Dietary supplementation of vitamin C: an effective measure for protection against UV-B irradiation using fish as a model organism
Received
26th October 2018
, Accepted 3rd November 2018
First published on 5th November 2018
Abstract
The development of UV-B protective mechanisms in aquacultural species is essential for the sustainable production of healthy aqua crop. Freshwater carp Catla catla larvae (13.5 ± 1.12 mg) were fed with a diet containing 0.5% vitamin C (D1) and a control diet (D2) for 40 days. Each group was exposed to two doses of UV-B irradiation: 360 (5 min, D15 min and D25 min) and 720 mJ cm−2 (10 min, D110 min and D210 min) for 15 days. Significantly (p < 0.05) higher survival and average weight were recorded in D1 compared to D2 exposed to the same dose. Also, significantly (p < 0.001) higher nitric oxide synthase and lower thiobarbituric acid reactive substances and heat shock protein 70 levels were recorded in D15 min compared to the other groups. A direct relationship was found between the dose of UV-B and DNA fragmentation in muscles. DNA damage indices such as tail DNA, tail extent moment and olive tail moment were significantly (p < 0.01) lower in D15 min. Thus, supplementation of vitamin C in the diet provides UV-B protection to larvae.
Introduction
The freshwater ecosystem is a rich source of biodiversity. Solar ultraviolet radiation B (UV-B) is an environmental stressor, which affects the freshwater environment, and the organisms that inhabit it are particularly vulnerable because they are isolated and fragmented within a terrestrial landscape.1 The amount of ultraviolet radiation reaching the Earth's surface started increasing in the middle of the 20th century due to the deterioration of the stratospheric ozone layer.2 In water, UV radiation is absorbed by dissolved organic material, but water itself hardly absorbs it.3 Hence, UV-B penetration has been observed to a depth of 55 m2. In shallow rivers and streams with a low dissolved organic carbon concentration, UV-B radiation can penetrate to the bottom.2,4 DNA damage induced by UV-B exposure is mainly due to the direct excitation of its bases, leading to mutations and chromosome fragmentation.5,6 The alterations most frequently induced are cyclobutane pyrimidine dimers (CPDs) and pyrimidine (6-4) pyrimodone photoproducts (6-4PPs).7 These products can block DNA replication, alter gene expression by preventing transcription and cause mutagenesis or cell death.6,8,9 The harmful effects of UV-B radiation at the organism level include increased oxidative stress, impairment in larval development, reduced movement, changes in fatty acid profiles, skin lesions, and development of cataracts and lesions to the brain.10–13
Long term UV-B irradiation affects the immune system of carps14–16 and rainbow trout by modulating the functions of head kidney phagocytes and lymphocytes.13 Exposure to UV-B radiation increases the activities of enzymes protecting against oxidative stress and also affects the molecular defence mechanism.2,17 Some cells express protein from the family heat shock cognates 70 (Hsp 70) by a variety of environmental stress including UV-B radiation, which generates denatured proteins.18,19 Hsp 70 plays a protective role against tissue damage by binding to denatured proteins and attempting to restore their tertiary structure and functions.20,21
Evaluation of the response of freshwater fishes to UV-B radiation is significant since it helps in understanding the harmful effect of UV-B radiation at the organism level and simultaneously on the production of aqua crop. Catla is a eurythermal species that grows best at a water temperature ranging between 25–32 °C. They are typically surface-dwelling, zooplanktivores and usually participate in seeding migration by moving towards shallow water during monsoon season.22 However, the natural UV-B radiation is high in monsoon months and can easily penetrate the water. In Lake Naini, Delhi, India, the highest UV-B radiation (236.2 μW cm−2) was recorded in the month of June.23 Also, larvae cultured in open nursery ponds receive direct exposure to sunlight, which initiates changes in their overall physiology.
Vitamin C is one of the powerful reducing agents available to cells, serving as a co-factor for the incorporation of molecular oxygen into various substrates. Dietary supplementation of vitamin C during stressful periods facilitates efficient functioning of the immune and endocrine systems.24 Dietary supplementation of vitamin C also increases the regeneration ability of several fish.25 Fish and crustacean cannot synthesize vitamin C since they lack the enzyme gulonolactone oxidase, which is necessary in the last step of its biosynthesis.26 In the present study, we evaluated the impact of two different doses of UV-B radiation on the physiological responses of Catla catla larvae, which were used as a test model. Simultaneously, an attempt was made to confirm the ameliorating effect of dietary supplementation of the antioxidant vitamin C to reduce the harmful effects of UV-B radiation.25
Materials and methods
Experimental design
Catla catla, catla larvae were collected from the Mogra fish farm, West Bengal, India and brought to the wet laboratory. Larvae were acclimated 7 days. Then the larvae (13.5 ± 1.12 mg) were divided into two groups and introduced into aquarium (15 L) following the randomised block design. The stocking density was 20 larvae per aquarium. Larvae were cultured in dechlorinated tap water and the depth of water in each aquarium was 20 cm. The larvae were fed two types of diets: the test diet containing 0.5% vitamin C (D1) and the control diet (D2) without vitamin C (Table 1) at the rate of 5% of body weight. The water temperature was 28.5 °C ± 1.5 °C, pH ranged from 7.5–7.8 and the dissolved oxygen level was 5.5 ± 0.5 mg L−1. The larvae were exposed to UV-B radiation after 40 days of feeding. The impact of UV-B radiation was compared with the unexposed control in an earlier study. In the unexposed control catla larvae, their survival, growth, digestive enzyme activities and immunological parameters (lysozyme and nitric oxide synthase) were significantly (p < 0.05) higher compared to the UV-B irradiated larvae. Significantly (p < 0.05) lower carbonyl protein, thiobarbituric acid reactive substances, superoxide dismutase and heat shock protein 70 levels were found in the control unexposed catla compared to the exposed ones.14,23 Therefore, the present investigation focused on the positive effect of dietary supplementation of vitamin C to overcome the harmful effect of UV-B in carp larvae. The larvae fed without the vitamin C supplemented diet served as the control in the present study. All experiments were performed in accordance with the guidelines and regulations of the experiments with fish, in the Department of Zoology, University of Delhi. The experimental protocols were approved by the University of Delhi.
Table 1 Ingredients used for the preparation of the test and control diets
Ingredients (g kg−1 diet) |
Test diet (D1) |
Control diet (D2) |
Sea Cod (Sanofi India Limited, Mumbai, India): omega-3-PUFA, EPA and DHA.
Supradyn (Bayer Consumer Care AG, Switzerland): vitamin A = 10 000 IU, C = 150 mg, and E = 25 mg.
|
Dry fish powder |
583.3 |
583.3 |
Wheat flour |
400.2 |
402.7 |
Cod liver oila |
10.0 |
10.0 |
Vitamin-mineral premixb |
4.0 |
4.0 |
Vitamin C |
5.0 |
— |
Proximate analysis (% dry matter basis)
|
Crude protein |
42.1 |
44.63 |
Crude fat |
6.48 |
7.1 |
Energy value (cal g−1) |
3.49 |
3.6 |
UV-B exposure
The larvae fed with test diet (D1) and control diet (D2) were exposed to UV-B irradiation for 5 (D15 min and D25 min) and 10 (D110 min and D210 min) min daily for 15 days at a mean radiant energy of 80 μW cm−2. The total cumulative dose of 360/720 mJ cm−2 was received in the 5/10 min-exposed larvae during 15 days of exposure. Three replicates were used for each treatment. The individual aquarium was covered with a black plastic sheet to avoid outside light and reflectance between the aquarium itself. A Philips tube light (TL 20/12 RS, Holland) was used as the source of UV-B radiation (280–320 nm). The light was fixed at 20 cm above each aquarium. At this level the intensity of the light was 80 μW cm−2. All the tubes were pre-burned for 100 h to give a stable output.12,27,28 The ultraviolet radiation B at the water surface was measured using a Microtops II Sunphotometer (Solar Light Company, Philadelphia, USA). The larvae were kept under fluorescent lamps (Philips 20 W) without UV components following a photoperiod of 12 h:12 h.
Sampling
Larvae were collected and anaesthetised with MS-222 (0.01%) after 24 h of the last UV-B exposure. The survival rate and weight of each catla were recorded. Then muscle tissue sample was collected from the individual catla, pooled and stored immediately at −80 °C for various assays. For the DNA damage (Comet Assay) and heat shock protein (Hsp 70) study, the tissue was immediately processed for analysis.
Biochemical assays
Total tissue protein was determined following the method of Lowry et al.29 The optical density of the sample was recorded at 750 nm using a microplate reader (Synergy HT, Biotech, USA). Tissue glutamic oxaloacetic transaminase (GOT) and tissue glutamate pyruvate transaminase (GPT) were determined using diagnostic kits (Bayer Diagnostics, Baroda, India). Absorbance was measured at 340 nm in kinetic mode using a microplate reader and levels were expressed as IU L−1. Thiobarbituric acid reactive substances were measured following the method of Ohkawa et al.30 Absorbance was recorded at 532 nm. The standard was prepared using 1,1,3,3-tetramethoxy propane. The result was expressed as μmol MDA per mg protein. Lysozyme activity was determined by incubating 10 μL of tissue suspension with 1 mL of Micrococcus lysodeicticus (Sigma-Aldrich, St Louis, USA) suspended in acetate buffer (0.02 M, pH 5.5) for 60 min at room temperature.31 The absorbance was measured at 450 nm and expressed as μg per mg tissue. The standard was prepared using hen's egg lysozyme (Sigma-Aldrich, St Louis, USA). Nitric oxide synthase was measured following the method of Lee et al.32 The absorbance was recorded at 540 nm. The nitrite concentration was expressed as mM per mg tissue. Heat shock protein 70 concentrations in muscles were quantified using the Hsp 70 ELISA kit (CUSABIO, Wuhan, China). Absorbance was recorded at 450 nm and expressed as pg mL−1.
Study of DNA damage by single cell gel electrophoresis (Comet assay)
DNA damage was measured using the Comet assay method.33,34 The slide was kept in methanol overnight and burnt to avoid contamination from machine oil and dust.35 A layer of 0.1% agarose (low melting, Sigma-Aldrich, St Louis, USA) was uniformly spread on the slide (Sigma-Aldrich, St Louis) and dried at 45 °C using slide warmer (Hicon, India). This pre-coated slide was stored for future use. Tissue was minced into small pieces using a scissors in cold Hank's Blank Salt solution containing 20 mM of ethylenediaminetetraacetic acid (EDTA, Himedia, Mumbai, India) and diluted (1
:
30) with phosphate buffer saline, PBS (pH 7.4). The diluted muscle (10 μL) sample was mixed with 600 μL of 0.75% agarose. Then it was layered on the microscope slide pre-coated with 0.1% agarose.33 The slide was kept at 4 °C for 5 min to allow the formation of agarose gel. Then the slide was incubated in lysis buffer (pH 9.5) made up of 2.5% sodium dodecyl sulphate (Himedia, Mumbai, India), 1% sodium sarcosinate (Sigma-Aldrich, St Louis) and 25 mM EDTA for 15 min at 25–30 °C. Then it was washed in distilled water for 5 min at 10 °C and electrophoresed at 2 V cm−1 (Amersham Biosciences, USA) for 5 min at 10 °C. After a brief rinse in distilled water, the slide was dried at 45 °C on a slide warmer (Hicon). Then the slides was stained with propidium iodide (25 μg mL−1, Sigma-Aldrich, St Louis) after brief rehydration in distilled water for 5 min. The stained slide was observed, and its image acquired using an Axio Imager Upright Microscope (2.2 Carl Zeiss, Germany). Analysis of DNA distribution in the comet was carried out with comet imager (version 2.2, Metasystem). Fifty comets from different slides were measured for each replicate. Three replicates were used for each treatment. The scored parameters including tail length, tail moment and olive tail moment were studied to quantify the DNA damage. The detailed description of the calculation was provided in the software.
Statistical analysis
Results were expressed as mean ± SEM with a significance value using the t-test statistic to compare the differences between the doses and diets. Further, to study the interaction and main effect, two-way analysis of variance (ANOVA) was conducted using the SPSS software 19.0.
Results
Survival and growth of larvae
The effect of different doses of UV-B radiation on the performance of catla larvae and the role of vitamin C in reducing the harmful effect of radiation were evaluated in the present investigation. The survival rate and average weight were significantly (p < 0.05) lower in the larvae exposed to 720 mJ cm−2 radiation compared to that at 360 mJ cm−2 for both feeding regimes. A significantly (p < 0.05) higher survival rate and average weight were recorded in the vitamin C supplemented diet-fed catla compared to the control group exposed to the same UV-B dose (Table 2). The two-way analysis of variance (ANOVA) of the survival and the average weight showed a significant main effect for the diet and UV-B dose, but the interaction between the UV-B and diet was insignificant (p > 0.05).
Table 2 Effect of vitamin C on the survival, average weight, tissue glutamic oxaloacetic transaminase (GOT), tissue glutamate pyruvate transaminase (GPT), thiobarbituric acid reactive substances (TBARS), lysozyme, nitric oxide synthase, heat shock protein 70 (Hsp 70) and DNA damage in catla exposed to two different doses of UV-B radiation. Data are presented as mean ± SEM
Parameters |
360 mJ cm−2 (5 min) |
720 mJ cm−2 (10 min) |
D1 diet |
D2 diet |
p-Value |
D1 diet |
D2 diet |
p-Value |
For DNA damage, fifty comets were studied for each replicate, and three replicates were used for each treatment (n = 50 × 3). D1 = vitamin C supplemented diet, D2 = control diet; p – value derived from statistical ‘t’ test (two-tail test); and NS = not significant. |
Survival rate (%) |
84.77 ± 0.15 |
77.5 ± 1.44 |
p < 0.01 |
80 ± 0.58 |
70.23 ± 0.15 |
p < 0.01 |
Average weight (mg) |
257.14 ± 4.54 |
171.69 ± 7.25 |
p < 0.01 |
227.62 ± 6.62 |
139.29 ± 7.96 |
p < 0.01 |
GOT (IU L−1) |
0.11 ± 0.01 |
0.13 ± 0.01 |
NS |
0.12 ± 0.002 |
0.14 ± 0.001 |
p < 0.05 |
GPT (IU L−1) |
0.21 ± 0.004 |
0.26 ± 0.001 |
p < 0.01 |
0.21 ± 0.001 |
0.27 ± 0.001 |
p < 0.01 |
TBARS (mmol MDA per mg tissue) |
13.81 ± 1.41 |
77.28 ± 0.37 |
p < 0.01 |
18.93 ± 0.43 |
97.47 ± 0.74 |
p < 0.01 |
Lysozyme (μg per mg tissue) |
148.0 ± 12.22 |
116.0 ± 4.0 |
NS |
122.0 ± 10.0 |
86.0 ± 1.64 |
NS |
Nitric oxide synthase (mmol per mg tissue) |
73.54 ± 0.23 |
25.85 ± 2.71 |
p < 0.01 |
52.09 ± 3.24 |
24.93 ± 1.43 |
p < 0.01 |
Hsp 70 (pg ml−1) |
631.15 ± 10.88 |
712.03 ± 2.26 |
p < 0.01 |
772.8 ± 4.61 |
848.47 ± 22.14 |
p < 0.05 |
DNA damage
|
(a) Tail DNA |
41.72 ± 1.35 |
52.93 ± 2.34 |
p < 0.01 |
47.77 ± 1.76 |
78.2 ± 3.14 |
p < 0.01 |
(b) Tail extent moment |
6.36 ± 0.27 |
16.75 ± 1.52 |
p < 0.01 |
11.97 ± 1.16 |
37.79 ± 2.36 |
p < 0.01 |
(c) Olive tail moment |
3.38 ± 0.13 |
8.62 ± 0.99 |
p < 0.01 |
5.85 ± 0.63 |
20.93 ± 1.37 |
p < 0.01 |
Biochemical assays
The tissue glutamic oxaloacetic transaminase (GOT) and glutamate pyruvate transaminase (GPT) levels were maximum in the control diet-fed larvae (D210 min) exposed to the dose of 720 mJ cm−2 compared to the other groups. The GPT level was significantly (p < 0.05) lower in the larvae fed with the D1 diet compared to the D2 diet-fed group exposed to the same dose (Table 2). The two-way ANOVA of GOT showed an insignificant interaction as well as the main effect for the diet and UV-B dose, while GPT showed the significant main effect for the diets, but the interaction between the UV-B and diet was insignificant (p > 0.05).
The TBARS level was significantly (p < 0.01) higher in the control diet-fed larvae exposed to two different doses of UV-B radiation compared to the D1 diet-fed larvae. The TBARS level was minimum in the D1 diet-fed larvae exposed to a dose of 360 mJ cm−2. The lysozyme level was maximum in the D1 diet-fed larvae exposed to a dose of 360 mJ cm−2. In both feeding regimes, an inverse relationship was observed between the lysozyme level and the dose of UV-B radiation. A significantly (p < 0.01) higher level of nitric oxide synthase was detected in the D1 diet-fed larvae for both doses compared to the larvae fed with the D2 diet for the respective dose. The highest level was found in the D15 min treatment group. The Hsp 70 level was significantly (p < 0.05) higher in the D2 diet-fed larvae compared to the D1 treatment group. Hsp 70 was minimum in the D15 min group compared to the other groups (Table 2). The two-way ANOVA of TBARS and nitric oxide synthase showed significant interaction as well as the main effect for the diet and UV-B dose. Hsp 70 showed the significant main effect for UV-B dose only and significant interaction between the diet and UV-B dose (p < 0.01).
DNA damage
A direct relationship was found between the dose of UV-B radiation and DNA fragmentation (Fig. 1) in catla larvae. Significantly (p < 0.01) higher scores for tail DNA, tail extent moment and olive tail moment were found in the larvae exposed to a dose of 720 mJ cm−2 compared to the dose of 360 mJ cm−2 for each feeding regime. The DNA damage indices were significantly (p < 0.01) lower in the vitamin C supplemented diet-fed larvae compared to the control group (Table 2). The two-way ANOVA of tail DNA, tail extent moment and olive tail moment showed significant main and interaction effects for the diet and UV-B dose (p < 0.01).
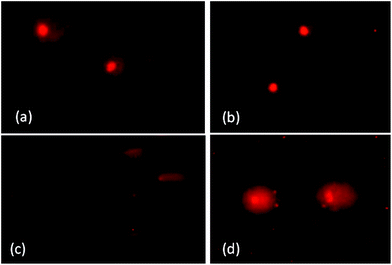 |
| Fig. 1 Pictures showing the DNA damage in the Catla catla larvae fed with vitamin C supplemented (D1) and control diets (D2) and exposed to either 360 or 720 mJ cm−2 UV-B radiation. (a) D2 diet-fed larvae exposed to 360 mJ cm−2 UV-B radiation, (b) D1 diet-fed larvae exposed to 360 mJ cm−2 UV-B radiation, (c) D2 diet-fed larvae exposed to 720 mJ cm−2 UV-B radiation and (d) D1 diet-fed larvae exposed to 720 mJ cm−2 UV-B radiation. Three replicates were used for each treatment and fifty comets for each replicate (n = 50 × 3). | |
Discussion
Exposure to shorter UV-B wavelengths results in higher levels of oxidative stress, degrades the immune system, decreases disease resistance capacity, leads to a higher expression of heat shock proteins and induction of DNA damage in different organisms.5,36–39 Oxidative stress results from the imbalance between the production of reactive oxygen species (ROS) principally from mitochondria and the ROS buffering activity of some enzymes or other antioxidant molecules (tocopherol, ascorbic acid and carotenes among others).40,41 Several studies clearly showed the low survival of pelagic embryos and larvae of temperate marine fishes and tropical freshwater carp larvae exposed to UV-B radiation.15,16,42,43 In the present study, the survival rate of catla larvae showed an inverse relationship with the dose of UV-B. Supplementation of vitamin C in their diet enhanced the survival rate of the catla larvae. Also, UV-B radiation affected the survival rate of the catla larvae.12 Singh et al.15 reported that dietary supplementation of vitamin C and seeds of Achyranthes aspera enhanced the survival rate of UV-B irradiated Labeo rohita, rohu larvae. The lower survival rate of catla exposed to a higher dose of UV-B radiation was the result of the loss of the protective and scavenging capability of the fish against severe oxidative stress. The damage may have been formed following the direct induction of DNA damage. Also, the larvae utilize their energy for survival against radiation, which was probably manifested through slower growth rates.37 A significantly higher average weight was observed in the antioxidant supplemented diet-fed catla larvae. Vitamin C is known as a booster for the immune system and detoxifying agent,25,44 which increases resistance to several bacterial and viral pathogens in fish.45
GOT and GPT are the indicators of liver damage. The UV-B-irradiated larvae showed a higher level of GPT in the present study. A similar result was also observed in an earlier study with catla larvae. The exposure of larvae to UV-B radiation caused higher levels of GOT and GPT compared to that in the control fish.15,16,46 This was due to the increased lipid peroxidation by the UV-B radiation, resulting in the poor condition of the liver. Supplementation of vitamin C in the diet of the catla improved their liver function, which was evident from the reduced levels of GOT and GPT. The feeding of a higher dose of vitamin C reduced the GOT level in Japanese eel, Anguilla japonica47 and sea bream Pagrus major.48
The oxidation of lipids is the most commonly used approach in free radical research because many organisms, especially aquatic ones contain a high amount of lipids with polyunsaturated fatty acid residues, which are substrates for lipid oxidation.49 The products of lipid peroxidation are unstable and categorised as primary, secondary and end products.50,51 The most frequently used method to monitor lipid peroxidation is based on measuring the end products. Among the products, they are represented by malonic dialdehyde (MDA) and 4-hydroxynonenal.52 The UV-B-induced oxidative damage of the cell membranes both in vitro and in vivo were assessed by measuring the formation of lipid peroxidation products such as MDA and TBARS. All cellular components are susceptible to the action of reactive oxygen species; however, biological membranes are most severely affected.38,53,54Girella laevifrons, sea chubs, were exposed to 1, 4 and 5 h of UV-B radiation and lipid peroxidation was maximum after 4 h exposure.29 The application of HPLC and immune techniques is another approaches to measure the end products of lipid peroxidation.55 TBARS was significantly lower in the vitamin C supplemented diet-fed larvae exposed at both doses of UV-B. Vitamin C, the major antioxidant additive used in the food industry, reduces the oxidative stress in animals.25,48,56,57 A lower level of TBARS was found in the vitamin C supplemented diet-fed rohu larvae.15
Lysozyme activity is an important index of innate immunity in fish and is ubiquitous in its distribution among living organisms,58 which varies depending on the degree of stress and infection. The lowest lysozyme activity was recorded in the larvae exposed to a higher dose of UV-B radiation. However, the lysozyme activity increased in the antioxidant supplemented diet-fed catla. This confirmed the role of vitamin C as first line of natural antioxidant defence against environmental stress, such as UV-B radiation.
Nitric oxide synthase activity regulates the production of nitric oxide. Nitric oxide functions as an intracellular messenger to stimulate guanylate cyclase.59 It induces biological effects by directly activating many intercellular molecules.60 The inducible isoform iNOS produces a large amount of nitric oxide as a defence mechanism. Feeding larvae with vitamin C supplemented diets enhances their nitric oxide synthase level. In UV-B irradiated rohu larvae, the dietary supplementation of vitamin C enhanced the nitric oxide synthase level compared the control diet-fed larvae.15
Animals can survive in adverse conditions through several ways, and one well-characterized mechanism is by the induction of stress proteins such as heat shock proteins. These proteins are synthesized constitutively in cells and are induced after exposure to stress including heat, cold, infections and diseases.61 Stress proteins are being considered for their potential role as sensitive markers of cell injury since they are related with the immune response and autoimmune diseases.62 In the present study, UV-B radiation induced the expression of Hsp 70. A Similar result was also observed in UV-B irradiated pike (Esox lucius) embryos, whose level of Hsp/Hsc 70 was found to be higher after 3 h of exposure.19 Many studies also reported that an increase in the level of Hsp 70 may be associated with reduced individual fitness.63–66 This was confirmed in the present study, where a higher level of Hsp 70 was found in the control diet-fed larvae. This resulted in poor survival, growth and physiological responses in the larvae.
DNA damage can be caused directly by exposure to UV radiation or indirectly through the production of ROS.37,67 Reactive oxygen species oxidize the bases, leading to DNA breaks.6,68 DNA damage can lead to the expression of p53
69 chromosomal fragmentation6 and apoptosis or cellular necrosis in many organisms. Although the comet assay is a useful tool for the study of DNA damage, it has some limitations. It depends on the tissue, organism, damage causing agent, etc. Moreover, the effect on cell-cycle checkpoints is not identifiable with this assay.70 The vitamin C supplemented diet provided efficient repair mechanisms through the antioxidant potency in the larvae. The α-tocopherol (vitamin E) present in the supplied diet (as vitamin-mineral premix) may act with vitamin C to prevent the formation of UV-B-induced CPD.71 Also, the water-soluble antioxidant vitamin C can reduce tocopheroxyl radicals directly or indirectly and thus, supports the antioxidant activity of vitamin E.72
Many studies showed that vitamin C has the ability to regenerate and/or spare vitamin E in fish, such as in Atlantic salmon, Salmo salar,73 yellow perch, Perca flavescens74 and red sea bream, Pagrus major.25,75 The results of the comet assay reflected the increase in CPD repairing processes, while there was a decrease in the p53 protein level. An earlier study showed that vitamin E inhibits the induction of CPD and oxidatively generates DNA damage.71 Hence, it reduced the DNA damage even at higher dose of UV-B radiation. A higher content of CDP dimers was recorded in UV-B irradiated Gadus morhua, Atlantic cod,36 and Strongylocentrotus droebachiensis, sea urchin.37 Vehniainen et al.19 reported the induction of CPDs in Northern pike exposed at the highest fluence rate of 300 and 540 mW m−2. In sea chubs, a direct relationship was found between the duration of exposure and their tail moment.39
Conclusions
The dietary supplementation of the antioxidant vitamin C provides protection against stress induced by UV-B radiation in catla larvae in culture conditions. This was evident from the significant interaction observed between the diets and UV-B doses for TBARS, nitric oxide synthase, Hsp 70 and DNA damage. Vitamin C modulated the immune system and resulted in a higher survival and average weight in the larvae. This useful information helps to provide protection to the aquacultural species in the present scenario of climate change.
Contribution of authors
RC and JGS have planned and designed the experiment. They helped MKS during experiment. MKS conducted the experiment, prepared graphs. RC has played major role in the analysis of data and manuscript preparation. PM has assisted in statistical analysis of data and related interpretation.
Conflicts of interest
The authors declare no competing financial interests.
Acknowledgements
We are grateful to Department of Biotechnology, DBT, New Delhi for financial support in the form of project to RC. KMS is thankful to the University of Delhi for providing him UGC Science Meritorious Research Fellowship.
References
- K. D. Fausch, C. E. Torgersen, C. V. Baxter and H. W. Li, Landscapes to riverscapes: bridging the gap between research and conservation of stream fishes, Bioscience, 2012, 52, 483–498 CrossRef.
- L. Behrendt, M. E. Jönsson, J. V. Goldstonea and J. J. Stegeman, Induction of cytochrome P450 1 genes and stress response genes in developing zebrafish exposed to ultraviolet radiation, Aquat. Toxicol., 2010, 98, 74–82 CrossRef CAS PubMed.
- M. Tedetti, High penetration of ultraviolet radiation in the south east Pacific waters, Geophys. Res. Lett., 2007, 34, 1–5 CrossRef.
- A. H. Sayed, A. T. Ibrahim, I. A. A. Mekkawy and U. M. Mahmoud, Acute effects of ultraviolet-A on some biochemical and histological parameters of African catfish C. gariepinus (Burchell, 1822), Photochem. Photobiol., 2007, 89, 170–174 CrossRef CAS PubMed.
- J. Cadet, E. Sage and T. Douki, Ultraviolet radiation-mediated damage to cellular DNA, Mutat. Res., 2005, 571, 3–17 CrossRef CAS PubMed.
- L. G. Schaumburg, G. L. Polettaa, A. Imhof and P. A. Siroski, Ultraviolet radiation-induced genotoxic effects in the broad-snouted caiman, Caiman latirostris, Mutat. Res., 2010, 700, 67–70 CAS.
- S. Courdavault,
et al., Repair of the three main types of bipyrimidine DNA photoproducts in human keratinocytes exposed to UVB and UVA radiations, DNA Repair, 2005, 4, 836–844 CrossRef CAS PubMed.
-
A. Blaustein and N. Sengsavanh, Ultraviolet Radiation, in Encyclopedia of Biodiversity, Academic Press, Waltham, MA, 2001 Search PubMed.
- A. A. Vink and L. Roza, Biological consequences of cyclobutane pyrimidine dimmers, J. Photochem. Photobiol., B, 2001, 65, 101–104 CrossRef CAS.
- R. J. Hunter, J. H. Taylor and H. G. Moser, Effects of ultraviolet irradiation on eggs and larvae of northern anchovy, Engraulis mordax, and the Pacific mackerel, Scomber japonicus, during embryonic stage, Photochem. Photobiol., 1979, 29, 325–338 CrossRef PubMed.
- J. G. Sharma, R. Masuda and R. M. Tanaka, Orientation behaviour of Pagrus major, larvae exposed to UV-B radiation in laboratory condition, Int. J. Radiat. Biol., 2007, 83, 49–52 CrossRef CAS PubMed.
- J. G. Sharma, P. Mittal and R. Chakrabarti, Development of survivorship model for UV-B irradiated Catla catla larvae, Aquat. Ecol., 2008, 42, 17–23 CrossRef.
- E. S. Markkula, H. M. Salo, A. K. Rikalainen and E. L. Jokinen, Long-term UVB irradiation affects the immune functions of carp (Cyprinus carpio) and rainbow trout (Oncorhynchus mykiss), Photochem. Photobiol., 2009, 85, 347–352 CrossRef CAS PubMed.
- J. G. Sharma, Y. V. Rao, S. Kumar and R. Chakrabarti, Impact of UV-B radiation on the digestive enzymes and immune system of larvae of Indian major carp Catla catla, Int. J. Radiat. Biol., 2010, 3, 181–186 CrossRef PubMed.
- M. K. Singh, J. G. Sharma and R. Chakrabarti, Impact of UV-B radiation on the physiology of freshwater carp Labeo rohita larvae and evaluation of UV-B protective properties of seeds of Achyranthes aspera and vitamin C, Agric. Res., 2013, 2, 166–171 CrossRef CAS.
- M. K. Singh, J. G. Sharma and R. Chakrabarti, Effect of UV-B radiation on the defence system of Labeo rohita (Actinopterygii: Cypriniformes: Cyprinidae) larvae and its modulation by seed of Devil's Horsewhip, Achyranthes aspera, Acta Ichthyol. Piscatoria, 2013, 43, 119–126 CrossRef.
- R. A. Charron, J. C. Fenwich, D. R. S. Lean and T. W. Moon, Ultraviolet-B radiation effects on antioxidant status and survival in the zebra fish Brachydanio rerio, Photochem. Photobiol., 2000, 72, 327–333 CrossRef CAS PubMed.
- B. M. Sanders, Stress proteins in aquatic organisms: an environmental perspective, Crit. Rev. Toxicol., 1993, 23, 49–75 CrossRef CAS PubMed.
- E. R. Vehniainen, K. Vahakangas and A. O. J. Oikari, UV-B exposure causes DNA damages and changes in protein expression in Northern pike (Esox lucius) posthatched embryo, Photochem. Photobiol., 2012, 88, 363–370 CrossRef CAS PubMed.
- F. U. Hartl, Molecular chaperones in cellular protein folding, Nature, 1996, 381, 571–579 CrossRef CAS PubMed.
- B. Bukau and A. L. Horwich, The Hsp70 and Hsp60 chaperone machines, Cell, 1998, 92, 351–366 CrossRef CAS PubMed.
-
Food and Agricultural Organization, FAO, The state of the world fisheries and aquaculture, FAO fisheries and aquaculture department, Food and Agriculture Organization of the United Nations (FAO), Rome, Italy, 2004 Search PubMed.
- M. K. Singh, J. G. Sharma and R. Chakrabarti, Simulation study of natural UV-B radiation on Catla catla and its impact on physiology, oxidative stress, Hsp 70 and DNA fragmentation, J. Photochem. Photobiol., B, 2015, 149, 156–163 CrossRef CAS PubMed.
-
J. Gabaudan and V. Verlhac, Critical review of the requirements of ascorbic acid in cold and cool water fishes (salmonids, percids, plecoglossids, and flatfishes), in Ascorbic acid in aquatic organisms, ed. K. Dabrowski, CRC Press LLC, U.S.A., 2001, pp. 33–48 Search PubMed.
- J. Gao, S. Koshio, M. Ishikawa, S. Yokoyama and R. E. P. Mamauag, Interactive effects of vitamin C and E supplementation on growth performance, fatty acid composition and reduction of oxidative stress in juvenile Japanese flounder Paralichthys olivaceus fed dietary oxidized fish oil, Aquaculture, 2014, 422–423, 4–90 Search PubMed.
- K. Dabrowski, Ascorbic acid status in the early life of whitefish (Coregonus lavaretus L.), Aquaculture, 1990, 84, 61–70 CrossRef CAS.
- K. S. Wang and T. C. Chai, Reduction in omega-3-fatty acids by UV-B irradiation in microalgae, J. Appl. Phycol., 1994, 6, 415–421 CrossRef CAS.
- S. Nahon, V. A. C. Porras, A. M. Pruski and F. Charles, Sensitivity to UV radiation in early life stages of the Mediterranean sea urchin Sphaerechinus granularis (Lamarck), Sci. Total Environ., 2009, 407, 1892–1900 CrossRef CAS PubMed.
- O. H. Lowry, N. J. Rosebrough and A. R. Farr, Protein measurement with the folin phenol reagent, J. Biol. Chem., 1951, 193, 265–275 CAS.
- H. Ohkawa, N. Ohishi and K. Yagi, Assay for lipid peroxides in animal tissues by thiobarbituric acid reaction, Anal. Biochem., 1979, 95, 351–358 CrossRef CAS PubMed.
- A. K. Siwicki, Immunostimulating influence of levamisole on nonspecific immunity in carp (Cyprinus carpio), Dev. Comp. Immunol., 1989, 13, 87–91 CrossRef CAS PubMed.
- D. U. Lee, Y. J. Kang and M. K. Park, Effects of 13-alkyl-substituted berberine alkaloids on the expression of COX-II, TNF-α, iNOS and IL-12 production in LPS stimulated macrophages, Life Sci., 2003, 73, 1401–1412 CrossRef CAS PubMed.
- S. Chandna, Single-cell gel electrophoresis assay monitors precise kinetics of DNA fragmentation induced during programmed cell death, Cytometry, Part A, 2004, 61, 127–133 CrossRef PubMed.
- O. Osting and K. Johanson, Microelectrophorectic study of radiation induced DNA damages in individual mammalian cells, Biochem. Biophys. Res. Commun., 1984, 123, 291–298 CrossRef.
- N. P. Singh, M. T. McCoy, R. R. Tice and E. L. Schneider, A simple technique for quantitation of low levels of DNA damage in individual cells, Exp. Cell Res., 1988, 175, 184–191 CrossRef CAS PubMed.
- M. P. Lesser, J. H. Farrel and C. W. Walker, Oxidative stress and p53 expression in the larvae of Atlantic cod (Gadus morhua) exposed to ultraviolet (290-400 nm) radiation, J. Exp. Biol., 2001, 204, 157–164 CAS.
- M. P. Lesser and T. M. Barry, Survivorship, development, and DNA damage in echinoderm embryos and larvae exposed to ultraviolet radiation (290-400 nm), J. Exp. Mar. Biol. Ecol., 2003, 292, 75–91 CrossRef CAS.
- V. A. Terra,
et al., Time-dependent reactive species formation and oxidative stress damage in the skin after UVB irradiation, J. Photochem. Photobiol., B, 2012, 109, 34–41 CrossRef CAS PubMed.
- A. Carrasco-Malio,
et al., Are the intertidal fish highly resistant to UV-B radiation? A study based on oxidative stress in Girella laevifrons (Kyphosidae), Ecotoxicol. Environ. Saf., 2014, 100, 93–98 CrossRef CAS PubMed.
- L. Ligouri,
et al., Oxidative stress, aging, and diseases, Clin. Interventions Aging, 2018, 13, 757–772 CrossRef PubMed.
- P. Blier, Fish Health: an oxidative stress perspective, Fish. Aquacult. J., 2014, 5, 1 Search PubMed.
- R. D. Vetter, A. Kurtzman and T. Mori, Diel cycles of DNA damage and repair in eggs and larvae of Northern anchovy, Engraulix mordax, exposed to solar ultraviolet radiation, Photochem. Photobiol., 1999, 69, 27–33 CrossRef CAS.
- J. G. Sharma and R. Chakrabarti, Effects of UV-B radiation on the gills of Catla catla during early developmental stage, Toxicol. Environ. Chem., 2006, 88, 367–371 CrossRef CAS.
- K. Anbarasu and M. R. Chandran, Effect of ascorbic acid on the immune response of the catfish, Mystus gulio (Hamilton), to different bacterins of Aeromonas hydrophila, Fish Shellfish Immunol., 2001, 11, 347–355 CrossRef CAS PubMed.
-
S. P. Lall, Nutrition and Health of Fish, in Advances en Nutrición Acuícola, V Memorias del V Simposium Internacional de Nutrición Acuícola, ed. L. E. Cruz-Suárez, D. Ricque-Marie, M. Tapia-Salazar, M. A. Olvera-Novoa and R. Civera-Cerecedo, Mérida, Yucatán, Mexico, 2000, pp. 19–22 Search PubMed.
- J. G. Sharma, M. K. Singh and R. Chakrabarti, Physiological responses of Catla catla larvae fed with Achyranthes aspera seed enriched diet and exposed to UV-B radiation, Indian J. Biochem. Biophys., 2015, 52, 155–160 CAS.
- T. Ren,
et al., Influence of dietary vitamin C and bovine lactoferrin on blood chemistry and nonspecific immune responses of Japanese eel, Anguilla japonica, Aquaculture, 2007, 267, 31–37 CrossRef CAS.
- J. Gao,
et al., Effect of dietary oxidized fish oil and vitamin C supplementation on growth performance and reduction of oxidative stress in red sea bream Pagrus major, Aquacult. Nutr., 2013, 19, 35–44 CrossRef.
- O. I. Kubrak,
et al., Cobalt-induced oxidative stress in brain, liver and kidney of goldfish Carassius auratus, Chemosphere, 2011, 85, 983–989 CrossRef CAS PubMed.
- V. I. Lushchak and T. V. Bagnyukova, Hypoxia induces oxidative stress in tissues of a goby fish, the rotan Percottus glenii, Comp. Biochem. Physiol., Part B: Biochem. Mol. Biol., 2007, 148, 390–397 CrossRef PubMed.
- V. I. Lushchak, Environmentally induced oxidative stress in aquatic animals, Aquat. Toxicol., 2011, 101, 13–30 CrossRef CAS PubMed.
- D. R. Janero, Malondialdehyde and thiobarbituric acid-reactivity as diagnostic indices of lipid peroxidation and peroxidative tissue injury, Free Radicals Biol. Med., 1990, 9, 515–540 CrossRef CAS PubMed.
- G. Aldini,
et al., Effects of UVB radiation on 4-hydroxy-2-trans-nonenal metabolism and toxicity in human keratinocytes, Chem. Res. Toxicol., 2007, 20, 416–423 Search PubMed.
- W. Eiberger,
et al., Oxidative stress impairs the repair of oxidative DNA base modifications in human skin fibroblasts and melanoma cells, DNA Repair, 2008, 7, 912–921 CrossRef CAS PubMed.
- K. Claeson, G. Thorsen and B. Karlberg, Methyl malondialdehyde as an internal standard for the determination of malondialdehyde, J. Chromatogr. B: Biomed. Sci. Appl., 2001, 751, 315–323 CrossRef CAS.
- D. R. Tocher,
et al., Comparative study of antioxidant defence mechanisms in marine fish fed variable levels of oxidised oil and vitamin E, Aquacult. Int., 2003, 11, 195–216 CrossRef CAS.
- J. Gao,
et al., Effects of dietary oxidized fish oil with vitamin E supplementation on growth performance and reduction of lipid peroxidation in tissues and blood of red sea bream Pagrus major, Aquaculture, 2012, 356–357, 73–79 CrossRef CAS.
- S. Saurabh and P. K. Sahoo, Lysozyme: an important defence molecule of fish innate immune system, Aquacult. Res., 2008, 39, 223–239 CrossRef CAS.
- L. Rochette,
et al., Nitric oxide synthase inhibition and oxidative stress in cardiovascular diseases: possible therapeutic targets?, Pharmacol. Ther., 2013, 140, 239–257 CrossRef CAS PubMed.
- M. A. Vargas,
et al., Participation of nitric oxide in the color change induced by UV-B radiation in the crab Chasmagnathus granulates, Pigm. Cell Melanoma Res., 2008, 2, 184–191 CrossRef PubMed.
- Y. Y. Sung, C. Pineda, T. H. MacRae, P. Sorgeloos and P. Bossier, Exposure of gnotobiotic Artemia fransiscana larvae to abiotic stress promotes heat shock protein 70 synthesis and enhances resistance to pathogenic Vibrio campbellii, Cell Stress Chaperones, 2008, 13, 59–66 CrossRef CAS PubMed.
- R. J. Roberts, C. Agius, C. Saliba, P. Bossier and Y. Y. Sung, Heat shock proteins (chaperones) in fish and shellfish and their potential role in relation to fish health: a review, J. Fish Dis., 2010, 33, 789–801 CrossRef CAS PubMed.
- M. E. Feder, N. Blair and H. Figueras, Natural thermal stress and heat-shock protein expression in Drosophila larvae and pupae, Funct. Ecol., 1997, 11, 90–100 CrossRef.
- R. Silbermann and M. Tatar, Reproductive costs of heat shock protein in transgenic Drosophila melanogaster, Evol. Ecol., 2000, 54, 2038–2045 CAS.
- J. G. Sorensen, T. N. Kristensen and V. Loeschcke, The evolutionary and ecological role of heat shock proteins, Ecol. Lett., 2003, 6, 1025–1037 CrossRef.
- M. Tine, F. Bonhomme, D. J. Mckenzie and J. D. Durand, Differential expression of the heat shock protein Hsp70 in natural populations of the tilapia, Sarotherodon melanotheron, acclimatised to a range of environmental salinities, BMC Ecol., 2010, 10, 1–8 CrossRef PubMed.
- M. J. Peak and J. G. Peak, Hydroxyl radical quenching agents protect against DNA breakage caused by both 365 nm and gamma radiation, Photochem. Photobiol., 1990, 51, 649–652 CrossRef CAS PubMed.
- D. P. Häder, H. D. Kumar, R. C. Smith and R. C. Worrest, Effects of solar UV radiation on aquatic ecosystems and interactions with climate change, Photochem. Photobiol. Sci., 2007, 6, 267–285 RSC.
- J. Renzing, S. Hansen and D. P. Lane, Oxidative stress is involved in the UV activation of p53, J. Cell Sci., 1996, 109, 1105–1112 CAS.
- L. Wenjuan, A. M. Michael and Z. Wei-Guo, The comet assay: a sensitive method for detecting DNA damage in individual cells, Methods, 2009, 48, 46–53 CrossRef PubMed.
- G. J. Delinasios, M. Karbaschi, M. S. Cooke and A. R. Young, Vitamin E inhibits the UVAI induction of “light” and “dark” cyclobutane pyrimidine dimers, and oxidatively generated DNA damage, in keratinocytes, Sci. Rep., 2018, 8, 423–235 CrossRef PubMed.
- H. Sies, W. Stahl and A. R. Sundquist, Antioxidant functions of vitamins: vitamins E and C, beta-carotene, and other carotenoids, Ann. N. Y. Acad. Sci., 1992, 30, 7–20 CrossRef.
- K. Hamre, R. Waagbo, R. K. Berge and O. Lie, Vitamins C and E interact in juvenile Atlantic salmon (Salmo salar, L), Free Radicals Biol. Med., 1997, 22, 137–149 CrossRef CAS PubMed.
- K. J. Lee and K. Dabrowski, Interaction between vitamins C and E affects their tissue concentrations, growth, lipid oxidation, and deficiency symptoms in yellow perch (Perca flavescens), Br. J. Nutr., 2003, 89, 589–596 CrossRef CAS PubMed.
- J. Gao,
et al., Interaction between vitamin C and E on oxidative stress in tissues and blood of red sea bream (Pagrus major), Aquacult. Sci., 2012, 60, 119–126 CAS.
|
This journal is © The Royal Society of Chemistry and Owner Societies 2019 |
Click here to see how this site uses Cookies. View our privacy policy here.