(a) E. Negishi and L. Anastasia, Chem. Rev., 2003, 103, 1979 CrossRef CAS PubMed;
(b) G. Zeni and R. C. Larock, Chem. Rev., 2004, 104, 2285 CrossRef CAS PubMed;
(c) M. C. Willis, Chem. Rev., 2010, 110, 725 CrossRef CAS PubMed;
(d) Z.-Z. Zhang, B. Liu, C.-Y. Wang and B.-F. Shi, Org. Lett., 2015, 17, 4094 CrossRef CAS PubMed;
(e) W. Liu, Z. Chen, L. Li, H. Wang and C.-J. Li, Chem. – Eur. J., 2016, 22, 5888 CrossRef CAS PubMed.
-
(a) J. P. Brand and J. Waser, Chem. Soc. Rev., 2012, 41, 4165 RSC;
(b) T. Bauer, Coord. Chem. Rev., 2015, 299, 83 CrossRef CAS.
-
(a) J. Y. Kang and B. T. Connell, J. Org. Chem., 2011, 76, 6856 CrossRef CAS PubMed;
(b) G. A. Molander and K. M. Traister, Org. Lett., 2013, 15, 5052 CrossRef CAS PubMed;
(c) M.-M. Zhang, J. Gong, R.-J. Song and J.-H. Li, Eur. J. Org. Chem., 2014, 6769 CrossRef CAS;
(d) H.-C. Shen, P.-S. Wang, Z.-L. Tao, Z.-Y. Han and L.-Z. Gong, Adv. Synth. Catal., 2017, 359, 2383 CrossRef CAS.
-
(a) H. P. Bi, L. Zhao, Y. M. Liang and C. J. Li, Angew. Chem., Int. Ed., 2009, 48, 792 CrossRef CAS PubMed;
(b) H. P. Bi, Q. F. Teng, M. Guan, W. W. Chen, Y. M. Liang, X. J. Yao and C. J. Li, J. Org. Chem., 2010, 75, 783 CrossRef CAS PubMed;
(c) G. Cahiez, O. Gager and J. Buendia, Angew. Chem., Int. Ed., 2010, 49, 1278 CrossRef CAS PubMed;
(d) Y.-S. Feng, Z.-Q. Xu, L. Mao, F.-F. Zhang and H.-J. Xu, Org. Lett., 2013, 15, 1472 CrossRef CAS PubMed;
(e) K. Indukuri and O. Riant, Adv. Synth. Catal., 2017, 359, 2425 CrossRef CAS;
(f) C. Minozzi, A. Caron, J. C. Grenier-Petel, J. Santandrea and S. K. Collins, Angew. Chem., Int. Ed., 2018, 57, 5477 CrossRef CAS PubMed;
(g) J. Skotnitzki, V. Morozova and P. Knochel, Org. Lett., 2018, 20, 2365 CrossRef CAS PubMed.
- H. Ohmiya, H. Yorimitsu and K. Oshima, Org. Lett., 2006, 8, 3093 CrossRef CAS PubMed.
-
(a) J. Yi, X. Lu, Y.-Y. Sun, B. Xiao and L. Liu, Angew. Chem., Int. Ed., 2013, 52, 12409 CrossRef CAS PubMed;
(b) Q.-H. Li, Y. Ding and X.-J. Yang, Chin. Chem. Lett., 2014, 25, 1296 CrossRef CAS;
(c) P. M. P. Garcia, P. Ren, R. Scopelliti and X. L. Hu, ACS Catal., 2015, 5, 1164 CrossRef;
(d) L. Huang, A. M. Olivares and D. J. Weix, Angew. Chem., Int. Ed., 2017, 56, 11901 CrossRef CAS PubMed;
(e) W. Li, J. K. Boon and Y. Zhao, Chem. Sci., 2018, 9, 600 RSC.
-
(a) R. H. Pouwer, C. M. Williams, A. L. Raine and J. B. Harper, Org. Lett., 2005, 7, 1323 CrossRef CAS PubMed;
(b) X. Liu, Z. Wang, X. Cheng and C. Li, J. Am. Chem. Soc., 2012, 134, 14330 CrossRef CAS PubMed.
- Y. Shen, B. Huang, J. Zheng, C. Lin, Y. Liu and S. Cui, Org. Lett., 2017, 19, 1744 CrossRef CAS PubMed.
-
(a) S. K. Khetan and T. J. Collins, Chem. Rev., 2007, 107, 2319 CrossRef CAS PubMed;
(b) P. Anastas and N. Eghbali, Chem. Soc. Rev., 2010, 39, 301 RSC.
-
(a) A. Albini and M. Fagnoni, Green Chem., 2004, 6, 1 RSC;
(b) T. Hoshikawa, S. Kamijo and M. Inoue, Org. Biomol. Chem., 2013, 11, 164 RSC;
(c) F. Le Vaillant, T. Courant and J. Waser, Angew. Chem., Int. Ed., 2015, 54, 11200 CrossRef CAS PubMed;
(d) J. Yang, J. Zhang, L. Qi, C. Hu and Y. Chen, Chem. Commun., 2015, 51, 5275 RSC;
(e) K. Jia, F. Zhang, H. Huang and Y. Chen, J. Am. Chem. Soc., 2016, 138, 1514 CrossRef CAS PubMed.
- H. Huang, G. Zhang, L. Gong, S. Zhang and Y. Chen, J. Am. Chem. Soc., 2014, 136, 2280 CrossRef CAS PubMed.
- J. Xie, S. Shi, T. Zhang, N. Mehrkens, M. Rudolph and A. S. Hashmi, Angew. Chem., Int. Ed., 2015, 54, 6046 CrossRef CAS PubMed.
- W. Liu, L. Li and C.-J. Li, Nat. Commun., 2015, 6, 6526 CrossRef CAS PubMed.
-
(a) G. Li, R. Chen, L. Wu, Q. Fu, X. Zhang and Z. Tang, Angew. Chem., Int. Ed., 2013, 52, 8432 CrossRef CAS PubMed;
(b) G. Li, L. Wu, G. Lv, H. Liu, Q. Fu, X. Zhang and Z. Tang, Chem. Commun., 2014, 50, 6246 RSC.
-
(a) Y. Chen, A. S. Kamlet, J. B. Steinman and D. R. Liu, Nat. Chem., 2011, 3, 146 CrossRef CAS PubMed;
(b) M. H. Larraufie, R. Pellet, L. Fensterbank, J. P. Goddard, E. Lacote, M. Malacria and C. Ollivier, Angew. Chem., Int. Ed., 2011, 50, 4463 CrossRef CAS PubMed;
(c) C. Zheng and S.-L. You, Chem. Soc. Rev., 2012, 41, 2498 RSC;
(d) L. J. Rono, H. G. Yayla, D. Y. Wang, M. F. Armstrong and R. R. Knowles, J. Am. Chem. Soc., 2013, 135, 17735 CrossRef CAS PubMed;
(e) L. Qi and Y. Chen, Angew. Chem., Int. Ed., 2016, 55, 13312 CrossRef CAS PubMed;
(f) C. Wang, K. Harms and E. Meggers, Angew. Chem., Int. Ed., 2016, 55, 13495 CrossRef CAS PubMed;
(g) J. Zhang, Y. Li, F. Zhang, C. Hu and Y. Chen, Angew. Chem., Int. Ed., 2016, 55, 1872 CrossRef CAS PubMed;
(h) K. N. Lee, Z. Lei and M.-Y. Ngai, J. Am. Chem. Soc., 2017, 5003 CrossRef CAS PubMed.
- K. Nakajima, S. Nojima, K. Sakata and Y. Nishibayashi, ChemCatChem, 2016, 8, 1028 CrossRef CAS.
-
(a) W. Chen, Z. Liu, J. Tian, J. Li, J. Ma, X. Cheng and G. Li, J. Am. Chem. Soc., 2016, 138, 12312 CrossRef CAS PubMed;
(b) W. Huang, W. Chen, G. Wang, J. Li, X. Cheng and G. Li, ACS Catal., 2016, 6, 7471 CrossRef CAS;
(c) H.-H. Zhang and S. Yu, J. Org. Chem., 2017, 82, 9995 CrossRef CAS PubMed;
(d) F. J. Gu, W. H. Huang, X. Liu, W. X. Chen and X. Cheng, Adv. Synth. Catal., 2018, 360, 925 CrossRef CAS;
(e) C. Verrier, N. Alandini, C. Pezzetta, M. Moliterno, L. Buzzetti, H. B. Hepburn, A. Vega-Peñaloza, M. Silvi and P. Melchiorre, ACS Catal., 2018, 8, 1062 CrossRef CAS.
-
(a) A. Gutierrez-Bonet, J. C. Tellis, J. K. Matsui, B. A. Vara and G. A. Molander, ACS Catal., 2016, 6, 8004 CrossRef CAS PubMed;
(b) K. Nakajima, S. Nojima and Y. Nishibayashi, Angew. Chem., Int. Ed., 2016, 55, 14106 CrossRef CAS PubMed;
(c) L. Buzzetti, A. Prieto, S. R. Roy and P. Melchiorre, Angew. Chem., Int. Ed., 2017, 56, 15039 CrossRef CAS PubMed;
(d) A. Gutierrez-Bonet, C. Remeur, J. K. Matsui and G. A. Molander, J. Am. Chem. Soc., 2017, 139, 12251 CrossRef CAS PubMed.
- X. Liu, R. Liu, J. Dai, X. Cheng and G. Li, Org. Lett., 2018, 20, 6906 CrossRef CAS PubMed.
- E. v. Meyer, J. Prakt. Chem., 1908, 78, 497 CrossRef.
-
(a) X.-Q. Zhu, Y.-C. Liu and J.-P. Cheng, J. Org. Chem., 1999, 64, 8980 CrossRef CAS;
(b) G. Park, S. Y. Yi, J. Jung, E. J. Cho and Y. You, Chem. – Eur. J., 2016, 22, 17790 CrossRef CAS PubMed;
(c) L. Yuan, G.-B. Shen, Y.-H. Fu and X.-Q. Zhu, J. Phys. Org. Chem., 2017, 30, e3599 CrossRef.
- E. Speckmeier, T. G. Fischer and K. Zeitler, J. Am. Chem. Soc., 2018, 140, 15353 CrossRef CAS PubMed.
- J. Zhang, P. Li and L. Wang, Org. Biomol. Chem., 2014, 12, 2969 RSC.
- L. Eberson, M. P. Hartshorn, J. J. McCullough, O. Persson and F. Radner, Acta Chem. Scand., 1998, 52, 1024 CrossRef CAS.
- J. Luo and J. Zhang, ACS Catal., 2016, 6, 873 CrossRef CAS.
Footnote |
† Electronic supplementary information (ESI) available. See DOI: 10.1039/c8ob02912a |
|
This journal is © The Royal Society of Chemistry 2019 |
Click here to see how this site uses Cookies. View our privacy policy here.