DOI:
10.1039/C8QM00510A
(Research Article)
Mater. Chem. Front., 2019,
3, 216-223
Encapsulation of pentazole gold nanoparticles into modified polycyanostyrene and polynitrostyrene microspheres as efficient catalysts for cinnoline synthesis and hydration reaction†
Received
7th October 2018
, Accepted 3rd December 2018
First published on 5th December 2018
Abstract
Modified polycyanostyrene and polynitrostyrene microspheres were designed and synthesized, which proved to be an effective carrier to prepare gold nanoparticles with pentazole gold as a catalyst precursor. The developed gold nanoparticles were characterized using scanning electron microscopy, transmission electron microscopy, energy dispersive X-ray, X-ray power diffraction and X-ray photoelectron spectrometry. In addition, it was observed that this catalytic system successfully achieved application in the synthesis of cinnoline derivatives and hydration reactions in high yields. Mechanism investigation was conducted to better understand these transformations and catalyst systems. To the best of our knowledge, this is the first example of utilizing pentazole gold as the catalyst precursor and achieving catalytic applications.
Introduction
Polymer nanocomposites have been an interesting and useful topic of current research and attracted a great deal of attention during the past several years for broad applications in sensors, high-performance composites, electronic devices and catalytic synthesis.1 It is considered that polymer nanocomposites are particularly fascinating materials because polystyrene (PS) polymers usually have elegent electrical, thermal and mechanical properties compared to many conventional composites.2 Importantly, polystyrene polymers can be conveniently prepared to form excellent monodisperse spheres from the micrometer to nanometer size range.3 Despite all the mentioned achievements, the improvement and modification of polystyrene polymers is still a fascinating field and it remains an interesting task to develop a highly effective method to show the performance and characteristics of polystyrene polymers.4
During the past several decades, gold catalysis has gained tremendous interest and is still considered to be one of the most promising research areas in organic chemistry.5,6 Compared to gold(I) and gold(III) catalysis, gold(0) catalysis (gold nanoparticles) is more adjustable and promising but the reactions are relatively limited and less developed.7,8 Recently, Shi and our colleagues disclosed that triazole could be used as an effective ligand to coordinate with gold, thus revealing good stability with excellent catalytic activities.9 In addition, triazoles have found another use as counter anions in gold catalysis and highlighted the application of triazoles.10 As a result of the continuing effort to expand more bulky ligands11 and catalytic applications,12 pentazole was selected as a ligand to coordinate with gold for the synthesis of new skeleton gold complexes. We next expand the application of gold nanoparticles with pentazole gold as the catalyst precursors. In the beginning, we found that pentazole gold complexes could be synthesized in high yield. However, it was found that the pentazole gold complex revealed much lower stability than triazole gold and gold black observed when pentazole gold was used to catalyze cinnoline synthesis. Interestingly, this gold black still exhibited some catalytic activity. With this result in hand, one idea was aroused: if pentazole gold was used as a catalyst precursor for supported catalysts, what about the catalytic activity of this synthesized catalytic system.
Based on these considerations, herein, we report the synthesis of modified polycyanostyrene (PCS) microspheres with cyanostyrene as the staring material, which was characterized using scanning electron microscopy (SEM) and transmission electron microscopy (TEM). Next, the synthesized pentazole gold complex was supported on these modified polycyanostyrene microspheres (Scheme 1). The gold nanoparticles supported by modified polycyanostyrene microspheres were characterized using scanning electron microscopy, transmission electron microscopy, energy dispersive X-ray, X-ray power diffraction and X-ray photoelectron spectrometry.
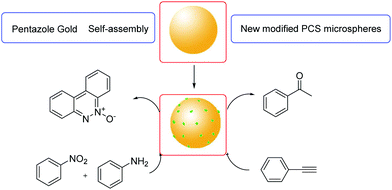 |
| Scheme 1 Encapsulation of gold nanoparticles into modified polycyanostyrene microspheres with pentazole gold complexes as the precursor. | |
Results and discussion
Synthesis of modified polycyanostyrene microspheres
PVP (1.400 g) and azoisobutyronitrile (0.100 g) were dissolved in isopropanol (80 mL), and the obtained mixture was heated up to 70 °C in a Schlenk tube, and simultaneously aerated with nitrogen to eliminate the effect of oxygen (Scheme 2). Subsequently, 4-cyanostyrene (10.0 g) was added into the above-mentioned mixture under vigorous mechanical stirring. The polymerization proceeded for 24 h at room temperature under stirring conditions. Finally, the resultant modified polycyanostyrene microspheres (PCS) were collected by centrifugation, and washed with water and ethanol several times, followed by vacuum freeze-drying for 12 h.
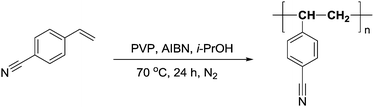 |
| Scheme 2 The synthesis of modified polycyanostyrene microspheres. | |
Synthesis of pentazole gold supported by modified polycyanostyrene microspheres
PPh3AuCl (1.0 mmol) and AgOTf (1.1 mmol) were dissolved in CH2Cl2 (5 mL) at room temperature. The solution was stirred for 5 min, and pentazole (1.0 mmol) was added to the above mixture. Then the mixture was stirred for 5 hours at room temperature. The following cloudy solution was filtered through a short pad of celite and washed with CH2Cl2 several times, and the clear solution was concentrated under vacuum. After this, petroleum ether was added slowly drop-wise on top of the DCM layer. The resultant purple solid was collected and washed with petroleum ether several times, followed by being dried by a vacuum pump.
Modified polycyanostyrene (PCS) microspheres (1.000 g) were dispersed in ethanol (100 mL) under ultrasonication, and pentazole gold (50 mg) was dissolved in ethanol (20 mL), and added to the above disperson liquid at 78 °C. After 12 hours, the reaction was cooled to room temperature. The composite (5% loading, w/w) was collected by centrifugation, and washed with ethanol several times, followed by vacuum freeze-drying for 12 h.
Characterization of modified polycyanostyrene microspheres
After the synthesis of these modified polycyanostyrene microspheres (PCS), the characterization of the PCS was carried out. SEM and TEM images revealed that the particle size of the polycyanostyrene microspheres was obviously smaller than that of PS microspheres. It was observed that specific surface area was enhanced from BET characterization (see ESI†).
Characterization of pentazole gold supported by modified polycyanostyrene microspheres
This pentazole gold supported by modified polycyanostyrene microspheres (PA-Au–PCS) was characterized through scanning electron microscopy (SEM), energy dispersive X-ray (EDX), X-ray power diffraction (XRD), transmission electron microscopy (TEM) and X-ray photoelectron spectrometry (XPS). SEM and TEM images of Fig. 1 revealed that gold nanoparticles were supported on modified polycyanostyrene microspheres (PCS). The morphologies and structural characteristics of the admixture could be observed diametrically from scanning electron microscopy and transmission electron microscopy. As delineated in Fig. 1, it was clearly observed that the surface of the modified polycyanostyrene microspheres had lots of gold particles. The EDS images revealed that the peak at 2.12 keV and 9.7 keV demonstrated that metallic gold nanoparticles have been presented in the PCS (Fig. 2).
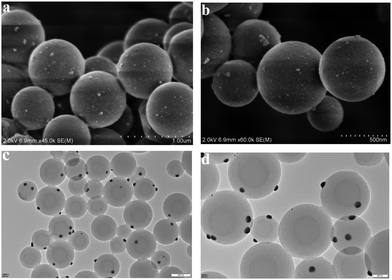 |
| Fig. 1 SEM images of (a and b) PA-Au–PCS; TEM images of (c and d) PA-Au–PCS. | |
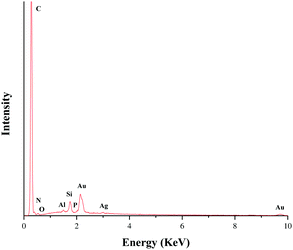 |
| Fig. 2 EDS images of PA-Au–PCS. | |
Fig. 3 shows the X-ray diffraction patterns of these modified polycyanostyrene microspheres and PA-Au–PCS. The wide-angle XRD pattern of the PA-Au–PCS revealed that peaks at 2θ = 38.2°, 44.4°, 64.6° and 77.6° were assigned to the (1 1 1), (2 0 0), (2 2 0) and (3 1 1) crystal planes, which confirmed that the Au nanoparticles had been successfully supported on the modified polycyanostyrene microspheres.
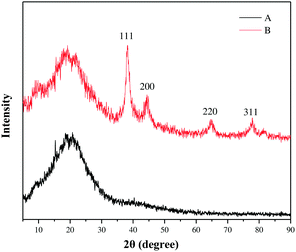 |
| Fig. 3 XRD pattern of (A) PCS, and (B) PA-Au–PCS. | |
The chemical compositions and surface chemical states of the PA-Au–PCS composites were also proved by XPS. The peaks of the identical XPS wide-scan spectra revealed that C, O, N, Si and Au chemical elements could be clearly observed (Fig. 4a). The inset of Fig. 4b indicated that the binding energies at 83.25 and 86.86 eV belonged to Au0.
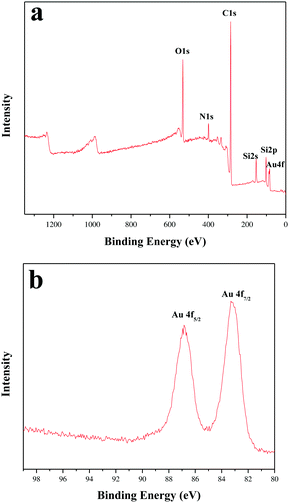 |
| Fig. 4 (a) XPS wide-scan spectrum of PA-Au–PCS. (b) High-resolution spectrum of Au. | |
Catalytic activity
Cinnoline derivatives represent useful and important units of heterocycles, and have gained much attention due to their biological activities, such as antifungal, antimalarial, antihyperglycemic, cytotoxic, antiparasitic, anti-inflammatory and antibacterial. However, the synthesis of the cinnoline derivatives was less studied. Although several examples were developed, the reactions were usually carried out with harsh conditions, like high temperature, sealed flask, absolute anhydrous solvent and strong bases etc.13 In addition, the yields were not satisfactory to industrial requirements and no system research was described. With this PA-Au–PCS catalyst in hand, the cinnoline synthesis was used to examine this catalyst. So, the reaction of nitrobenzene (1a) with aniline (2a) was chosen as a model reaction to try in the presence of a PA-Au–PCS catalyst. As the description of Table 1 showed, solvents and bases were screened to obtain the best reaction conditions. Only trace desired product (3a) was achieved with sodium carbonate as a base (Table 1, entries 2 and 3); similarly, the reaction with K2CO3 afforded the product (3a) in trace yields (entries 6 and 7). However, the base KOH could produce good yields of 75% and 68% respectively (entries 1 and 4). The product (3a) was separated in a better yield (85%) with tBuOK as a base (entry 5). Next, various solvents were tried on the reaction with tBuOK (entries 8–14). Most of the reactions proceeded well in moderate to good yields except DMF and CH3CN as the solvent. In addition to this, trace product (3a) was obtained with cesium carbonate (entry 15). After screening a series of conditions, it was found that tBuOK was the best base in toluene under reflux conditions. It should be noted that only moderate yield was obtained with PPh3AuCl as a catalyst, which indicated that the modified polycyanostyrene microspheres were effective carriers to prepare gold nanoparticles with pentazole gold as a catalyst precursor and revealed much higher catalytic activity than homogeneous catalyst PPh3AuCl.
Table 1 Optimization of the reaction conditionsab
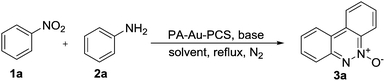
|
Entry |
Base |
Solvent |
Yieldb [%] |
Reagents and conditions: 1a (1.0 mmol), 2a (1.0 mmol), catalyst (5 wt%, 1 mol%), base (1.0 mmol), solvent (3.0 mL), 3 h, reflux.
Yields of isolated product.
PPh3AuCl was used.
|
1 |
KOH |
Toluene |
75 |
2 |
Na2CO3 |
Toluene |
Trace |
3 |
Na2CO3 |
Xylene |
Trace |
4 |
KOH |
Xylene |
68 |
5 |
tBuOK |
Toluene |
85 |
6 |
K2CO3 |
Toluene |
Trace |
7 |
K2CO3 |
Xylene |
Trace |
8 |
tBuOK |
Xylene |
78 |
9 |
tBuOK |
n-Hexane |
74 |
10 |
tBuOK |
1,4-Dioxane |
81 |
11 |
tBuOK |
THF |
76 |
12 |
tBuOK |
DMF |
Trace |
13 |
tBuOK |
CH3CN |
Trace |
14 |
tBuOK |
tert-Amyl alcohol |
71 |
15 |
Cs2CO3 |
Toluene |
Trace |
16 |
tBuOK |
Toluene |
52c |
Having established the optimal conditions, we explored the scope of the PA-Au–PCS catalyzed coupling reaction and the results are shown in Table 2. Generally, this reaction could be proceeded smoothly and afford the desired products in moderate to high yields. We could find that the functional groups in diverse substrates were all well tolerated, such as fluoro, chloro, bromine, methyl, methoxy, iso-propyl and tert-butyl. Meanwhile, it was revealed that the tert-butyl substrate afforded lower yields than other electron-donating moieties, such as 3ap (70%), 3aq (68%) and 3ar (62%). Notably, it was observed that the substrates with electron-donating groups gave slightly lower yields.
Table 2 Substrate scope experiments of aromatic amine and nitrobenzene derivativesab
Conditions: 1a (1.0 mmol), 2a (1.0 mmol), catalyst (5 wt%, 1 mol%), tBuOK (1.0 mmol), toluene (3.0 mL), 3 h, reflux.
Isolated yields based on 1a.
|
|
Additionally, we attempted to catalyze the hydration of alkynes with the newly developed PA-Au–PCS as a catalyst. As shown in Table 3, almost all the substrates could be suitable for this transformation in good to high yields. Several substrates with important functional groups were also tolerated, including chloro, fluoro, bromo and other alkyl substituents, at different positions of the moiety of the aryl groups. Furthermore, good yields were achieved for the substrates with methoxy and naphthalene groups. Interestingly, the substrates with some non-benzene ring structures could also work well and afford high yield such as n-amyl (5ao) and thienyl (5ap).
Table 3 PA-Au–PCS catalyzed hydration of alkynesab
Conditions: 4a (1.0 mmol), AgOTf (5 mol%), catalyst (5 wt%, 1 mol%), HOAc/H2O (10 : 1 = v : v, 4 mL), 12 h, 100 °C.
Isolated yields based on 4a.
PPh3AuCl was used.
|
|
Mechanism exploration
Catalyst exploration
This gold supported by modified polycyanostyrene microspheres (PA-Au–PCS) revealed good catalytic activity in these two transformations, which indicated that the supposed idea was feasible. (1) One important reason is the successful synthesis of these modified polycyanostyrene microspheres, which provided different morphologies compared with the classical PS microspheres from transmission electron microscopy. The most possible factor might be from the introduction of high electron-withdrawing cyano groups in the modified microspheres, which thus led to high catalytic activity. To better explain this, we designed another modified microsphere with an electron-donating group, which was synthesized in this context after several attempts. However, the polymethoxylstyrene microspheres failed to be synthesized with a similar method and only polybromostyrene microspheres (PBS) were obtained after some attempts, which suggested that modified microspheres with an electron-donating group might not be appropriate (Scheme 3). Meanwhile, polynitrostyrene microspheres (PNS) were synthesized to further test this.
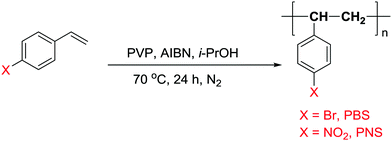 |
| Scheme 3 The synthesis of modified polybromostyrene (PBS) and polynitrostyrene (PNS) microspheres. | |
For these synthesized polybromostyrene (PBS) and polynitrostyrene (PNS) microspheres, different morphologies were also found compared to classical PS microspheres and polycyanostyrene microspheres. It was observed that pentazole gold was supported on these modified polybromostyrene microspheres, which showed low catalytic activity for the hydration of alkynes with several substrates showing no conversion (Scheme 4). To better illustrate this, the corresponding experiments with PA-Au@PNS as the catalyst revealed that high yields of the products were achieved, which further suggested that modified microspheres with an electron-withdrawing group might be appropriate for this transformation.
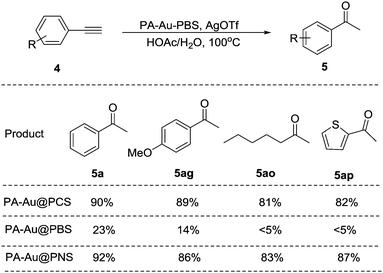 |
| Scheme 4 The comparison of the catalysts PA-Au@PCS and PA-Au@PBS in the hydration of alkyne. | |
(2) Another factor that couldn’t be ignored was the introduction of pentazole gold. When pentazole gold(I) was used to catalyze cinnoline synthesis in the homogeneous state, pentazole gold was found to decompose and gold mirror (or gold black) was obtained, although high catalytic activity was achieved, which implied that pentazole gold might be an effective and promising catalyst precursor. As expected, highly active gold nanoparticles were obtained, when this unstable pentazole gold was supported on modified polycyanostyrene microspheres (Scheme 5). Meanwhile, several other gold catalysts were also tested and the experiments disclosed that the modified polycyanostyrene microspheres and pentazole gold were both key impactors for the high activity of this developed catalytic system.
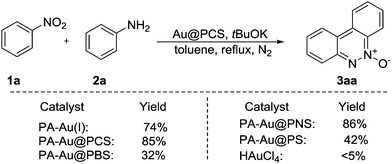 |
| Scheme 5 The experiments for catalyst exploration. | |
Furthermore, we explored the possible reaction pathway to better understand this transformation. We noticed that this reaction might undergo radical pathway and competition experiments for radical progress were carried out. Next, the control reaction of cinnoline synthesis was performed with one equivalent of 2,2,6,6-tetramethyl-1-piperidinyloxy (TEMPO) as a radical scavenger. The experiments showed that the cinnoline product (3aa) couldn’t be obtained, which suggested that this transformation couldn’t rule out the possibility of free-radical intermediates under this catalytic system (Scheme 6).
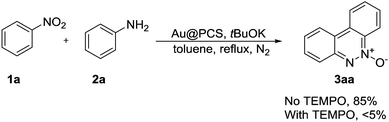 |
| Scheme 6 Control experiments. | |
Reusability of the catalyst
The recycling experiment of a PA-Au–PCS catalyst was next explored. After the reaction of aniline and nitrobenzene was finished, this PA-Au–PCS catalyst was separated easily by centrifugation, followed by washing with EtOH and H2O several times and vacuum freeze-drying for 12 hours. Subsequently, it was found that the reaction of aniline and nitrobenzene could take place in slightly low yields with the recovered PA-Au–PCS polymer as the catalyst even five times (Scheme S1, ESI†). Meanwhile, we also tested the PA-Au–PNS catalyst and it was pleasing that much better recyclability was achieved, when the PA-Au–PNS catalyst was reused. It was observed that almost the same yield was achieved even when the recovered MT–Ir was reused five times (Scheme 7).
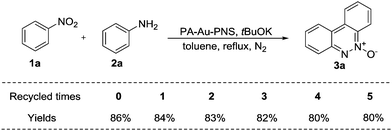 |
| Scheme 7 Recyclability of the PA-Au–PNS catalyst in the reaction of aniline and nitrobenzene. | |
Finally, to better explain this PA-Au–PCS and PA-Au–PNS catalyst, an XRD experiment was performed to examine the recycled catalyst. After five runs, the diffraction peak of gold decreased in PA-Au–PCS, which revealed that there was a loss of gold for PA-Au–PCS in the reaction, which well explained the phenomenon that the reaction rate gradually decreased with the recovered catalyst (Fig. 5). At the same time, XRD experiment of the PA-Au–PNS catalyst revealed that the diffraction peak of gold was not decreased obviously, which implied that high activity with better recyclability for the PA-Au–PNS catalyst was achieved (Fig. 6). In addition, the amount of gold leaching into solution for the reaction of nitrobenzene with aniline was tested through ICP measurements. The amount of gold leaching after five times was determined by ICP assessment and found to be only 2.13% (%, w/w) for PA-Au–PNS, while 18.94% (%, w/w) was found for PA-Au–PCS. Therefore, the ICP analysis well explained the reason for the moderate recyclability of PA-Au–PCS (poor stability) and revealed that PA-Au–PNS had good stability.
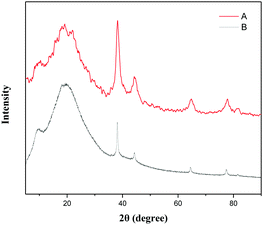 |
| Fig. 5 XRD pattern of (A) fresh PA-Au–PCS catalyst; and (B) PA-Au–PCS catalyst after five runs. | |
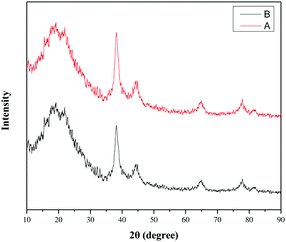 |
| Fig. 6 XRD pattern of (A) fresh PA-Au–PNS catalyst; and (B) PA-Au–PNS catalyst after five runs. | |
Conclusions
In conclusion, gold nanoparticles supported by modified polycyanostyrene and polynitrostyrene microspheres were designed and synthesized, and were characterized using scanning electron microscopy, transmission electron microscopy, energy dispersive X-ray, X-ray power diffraction and X-ray photoelectron spectrometry. This catalytic system successfully achieved applications in the synthesis of cinnoline derivatives and hydration reactions. Further investigation on the range of substrates and other reaction systems is in progress.
Conflicts of interest
There are no conflicts to declare.
Acknowledgements
We gratefully acknowledge financial support of this work by the National Natural Science Foundation of China (21776111 and 21861039), the Fundamental Research Funds for the Central Universities (JUSRP 51627B) and MOE & SAFEA for the 111 Project (B13025).
Notes and references
-
(a) K. Klucinska, E. Jaworska, P. Gryczan, K. Maksymiuk and A. Michalska, Chem. Commun., 2015, 51, 12645 RSC;
(b) B. Li, Y. Xie, M. Jing, G. Rong, Y. Tang and G. Zhang, Langmuir, 2006, 22, 9380 CrossRef CAS;
(c) C. Kahlfuss, S. Denis-Quanquin, N. Calin, E. Dumont, M. Garavelli, G. Royal, S. Cobo, E. Saint-Aman and C. Bucher, J. Am. Chem. Soc., 2016, 138, 15234 CrossRef CAS;
(d) Z. Li and K. S. Suslick, ACS Appl. Mater. Interfaces, 2018, 10, 15820 CrossRef CAS;
(e) M. P. Kim, D. J. Kang, D.-W. Jung, A. G. Kannan, K.-H. Kim, K. H. Ku, S. G. Jang, W.-S. Chae, G.-R. Yi and B. J. Kim, ACS Nano, 2012, 6, 2750 CrossRef CAS PubMed;
(f) J. Xu, G. Chen, R. Yan, D. Wang, M. Zhang, W. Zhang and P. Sun, Macromolecules, 2011, 44, 3730 CrossRef CAS;
(g) Y. Lan, L. Yang, M. Zhang, W. Zhang and S. Wang, ACS Appl. Mater. Interfaces, 2010, 2, 127 CrossRef CAS PubMed;
(h) Z. Deng, H. Zhu, B. Peng, H. Chen, Y. Sun, X. Gang, P. Jin and J. Wang, ACS Appl. Mater. Interfaces, 2012, 4, 5625 CrossRef CAS PubMed;
(i) E. Woznica, K. Maksymiuk and A. Michalska, Anal. Chem., 2014, 86, 411 CrossRef CAS PubMed;
(j) X. Zhou, X. Li, H. Sun, P. Sun, X. Liang, F. Liu, X. Hu and G. Lu, ACS Appl. Mater. Interfaces, 2015, 7, 15414 CrossRef CAS PubMed;
(k) X. Wang, G. Li, G. Hong, Q. Guo and X. Zhang, ACS Appl. Mater. Interfaces, 2017, 9, 41323 CrossRef CAS.
-
(a) T. Wang, Y. Zheng, X. Wang, D. E. Austin and Z. Zhang, Anal. Chem., 2017, 89, 7988 CrossRef CAS;
(b) Q. Zhao, J. Piao, W. Peng, Y. Wang, B. Zhang, X. Gong and J. Chang, ACS Appl. Mater. Interfaces, 2018, 10, 3324 CrossRef CAS;
(c) W. Han, Y. Bai, S. Liu, C. Ge, L. Wang, Z. Ma, Y. Yang and X. Zhang, Composites, Part A, 2017, 102, 218 CrossRef CAS;
(d) F. Faraguna, P. Poetschke and J. Pionteck, Polymer, 2017, 132, 325 CrossRef CAS;
(e) D. Hirayama and C. Saron, Polymer, 2018, 135, 271 CrossRef CAS;
(f) V. Hirschberg, L. Schwab, M. Cziep, M. Wilhelm and D. Rodrigue, Polymer, 2018, 138, 1 CrossRef CAS;
(g) J. Cheng, Z. Chen, J. Zhou, Z. Cao, D. Wu, C. Liu and H. Pu, Appl. Surf. Sci., 2018, 440, 946 CrossRef CAS;
(h) Y.-C. Lo, Y.-J. Chiu, H.-F. Tseng and J.-T. Chen, Langmuir, 2017, 33, 12300 CrossRef CAS PubMed.
-
(a) X. Song, Y. Zhao, H. Wang and Q. Do, Langmuir, 2009, 25, 4443 CrossRef CAS PubMed;
(b) S. Pargen, C. Willems, H. Keul, A. Pich and M. Moeller, Macromolecules, 2012, 45, 1230 CrossRef CAS;
(c) S. T. Ha, O. O. Park and S. H. Im, Macromol. Res., 2010, 18, 935 CrossRef CAS;
(d) Y. Li, Z. Wu and S. Ye, New J. Chem., 2015, 39, 8108 RSC.
-
(a) S. Wang, M. Zhang and W. Zhang, ACS Catal., 2011, 1, 207 CrossRef CAS;
(b) W. Du, J. Guo, H. Li and Y. Gao, ACS Sustainable Chem. Eng., 2017, 5, 7514 CrossRef CAS;
(c) A. Bousquet, R. Perrier-Cornet, E. Ibarboure, E. Papon, C. Labrugere, V. Heroguez and J. Rodriguez-Hernandez, Biomacromolecules, 2008, 9, 1811 CrossRef CAS PubMed;
(d) C. Liu, J. Liu, D. Gao, M. Ding and J.-M. Lin, Anal. Chem., 2010, 82, 9418 CrossRef CAS PubMed;
(e) W. Li, T. Qiu, L. Wang, S. Ren, J. Zhang, L. He and X. Li, ACS Appl. Mater. Interfaces, 2013, 5, 883 CrossRef CAS PubMed;
(f) L.-S. Wan, J. Lv, B.-B. Ke and Z.-K. Xu, ACS Appl. Mater. Interfaces, 2010, 2, 3759 CrossRef CAS PubMed;
(g) J.-B. Qu, Y. Liu, J.-Y. Liu, G.-S. Huan, S.-N. Wei, S.-H. Li and J.-G. Liu, Macromolecules, 2018, 51, 4085 CrossRef CAS;
(h) H. Weng, X. Huang, M. Wang, X. Ji and X. Ge, Langmuir, 2013, 29, 15367 CrossRef CAS PubMed;
(i) A. K. Mishra, C. Choi, S. Maiti, Y. Seo, K. S. Lee, E. Kim and J. K. Kim, Polymer, 2018, 139, 68 CrossRef CAS;
(j) R. Barbey and S. Perrier, Macromolecules, 2014, 47, 6697 CrossRef CAS;
(k) C.-L. Yu, X. Wang, C. Chen and F. Zhang, Ind. Eng. Chem. Res., 2014, 53, 2244 CrossRef CAS.
- For selected recent reviews on Au catalysis, see:
(a) A. Corma, A. Leyva-Pérez and M. Sabater, Chem. Rev., 2011, 111, 1657 CrossRef CAS PubMed;
(b) M. Rudolph and A. S. K. Hashmi, Chem. Soc. Rev., 2012, 41, 2448 RSC;
(c) L.-P. Liu and G. B. Hammond, Chem. Soc. Rev., 2012, 41, 3129 RSC;
(d) D. Garayalde and C. Nevado, ACS Catal., 2012, 2, 1462 CrossRef CAS;
(e) C. Obradors and A. M. Echavarren, Chem. Commun., 2014, 50, 16 RSC;
(f) E. Soriano and I. Fernández, Chem. Soc. Rev., 2014, 43, 3041 RSC;
(g) Y. Zhu, L. Sun, P. Lu and Y. Wang, ACS Catal., 2014, 4, 1911 CrossRef CAS;
(h) A. C. Jones, J. A. May, R. Sarpong and B. M. Stoltz, Angew. Chem., Int. Ed., 2014, 53, 2556 CrossRef CAS PubMed;
(i) R. Dorel and A. M. Echavarren, Chem. Rev., 2015, 115, 9028 CrossRef CAS PubMed;
(j) E. Levin, E. Ivry, C. E. Diesendruck and N. G. Lemcoff, Chem. Rev., 2015, 115, 4607 CrossRef CAS PubMed;
(k) D. Pflästerer and A. S. K. Hashmi, Chem. Soc. Rev., 2016, 45, 1331 RSC;
(l) Z. Zheng, Z. Wang, Y. Wang and L. Zhang, Chem. Soc. Rev., 2016, 45, 4448 RSC;
(m) A. S. K. Hashmi and G. J. Hutchings, Angew. Chem., Int. Ed., 2006, 45, 7896 CrossRef PubMed;
(n) A. M. Asiria, A. Stephen and K. Hashmi, Chem. Soc. Rev., 2016, 45, 4471 RSC.
-
(a) L. Zhang, Acc. Chem. Res., 2014, 47, 877 CrossRef CAS PubMed;
(b) C. Obradors and A. M. Echavarren, Acc. Chem. Res., 2014, 47, 902 CrossRef CAS PubMed;
(c) B. Alcaide and A. P. Almendros, Acc. Chem. Res., 2014, 47, 939 CrossRef CAS PubMed;
(d) D.-H. Zhang, X.-Y. Tang and M. Shi, Acc. Chem. Res., 2014, 47, 913 CrossRef CAS PubMed;
(e) R. K. Shiroodi, M. Soltani and V. Gevorgyan, J. Am. Chem. Soc., 2014, 136, 9882 CrossRef PubMed;
(f) X. Hu, D. Martin, M. Melaimi and G. Bertrand, J. Am. Chem. Soc., 2014, 136, 13594 CrossRef CAS PubMed;
(g) X.-Z. Shu, M. Zhang, Y. He, H. Frei and F. D. Toste, J. Am. Chem. Soc., 2014, 136, 5844 CrossRef CAS PubMed;
(h) S. N. Karad and R.-S. Liu, Angew. Chem., Int. Ed., 2014, 53, 9072 CrossRef CAS PubMed;
(i) Y. Ma, S. Zhang, S. Yang, F. Song and J. You, Angew. Chem., Int. Ed., 2014, 53, 7870 CrossRef CAS PubMed;
(j) L. Zhou, M. Zhang, W. Li and J. Zhang, Angew. Chem., Int. Ed., 2014, 53, 6542 CrossRef CAS PubMed;
(k) J. M. D’Oyley, A. E. Aliev and T. D. Sheppard, Angew. Chem., Int. Ed., 2014, 53, 10747 CrossRef PubMed;
(l) J. Guenther, S. Mallet-Ladeira, L. Estevez, K. Miqueu, A. Amgoune and D. Bourissou, J. Am. Chem. Soc., 2014, 136, 1778 CrossRef CAS PubMed.
-
(a) W. Rao, D. Susanti, B. J. Ayers and P. W. H. Chan, J. Am. Chem. Soc., 2015, 137, 6350 CrossRef CAS PubMed;
(b) C. Shu, Y.-H. Wang, B. Zhou, X.-L. Li, Y.-F. Ping, X. Lu and L.-W. Ye, J. Am. Chem. Soc., 2015, 137, 9567 CrossRef CAS PubMed;
(c) W. Xu, W. Wang and X. Wang, Angew. Chem., Int. Ed., 2015, 54, 9546 CrossRef CAS PubMed;
(d) Y. Tokimizu, S. Oishi, N. Fujii and H. Ohno, Angew. Chem., Int. Ed., 2015, 54, 7862 CrossRef CAS PubMed;
(e) J. C. Timmerman, B. D. Robertson and R. A. Widenhoefer, Angew. Chem., Int. Ed., 2015, 54, 2251 CrossRef CAS PubMed;
(f) M. Chen, Y. Chen, N. Sun, J. Zhao, Y. Liu and Y. Li, Angew. Chem., Int. Ed., 2015, 54, 1200 CrossRef CAS PubMed;
(g) F. Inagaki, C. Matsumoto, Y. Okada, N. Maruyama and C. Mukai, Angew. Chem., Int. Ed., 2015, 54, 818 CrossRef CAS PubMed;
(h) J. Xie, S. Shi, T. Zhang, N. Mehrkens, M. Rudolph and A. S. K. Hashmi, Angew. Chem., Int. Ed., 2015, 54, 6046 CrossRef CAS PubMed;
(i) W. Zi and F. D. Toste, Angew. Chem., Int. Ed., 2015, 54, 14447 CrossRef CAS PubMed;
(j) W. Zi, H. Wu, L. Hongjian and F. D. Toste, Angew. Chem., Int. Ed., 2015, 54, 8529 CrossRef PubMed;
(k) H. Kawai, W. J. Wolf, A. G. DiPasquale, M. S. Winston and F. D. Toste, J. Am. Chem. Soc., 2016, 138, 587 CrossRef CAS PubMed;
(l) Y. Wang, Z. Zheng and L. Zhang, J. Am. Chem. Soc., 2015, 137, 5316 CrossRef CAS PubMed;
(m) Y. Wang, M. Zarca, L.-Z. Gong and L. Zhang, J. Am. Chem. Soc., 2016, 138, 7516 CrossRef CAS PubMed;
(n) Z. Xu, H. Chen, Z. Wang, Z. Ying and L. Zhang, J. Am. Chem. Soc., 2016, 138, 5515 CrossRef CAS PubMed.
-
(a) D.-A. Roşca, D. A. Smith, D. L. Hughes and M. Bochmann, Angew. Chem., Int. Ed., 2012, 51, 10643 CrossRef PubMed;
(b) D.-A. Roşca, J. A. Wright, D. L. Hughes and M. Bochmann, Nat. Commun., 2013, 4, 2167 CrossRef PubMed;
(c) E. Langseth, M. L. Scheuermann, D. Balcells, W. Kaminsky, K. I. Goldberg, O. Eisenstein, R. H. Heyn and M. Tilset, Angew. Chem., Int. Ed., 2013, 52, 1660 CrossRef CAS PubMed;
(d) E. Langseth, A. Nova, E. A. Tråseth, F. Rise, S. Øien, R. H. Heyn and M. Tilset, J. Am. Chem. Soc., 2014, 136, 10104 CrossRef CAS PubMed;
(e) J. Guenther, S. Mallet-Ladeira, L. Estevez, K. Miqueu, A. Amgoune and D. Bourissou, J. Am. Chem. Soc., 2014, 136, 1778 CrossRef CAS PubMed;
(f) M. Joost, A. Amgoune and D. Bourissou, Angew. Chem., Int. Ed., 2015, 54, 15022 CrossRef CAS PubMed;
(g) F. Rekhroukh, R. Brousses, A. Amgoune and D. Bourissou, Angew. Chem., Int. Ed., 2015, 54, 1266 CrossRef CAS PubMed.
-
(a) D. Wang, R. Cai, S. Sharma, J. Jirak, S. K. Thummanapelli, N. G. Akhmedov, H. Zhang, X. Liu, J. Petersen and X. Shi, J. Am. Chem. Soc., 2012, 134, 9012 CrossRef CAS PubMed;
(b) H. Peng, Y. Xi, N. Ronaghi, B. Dong, N. G. Akhmedov and X. Shi, J. Am. Chem. Soc., 2014, 136, 13174 CrossRef CAS PubMed;
(c) Y. Xi, Y. Su, Z. Yu, B. Dong, E. J. McClain, Y. Lan and X. Shi, Angew. Chem., Int. Ed., 2014, 53, 9817 CrossRef CAS PubMed;
(d) Q. Wang, S. E. Motika, N. G. Akhmedov, J. L. Petersen and X. Shi, Angew. Chem., Int. Ed., 2014, 53, 5418 CrossRef CAS PubMed;
(e) H. Peng, N. G. Akhmedov, Y. Liang, N. Jiao and X. Shi, J. Am. Chem. Soc., 2015, 137, 8912 CrossRef CAS PubMed;
(f) R. Cai, M. Lu, E. Y. Aguilera, Y. Xi, N. G. Akhmedov, J. L. Petersen, H. Chen and X. Shi, Angew. Chem., Int. Ed., 2015, 54, 8772 CrossRef CAS PubMed;
(g) C. Qin, Y. Su, T. Shen, X. Shi and N. Jiao, Angew. Chem., Int. Ed., 2016, 55, 350 CrossRef CAS PubMed;
(h) S. E. Motika, Q. Wang, N. G. Akmendov, L. Wojtas and X. Shi, Angew. Chem., Int. Ed., 2016, 55, 11582 CrossRef CAS PubMed;
(i) S. Hosseyni, L. Wojtas, M. Li and X. Shi, J. Am. Chem. Soc., 2016, 138, 3994 CrossRef CAS PubMed.
-
(a) A. S. K. Hashmi and C. Lothschütz, ChemCatChem, 2010, 2, 133 CrossRef CAS;
(b) M. C. B. Jaimes, C. R. N. Bçhling, J. M. Serrano-Becerra and A. S. K. Hashmi, Angew. Chem., Int. Ed., 2013, 52, 7963 CrossRef PubMed;
(c) M. C. B. Jaimes, F. Rominger, M. M. Pereira, R. M. B. Carrilho, S. A. C. Carabineiroc and A. S. K. Hashmi, Chem. Commun., 2014, 50, 4937 RSC;
(d) J. Schießl, J. Schulmeister, A. Doppiu, E. Wörner, M. Rudolph, R. Karch and A. S. K. Hashmi, Adv. Synth. Catal., 2018, 360, 2493 CrossRef.
-
(a) Y. Yang, A. Qin, K. Zhao, D. Wang and X. Shi, Adv. Synth. Catal., 2016, 358, 1433 CrossRef CAS;
(b) Y. Yang, W. Hu, X. Ye, D. Wang and X. Shi, Adv. Synth. Catal., 2016, 358, 2583 CrossRef CAS;
(c) Z. Xu, D.-S. Wang, X. Yu, Y. Yang and D. Wang, Adv. Synth. Catal., 2017, 359, 3332 CrossRef CAS;
(d) R. Huang, Y. Yang, D.-S. Wang, L. Zhang and D. Wang, Org. Chem. Front., 2018, 5, 203 RSC.
-
(a) D. Wang, K. Zhao, C. Xu, H. Miao and Y. Ding, ACS Catal., 2014, 4, 3910 CrossRef CAS;
(b) D. Wang, X. Yu, X. Xu, B. Ge, X. Wang and Y. Zhang, Chem. – Eur. J., 2016, 22, 8663 CrossRef CAS PubMed;
(c) X. Yu, D.-S. Wang, Z. Xu, B. Yang and D. Wang, Org. Chem. Front., 2017, 4, 1011 RSC;
(d) X. Hu, H. Zhu, X. Sang and D. Wang, Adv. Synth. Catal., 2018, 360, 4293 CrossRef CAS;
(e) Q. Wu, L. Pan, G. Du, C. Zhang and D. Wang, Org. Chem. Front., 2018, 5, 2668 RSC;
(f) C. Ge, X. Sang, W. Yao, L. Zhang and D. Wang, Green Chem., 2018, 20, 1805 RSC;
(g) Z. Xu, X. Yu, X. Sang and D. Wang, Green Chem., 2018, 20, 2571 RSC.
-
(a) Y. Yu, S. K. Singh, A. Liu, T.-K. Li, L. F. Liub and E. J. LaVoie, Bioorg. Med. Chem., 2003, 11, 1475 CrossRef CAS PubMed;
(b) V. Benin and P. Kaszynski, J. Org. Chem., 2000, 65, 6388 CrossRef CAS PubMed;
(c) H.-R. Bjørsvik, R. R. González and L. Liguori, J. Org. Chem., 2004, 69, 7720 CrossRef PubMed;
(d) J. R. Hwu, A. R. Das, C. W. Yang, J.-J. Huang and M.-H. Hsu, Org. Lett., 2005, 7, 3211 CrossRef CAS PubMed;
(e) A. Slevin, T. Koolmeister and M. Scobie, Chem. Commun., 2007, 2506 RSC;
(f) M. J. Haddadin, R. M. B. Zerdan, M. J. Kurth and J. C. Fettinger, Org. Lett., 2010, 12, 5502 CrossRef CAS PubMed;
(g) D. Wang, X. Yu, W. Yao, W. Hu, C. Ge and X. Shi, Chem. – Eur. J., 2016, 22, 5543 CrossRef CAS PubMed;
(h) S. K. Sythana, S. Unni, Y. M. Kshirsagar and P. R. Bhagat, Eur. J. Org. Chem., 2014, 311 CrossRef CAS.
Footnote |
† Electronic supplementary information (ESI) available. See DOI: 10.1039/c8qm00510a |
|
This journal is © the Partner Organisations 2019 |
Click here to see how this site uses Cookies. View our privacy policy here.