DOI:
10.1039/C8RA08199A
(Paper)
RSC Adv., 2019,
9, 2152-2155
Polyphenol oxidase/gold nanoparticles/mesoporous carbon-modified electrode as an electrochemical sensing platform for rutin in dark teas†
Received
9th October 2018
, Accepted 6th January 2019
First published on 15th January 2019
Abstract
In the communication, by virtue of the excellent conductivity and great surface area of mesoporous carbon (FDU-15), the enhanced conductivity of Au NPs, and the good electrochemical response of polyphenol oxidase (PPO) to rutin, a PPO/AuNPs/FDU-15-modified electrode was used as a candidate for the determination of rutin in dark teas with satisfactory results.
1. Introduction
Rutin, also known as vitamin P, exists in many plants and multi-vitamin preparations.1–4 As a flavonoid compound, rutin exhibits a broad range of physiological activities, such as antibacterial, anti-aging, antioxidant, and anti-inflammatory, and has been widely used for the treatment of hypertension and vascular fragility.5,6 To date, different methods have been developed for rutin detection, such as capillary electrophoresis,7 performance liquid chromatography (HPLC),8 spectrophotometry,9 and chemiluminescene.10 However, most of them suffered some drawbacks including time-consuming, high-cost, and complicated construction, limiting their practical applications. Therefore, it is necessary to develop a simple, fast, economic, and precise method for the detection of rutin.
Currently, the electroanalytical method has attracted much attention owing to its simple implementation, good reproducibility, and high sensitivity.11 Given that the electrochemical active group of four hydroxyl groups in rutin, there have been many electroanalytical methods for the determination of rutin over the past decades.12–15 To the best of our knowledge, mesoporous carbon materials (FDU-15) with the features of tunable nanopores, fast electron transfer, and excellent electrocatalytic activity, have made it a good candidate for adsorbent, sensor and supercapacitor.16–21 For example, FDU-15 immobilized with GOD could efficiently enhance the electron transfer rate,22 but it lacked good thermostability. And glassy carbon electrodes modified with ordered FDU-15 have been widely used for the detection of morphine via electrochemical oxidation reaction,23 while the synthesis of them is complex. So, it is of significance to develop a FDU-15-based probe with simple synthesis, good stability, and enhanced transfer rate for the sensitive determination of rutin.
Recognizing these flaws, a simple method of evaporation induced self-assembly (EISA)24 was utilized to prepare phenolic resin as substrate of FDU-15. In this paper, FDU-15 was mobilized on the surface of bare glassy carbon electrode (GCE) to adsorb rutin owing to its excellent conductivity and great surface area (FDU-15/GCE), further functionalized with gold nanoparticles (AuNPs) to enhance the conductivity (AuNPs/FDU-15/GCE), then polyphenol oxidase (PPO) was tethered on the gaps of FDU-15 (PPO/AuNPs/FDU-15/GCE) owing to its excellent electrochemical response to rutin. Initially, the electrode exhibited quenched electrochemical signal, while an obvious catalytic oxidation reaction occurred on the surface of the electrode, inducing a significant increase in electrochemical signal, as depicted in Scheme 1. This developed electrochemical sensing platform was simple and low-cost, as well as highly selective and reliable, which would be a promising candidate for the detection of rutin levels in dark teas.
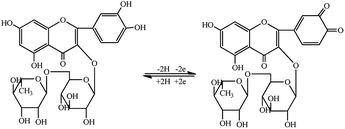 |
| Scheme 1 Schematic representation of electrochemical mechanism to rutin. | |
2. Results and discussion
2.1 Characterization of FDU-15
FDU-15 was prepared by EISA according to the reporting method,24 using surfactant of Pluronic F127 to obtain phenolic resin prepolymer, following by copolycondensation at room temperature. The obtained FDU-15 was characterized by field emission scanning electron microscopy (FESEM), transmission electron microscopy (TEM), and small-angle powder X-ray diffraction patterns (XRD), respectively. FDU-15 displayed the layered structure (Fig. 1A), and good mesoporous by the clear and orderly channel (Fig. 1B). Fig. 1C depicts a small-angle diffraction peak at about 0.8°, indicating the phenolic resin (100) plane of FDU-15 structure, with average pore size of about 4 nm. The results proved the mesoporous structure of FDU-15 was two-dimensional six-square channel.
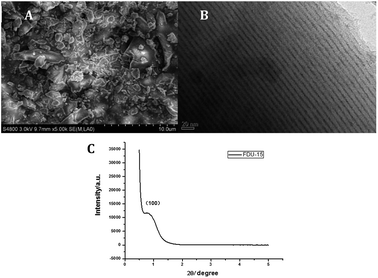 |
| Fig. 1 Characterization of FDU-15. FESEM (A), TEM (B), and XRD (C) images of FDU-15. | |
2.2 Electrochemical behavior of PPO/AuNPs/FDU-15/GCE
Our electrode was prepared following the process via the layer-by-layer assembly, of which bare GCE was functionalized successively to obtain FDU-15/GCE, AuNPs/FDU-15/GCE and PPO/AuNPs/FDU-15/GCE respectively. And their impedance measurements were performed by electrochemical impedance spectroscopy (ESI) in 1.0 mM of [Fe(CN)6]4−/3− containing 0.1 M of KCl solution. The electron transfer resistance of Ret can be seen from the semicircle domains of impedance spectra, and the smaller Ret, the better conductivity of the electrode. As shown in Fig. 2A, the GCE is naked with small Ret (curve a), while FDU-15/GCE exhibits large Ret upon modification of FDU-15 (curve b). After electrochemical deposition of AuNPs, the impedance value of AuNPs/FDU-15/GCE was dramatically reduced, which was similar to that of the GCE (curve c), demonstrating a good conductivity of AuNPs, promoting the electron transfer rate. Due to the poor conductivity, the biological macromolecular protein of PPO induced the charge transfer resistance (curve d). These results confirmed the successful construction of PPO/AuNPs/FDU-15/GCE via the layer-by-layer assembly process.
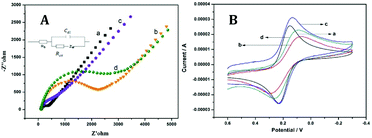 |
| Fig. 2 (A) EIS and (B) CV curves of (a) bare GCE, (b) FDU-15/GCE, (c) AuNPs/FDU-15/GCE and (d) PPO/AuNPs/FDU-15/GCE in PBS buffer solution containing 1.0 mM [Fe(CN)6]4−/3− and 0.1 M of KCl. | |
Furthermore, their cyclic voltammograms (CVs) were performed to prove the successful preparation of our electrode, in which the larger Ret value, the smaller corresponding peak current. As seen in Fig. 2B, the modified FDU-15 on bare GCE causes decreased peak current, and also a strong peak potential shift from 0.1 V to 0.05 V. In addition, the further deposited AuNPs displays increased peak current, suggesting that AuNPs could increase the specific surface area of electrode and promote the electron transfer on the surface of GCE. Especially, after the functionalization with PPO, the electrode exhibited the decreased peak current. These results were in line with that of Fig. 2A, further illustrating the layers-by-layer stacking of PPO/AuNPs/FDU-15/GCE electrode.
To examine the electrochemical effective surface area of the bare GCE and PPO/AuNPs/FDU-15/GCE, chronocoulometry was utilized according to the Anson's equation.25 Fig. S1† depicts the effective surface area of the naked GCE which was calculated to be 0.00105 cm2, while that of PPO/AuNPs/FDU-15/GCE to be 0.00360 cm2. The result indicated that the surface area of GCE was increased significantly after the layers assembly, which would be conducive to the transmission of electrons and the electrochemical response to rutin.
2.3 Determination of rutin in an aqueous solution
Owing to the aforementioned experimental results, we set about to investigate the electrochemical response of the electrode to rutin solution (10.0 μM). As shown in Fig. S2†, after incubation with rutin, the bare GCE and FDU-15/GCE display unobvious redox peak within the range of electric potential from 0 to 1.0 V, suggesting that there was no redox reaction on the surface of the electrodes. Compared to them, however, AuNPs/FDU-15/GCE exhibited enhanced peak current, even at about 0.57 V in PBS buffer solution (0.1 M, pH 2.0), indicating a good electrochemical response of AuNPs to rutin. Interestingly, upon modification of the layer of PPO, that is PPO/AuNPs/FDU-15/GCE, there was obviously enhanced redox peak current, in particular at about 0.57 V, further indicating the layer-by-layer assembly could efficiently enhance the electrochemical response to rutin. The results demonstrated that it was feasible to utilize PPO/AuNPs/FDU-15/GCE to detect rutin.
To obtain the optimum detection conditions, different experimental conditions including pH and scan rates were investigated upon incubation with rutin. As seen in Fig. S3†, the anodic peak potential is decreased with the increase of pH value from 2.0 to 8.0, and the slop is −62.6 mV pH−1 with R2 value of 0.9935, in particular close to the theoretical value of −57.6 mV pH−1 in single electron transfer process.26 Thus, the pH value of 2.0 was utilized in the following experiments. Furthermore, when the scan rate increased, the peak current gradually increased within the range of scan rate from 200 to 900 mV s−1, indicating that the electrochemical reaction of rutin on the electrode is a surface adsorption-controlled process, as shown in Fig. S4†. In particular, the increased peak potential shifted to positive direction, proving the reaction is irreversible.
To validate the sensitivity of our assay, the chronoamperometric curve of PPO/AuNPs/FDU-15/GCE were depicted in Fig. 3A at the working potential of 0.57 V. The results revealed that the oxidation current gradually increased with an increase in the concentration of rutin. Fig. 3B describes the linear relationship between the current and rutin levels. The current was good linearity with the concentration of rutin within the range of 1.5 × 10−6 to 2.8 × 10−5 M with R2 value of 0.998. The limit of detection (LOD) at signal-to-noise ratio of 3 for rutin was 5.1 × 10−7 M, demonstrating a relative sensitive assay method compared with previous reports.6 When the electrode was stored in the vacuum oven even for 24 h at room temperature, the corresponding standard deviation was less than 4.6% (data not shown), suggesting a good stability of the our constructed electrode. Compare to reported methods,12–15 our strategy exhibited excellent application prospect (Table S1†). These results indicated that our electrode can be employed as a potential candidate for the determination of rutin.
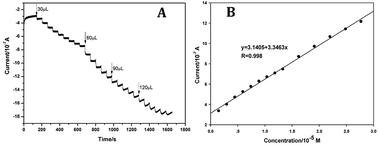 |
| Fig. 3 (A) Amperometric response of PPO/AuNPs/FDU-15/GCE to rutin at different concentrations in PBS buffer solution (0.1 M, pH 2.0) at working potential of 0.57 V. (B) Relationship between the current and rutin levels. | |
2.4 Determination of rutin in dark teas samples
To employ the modified electrode for the determination of rutin in real samples, the effect of common interfering species including inorganic ions of K+, Zn2+, Na+, NO3−, Cl− and PO43− (date not shown) and micromolecules were investigated, as seen in Fig. S5†. It was found that some common substances including glucose, p-aminophenol (PAP) and ascorbic acid (AA), even at 100 fold concentration, exhibited negligible change in the response current when they were coexisted in rutin. The good selectivity of PPO/Au/FDU-15/GCE demonstrated the feasibility for discriminating rutin from others in real samples.
Finally, the proposed electrode was performed for the determination of rutin in dark teas samples. The known amounts of rutin were added to the aqueous extract of dark teas, and the results were obtained by the standard addition method. As seen in Table 1, the average recoveries of rutin were in the range from 98.5% to 105.5% with the standard deviation of less than 2.2%. The obtained satisfactory results demonstrated the applicability for the determination of rutin in dark teas by the prepared PPO/Au/FDU-15/GCE via layer-by-layer assembly.
Table 1 Determination of rutin in dark teas samples
Samples |
Original found by this method |
Added (μM) |
Found (μM) |
Recovery (%) |
RSD (n = 3, %) |
ND: not detectable. |
1# |
NDa |
5.0 |
5.04 |
100.8 |
1.6 |
2# |
NDa |
10.0 |
9.85 |
98.5 |
2.3 |
3# |
NDa |
15.0 |
15.5 |
103.3 |
1.7 |
4# |
NDa |
20.0 |
20.2 |
101.0 |
2.0 |
3. Conclusions
In summary, we have developed a modified electrode PPO/AuNPs/FDU-15/GCE for the electrochemical detection of rutin. After successive modification of FDu-15, AuNPs and PPO on GCE, the constructed PPO/AuNPs/FDU-15/GCE was applied to discriminate rutin from ions and other common micromolecules, which exhibited a good specificity and stability. The electrochemical method for the determination of rutin had the linear range from 1.5 × 10−6 to 2.8 × 10−5 M with LOD down to 5.1 × 10−7 M. In particularly, PPO/AuNPs/FDU-15/GCE was successfully employed for rutin assay in dark teas with satisfactory results.
Conflicts of interest
There are no conflicts to declare.
Acknowledgements
The work was for the financial support from National Natural Science Foundation of China (No. 21275100, 21675042).
References
- W. Sun, D. Wang, Y.-Y. Zhang, X.-M. Ju, H.-X. Yang, Y.-X. Chen and Z.-F. Sun, Chinese J. Anal. Chem., 2013, 41(5), 709–713 CrossRef CAS.
- W. Sun, X. Wang, H. Zhu, X. Sun, F. Shi, G. Li and Z. Sun, Sens. Actuators, B, 2013, 178, 443–449 CrossRef CAS.
- W. Sun, Y. Wang, S. Gong, Y. Cheng, F. Shi and Z. Sun, Electrochim. Acta, 2013, 109, 298–304 CrossRef CAS.
- A. Y. Tesio, S. N. Robledo, A. M. Granero, H. Fernández and M. A. Zon, Sens. Actuators, B, 2014, 203, 655–662 CrossRef CAS.
- J. An, Y.-Y. Bi, C.-X. Yang, F.-D. Hu and C.-M. Wang, J. Pharm. Anal., 2013, 3(2), 102–108 CrossRef CAS PubMed.
- C. Cheng, Y. Huang, J. Wang, B. Zheng, H. Yuan and D. Xiao, Anal. Chem., 2013, 85(5), 2601–2605 CrossRef CAS PubMed.
- H. Cheng, J. Liang, Q. Zhang and Y. Tu, J. Electroanal. Chem., 2012, 674, 7–11 CrossRef CAS.
- K. Liu, J. Wei and C. Wang, Electrochim. Acta, 2011, 56(14), 5189–5194 CrossRef CAS.
- J.-L. He, Y. Yang, X. Yang, Y.-L. Liu, Z.-H. Liu, G.-L. Shen and R.-Q. Yu, Sens. Actuators, B, 2006, 114(1), 94–100 CrossRef CAS.
- Y. Huang, H. Yan and Y. Tong, J. Electroanal. Chem., 2015, 743, 25–30 CrossRef CAS.
- Y. Liu and J. Zhang, Microporous Mesoporous Mater., 2015, 206, 81–85 CrossRef CAS.
- D. Miao, J. Li, R. Yang, J. Qu, L. Qu and P. d. B. Harrington, J. Electroanal. Chem., 2014, 732, 17–24 CrossRef CAS.
- Y. Miao, Z. Zhang, Y. Gong, Q. Zhang and G. Yan, Biosens. Bioelectron., 2014, 52, 271–276 CrossRef CAS PubMed.
- W. Sun, L. Dong, Y. Lu, Y. Deng, J. Yu, X. Sun and Q. Zhu, Sens. Actuators, B, 2014, 199, 36–41 CrossRef CAS.
- W. Sun, Z. Sun, L. Zhang, X. Qi, G. Li, J. Wu and M. Wang, Colloids Surf., B, 2013, 101, 177–182 CrossRef CAS PubMed.
- Z. Dong, C. Dong, Y. Liu, X. Le, Z. Jin and J. Ma, Chem. Eng. J., 2015, 270, 215–222 CrossRef CAS.
- J. Gao, X. Wang, Q. Zhao, Y. Zhang and J. Liu, Electrochim. Acta, 2015, 163, 223–231 CrossRef CAS.
- Y. Gao, W. Zhu, J. Liu, D. Di, D. Chang, T. Jiang and S. Wang, Int. J. Pharm., 2015, 485(1–2), 25–30 CrossRef CAS PubMed.
- J. Górka, L. Baggetto, J. K. Keum, S. M. Mahurin, R. T. Mayes, S. Dai and G. M. Veith, J. Power Sources, 2015, 284, 1–9 CrossRef.
- Z. Li, D. Li, Z. Liu, B. Li, C. Ge and Y. Fang, Electrochim. Acta, 2015, 158, 237–245 CrossRef CAS.
- D.-H. Lin, Y.-X. Jiang, S.-R. Chen, S.-P. Chen and S.-G. Sun, Electrochim. Acta, 2012, 67, 127–132 CrossRef CAS.
- L. Wang, J. Bai, X. Bo, X. Zhang and L. Guo, Talanta, 2011, 83(5), 1386–1391 CrossRef CAS PubMed.
- N. F. Atta, A. Galal and E. H. El-Ads, Int. J. Electrochem. Sci., 2014, 9, 2113–2131 Search PubMed.
- Y. Meng, D. Gu, F. Zhang, Y. Shi, L. Cheng, D. Feng, Z. Wu, Z. Chen, Y. Wan, A. Stein and D. Zhao, Chem. Mater., 2006, 18(18), 4447–4464 CrossRef CAS.
- M. M. Islam and R. Kant, Electrochim. Acta, 2011, 56(12), 4467–4474 CrossRef CAS.
- Y. Meng, D. Gu, F. Zhang, Y. Shi, L. Cheng, D. Feng, Z. Wu, Z. Chen, Y. Wan, A. Stein and D. Zhao, Chem. Mater., 2006, 18(18), 4447–4464 CrossRef CAS.
Footnote |
† Electronic supplementary information (ESI) available. See DOI: 10.1039/c8ra08199a |
|
This journal is © The Royal Society of Chemistry 2019 |