DOI:
10.1039/C8RA08659A
(Paper)
RSC Adv., 2019,
9, 8104-8112
Polydatin ameliorates pulmonary fibrosis by suppressing inflammation and the epithelial mesenchymal transition via inhibiting the TGF-β/Smad signaling pathway
Received
19th October 2018
, Accepted 22nd January 2019
First published on 12th March 2019
Abstract
Pulmonary fibrosis is a chronic and progressive lung disease which results in a loss of pulmonary function and eventually respiratory failure. Inflammation and epithelial mesenchymal transition (EMT) play important roles in the pathogenesis of pulmonary fibrosis. This study aimed to investigate the therapeutic effect of polydatin (PD) in bleomycin-induced pulmonary fibrosis. A bleomycin-induced pulmonary fibrosis animal model used SD rats. Morphological changes were analyzed by hematoxylin-eosin staining. RT-qPCR and western blot were used for the detection of the expression of TGF-β1, collagen I, collagen III, E-cadherin, fibronectin and the ratios of p-Smad2/Smad2, p-Smad3/Smad3. The concentrations of PICP, PIIINP, TNF-α, IL-1β, IL-6 and IL-17 were measured by enzyme linked immunosorbent assay (Elisa) assay. Results showed that PD attenuated bleomycin-induced pulmonary fibrosis. The beneficial effect of PD was possibly related to the inhibition of inflammation and EMT through suppressing the TGF-β/Smad signaling pathway. Our findings suggested that PD might be a potential therapeutic candidate in the treatment of pulmonary fibrosis.
Introduction
Pulmonary fibrosis is a chronic and progressive lung disease which involves the gradual exchange of normal lung parenchyma with fibrotic tissue. Pulmonary fibrosis is characterized by fibroblast proliferation, excessive collagen accumulation and deposition of extracellular matrix (ECM) proteins which result in a loss of pulmonary function and eventually respiratory failure.1
The etiology of pulmonary fibrosis is various, of which inflammation is reported to play an important role in the pathogenesis of pulmonary fibrosis.2 Transforming growth factor (TGF)-β1 is an important mediator involved in pulmonary inflammation and the progression of fibrotic changes.3,4 TGF-β1 can induce collagen deposition, increase ECM production, and increase α-smooth muscle actin (α-SMA) expression in lung tissue, which contribute to pulmonary fibrosis.5,6 Besides that, fibroblasts and myofibroblasts which are involved in the repair of aberrant tissues and deposition of ECM components also play key roles in pulmonary fibrosis.7 Fibroblasts and myofibroblasts proliferate during the progression of fibrosis, leading to the disorder of epithelial–mesenchymal cells.8 In the patients with pulmonary fibrosis, approximately 80% of alveolar epithelial cells in the fibrotic loci express the mesenchymal marker α-SMA which is the feature of the epithelial-mesenchymal transition (EMT).9 Taken together, these findings suggest that inflammation and EMT may be potential key steps during pulmonary fibrosis, and inhibition of inflammation and EMT may be useful ways to ameliorate pulmonary fibrosis.
Polydatin (3,4′,5-trihydroxystibene-3-β-mono-D-glucoside; PD), is a natural component extracted from perennial herb Polygonum cuspidatum and a traditional Chinese herbal medicine.10 Previous studies indicated that PD exerted multiple biological activities including anti-inflammation and anti-oxidation. Chen reported that PD attenuated kidney inflammation by inhibiting NF-κB/NLRP3 inflammasome activation.11 Moreover, Cao reported that PD alleviated radiation-induced lung injury through activation of Sirt3 and inhibition of EMT.12 These studies revealed that PD had anti-inflammation and anti-EMT effects, which might be an effective agent for the treatment of pulmonary fibrosis. However, investigations on whether PD can ameliorate pulmonary fibrosis are few and more studies are needed.
In our present study, we established pulmonary fibrosis animal model using Sprague-Dawley (SD) rats and explored the effect of PD on the pathogenesis of pulmonary fibrosis. We observed that PD ameliorated pulmonary fibrosis through suppressing inflammation and EMT via inhibiting TGF-β/Smad signaling pathway.
Materials and methods
Reagents
Polydatin was obtained from the National Institutes for Food and Drug Control (purity >98%, HPLC; Beijing, China) (Fig. 1A). Bleomycin was bought from Taihe Co, Tianjin, China. PD was purchased from TW Reagent Co., Ltd, Shanghai, China and dissolved in dimethyl sulfoxide (DMSO) at a stock solution of 10 mM and directly diluted in medium to appropriate concentrations prior to the experiments. PICP, PIIINP, TNF-α, IL-1β, IL-6 and IL-17 Elisa kits were obtained from Wuhan Boster Biological Technology Ltd., Wuhan, P. R. China. TGF-β was obtained from Sigma, St. Louis, MO, USA. Primary antibodies against E-cadherin, fibronectin, p-Smad2, Smad2, p-Smad3, Smad3 and GAPDH were purchased from Cell Signalling Technology, CST, Beverly, Massachusetts.
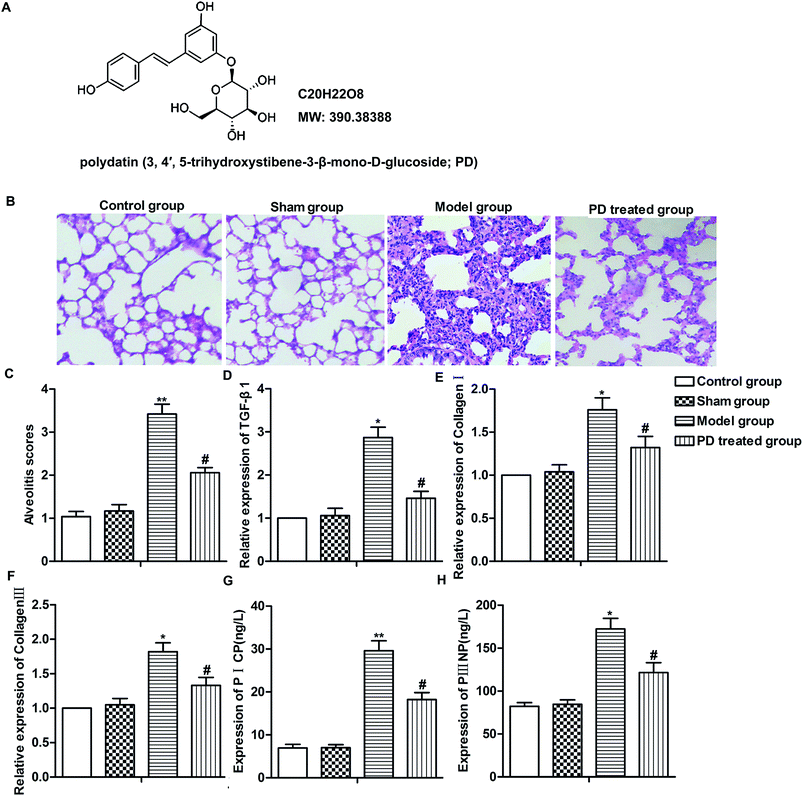 |
| Fig. 1 PD relieves bleomycin-induced pulmonary fibrosis. SD rats were divided into control group, sham group, model group and PD treated group as described in Materials and methods. (A) The chemical structure of PD. (B) Lung tissues in different groups were evaluated by HE staining. (C) Alveolitis scores of each group. (D–F) Relative expression of TGF-β1, collagen I and collagen III was measured by RT-qPCR. (G and H) Expression of PICP and PIIINP was detected by Elisa assay. All data were represented as the mean ± SD from three independent experiments. *P < 0.05, **P < 0.01 compared with sham group, #P < 0.05 compared with model group. | |
Animals and induction of pulmonary fibrosis
Male, 4–6 weeks-old and weighing 180–220 g SD rats were provided by the Experimental Animal Center of The Third Affiliated Hospital of Beijing University of Chinese Medicine. Rats were kept in an air-conditioned room with 12 h light cycle at 20–25 °C and 45–55% humidity, and were fed with standard laboratory chow and tap water ad libitum. Animal experiments were performed according to the guidelines of the National Institute of Health (NIH) and were approved by Medical Ethics Committee of The Third Affiliated Hospital of Beijing University of Chinese Medicine.
Pulmonary fibrosis animal model was conducted using bleomycin according to the published method.13 Briefly, rats were anaesthetized and then were cut in the middle of the neck skin. Trachea was exposed after separation of subcutaneous tissue, muscle, and fat, and was punctured with a needle of 1 ml syringe. Bleomycin (4 mg ml−1) in saline solution was delivered into the trachea with a modified syringe needle in a dosage of 5 mg kg−1 body weight. The rats were rotated immediately after receiving bleomycin to ensure an even drug distribution in the lung. Then the neck skin incision was sewn. After recovery from anesthesia, the rats were returned to their cages and allowed free access to food and water as normal. The rats were divided into 4 group with 6 mice in each group: (1) control group: normal and healthy SD rats without treatment; (2) sham group: rats received an operation and intratracheal instillation of the same volume of sterile saline; (3) model group: rats received an operation and a single intratracheal instillation of bleomycin; (4) PD treated group: rats received an operation and a combination with intratracheal instillation of bleomycin and intraperitoneal injection of PD (100 mg per kg per day) 28 days after administration of single-dose bleomycin. The dose of PD was selected according to the literature14 All rats (not model group) were sacrificed after 29 days of the bleomycin injection. The model group was sacrificed on the 14th of bleomycin administration to validate bleomycin-induced lung fibrosis on the 14th of the experiment.
Cell culture and treatment
Human fetal lung fibroblasts (HFL-1) were purchased from American Type Culture Collection (ATCC). Cells were cultured in Ham's F12K medium (Sigma, St Louis, MO) containing 10% FBS and 1% antibiotic-antimycotic solution at 37 °C in a humidified 5% CO2 incubator. HFL-1 cells were divided into 5 groups: (1) control group: HFL-1 cells without treatment; (2) DMSO group: HFL-1 cells were treated DMSO for 48 h; (3) TGF-β group: HFL-1 cells were treated with 10 ng ml−1 TGF-β for 48 h; (4) TGF-β + PD 50 μM group: HFL-1 cells were treated with 10 ng ml−1 TGF-β and 50 μM PD for 48 h; (5) TGF-β + PD 150 μM group: HFL-1 cells were treated with 10 ng ml−1 TGF-β and 150 μM PD for 48 h.
Morphological analysis and histochemical staining
The grades of pulmonary inflammation and fibrosis were analyzed by Hematoxylin–Eosin (HE) staining. Biopsies of lung tissues were fixed with 10% paraformaldehyde in sterile phosphate-buffered saline and em-bedded in paraffin wax. Sections were cut at 5 μm thickness using a microtome and deparaffinized tissue sections were subjected to staining for histological examination. Sections were stained with HE to observe morphology changes as described before.15 The slides were examined by light microscopy and photographed. Lung inflammation and fibrosis were evaluated by Szapiel scoring method, a standard four point scoring scale for intensity, with each slide scored as 1, 2, 3 or 4.
Reverse transcription-quantitative polymerase chain reaction (RT-qPCR)
Total RNAs were extracted from lung tissues using TRIzol (Invitrogen; Thermo Fisher Scientific, Inc.) according to the manufacturer's protocol. PrimeScript RT reagent kit (Takara Bio, Inc., Otsu, Japan) was used for transcription from RNA to cDNA. qPCR was performed in a final volume of 20 μL using a 2× SYBR Green qPCR kit (Takara Bio, Inc., Otsu, Japan). The expression levels of TGF-β1, collagen I and collagen III were calculated using the 2−ΔΔCt method with GAPDH as an internal control for normalization.
Enzyme linked immunosorbent assay (Elisa) assay
Blood samples were collected from rats and the serum was separated. Procollagen type I carboxy-terminal peptide (PICP), procollagen type III aminoterminal peptide (PIIINP), TNF-α, IL-1β, IL-6 and IL-17 in serum were determined by ELISA kits following the manufacturer's instructions.
Cell Counting Kit-8 (CCK-8) assay
CCK-8 assay was used for cell proliferation test. Cells were seeded into a 96-well plate with 2 × 103 cells per well in triplicate. The CCK-8 reagent (Dojindo Molecular Technologies, Inc. Japan) was added in each well (5 mg ml−1) at indicated time points and then incubated in dark at 37 °C for 2 h. Absorbance was determined at a wavelength of 450 nm.
Wound healing assay
HFL-1 cells (5 × 105) were seeded into 6-well plates and incubated for 24 h. After reaching 90–100% confluence, a sterile pipette tip was used to create a straight scratch to form a wound. After washing with PBS twice, the cells were cultured for another 24 h. Mitomycin C (10 μg ml−1) was added to the cell culture medium to inhibit cell replication according to literature.16 Cells which migrated to the wounded area were visualized and images were captured, the percentage of wound closure was determined.
Western blot assay
Proteins were extracted from cells using RIPA lysis buffer (Beyotime Institute of Biotechnology, Shanghai, China). The same amount of proteins were separated by 10% SDS-PAGE gel and then transferred into PVDF membranes (Bio-Rad Laboratories, Inc., Hercules, CA, USA). After blocking with 5% nonfat milk, the membranes were incubated with primary antibodies against E-cadherin (#14472, 1
:
1000, Cell Signalling Technology, CST, Beverly, Massachusetts), fibronectin (ab2413, 1
:
1000, Abcam, Cambridge, UK), p-Smad2 (#18338, 1
:
1000, CST), Smad2 (#8685, 1
:
1000, CST), p-Smad3 (#9520, 1
:
1000, CST), Smad3 (#9523, 1; 1000, CST) and GAPDH (#5174, 1
:
1000, CST) respectively at 4 °C overnight. Then the membranes were incubated with corresponding peroxidase-linked secondary antibodies at room temperature for 1 h. Signal detection was performed using an ECL system (Millipore, Billerica MA, USA).
Statistical analysis
Data was presented as the mean ± standard deviation (SD). Statistical significance was performed using Student's t test or one-way ANOVA test with SPSS 19.0. The difference was considered statistically significant at P < 0.05.
Results
PD relieves bleomycin-induced pulmonary fibrosis
Pulmonary fibrosis was induced by intratracheal administration of bleomycin in our study. As shown in Fig. 1B, in contrast to the normal alveolar morphology in control group and sham group, bleomycin treatment induced obvious alveolar wall thickening and massive infiltration of leukocytes in the interstitium, resulting in higher alveolitis scores compared with control group and sham group. However, PD treatment ameliorated interstitial fibrosis, infiltration of leukocytes and decreased alveolitis scores compared with model group significantly (Fig. 1B and C, **P < 0.01, #P < 0.05). Moreover, the level of TGF-β1, collagen I, collagen III, PICP and PIIINP were fibrosis indicators according to previous reports,17,18 thus, we detected the influence of PD on these biomarkers in bleomycin-induced pulmonary fibrosis model. Results from RT-qPCR and Elisa showed that bleomycin up-regulated the expression of TGF-β1, collagen I, collagen III and induced the production of PICP and PIIINP while PD suppressed these bleomycin-induced increase significantly (Fig. 1D–H, *P < 0.05, **P < 0.01, #P < 0.05). Our data indicated that PD relieved bleomycin-induced pulmonary fibrosis.
PD suppresses inflammation in pulmonary fibrosis
As inflammation plays an important role in the pathogenesis of pulmonary fibrosis. Report suggest that TNF-α, IL-1β, IL-6 and IL-17 were pro-inflammatory cytokines/chemokines that was associated with lung injury.19 Thus, we measured the expression of some inflammation cytokines (TNF-α, IL-1β, IL-6 and IL-17) using Elisa assay. We observed that bleomycin induced the production of TNF-α, IL-1β, IL-6 and IL-17 compared with sham group. However, PD treatment inhibited the production of these inflammation cytokines compared with model group (Fig. 2A–D, *P < 0.05, **P < 0.01, #P < 0.05). These results suggested that PD suppressed inflammation in pulmonary fibrosis.
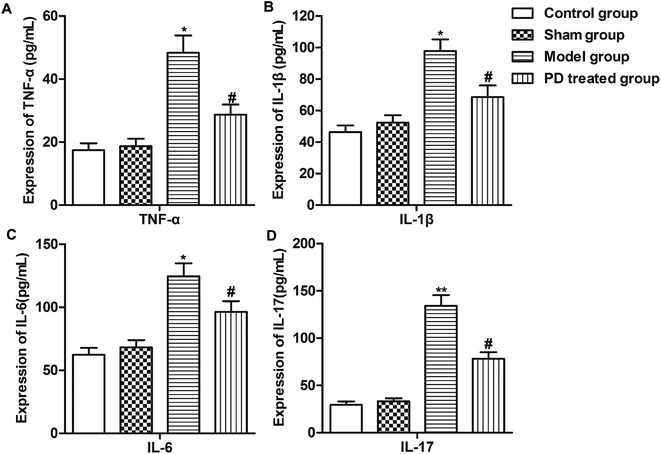 |
| Fig. 2 PD suppresses inflammation in pulmonary fibrosis. SD rats were divided into control group, sham group, model group and PD treated group as described in Materials and methods. (A–D) Expression of TNF-α, IL-1β, IL-6 and IL-17 was detected by Elisa assay. All data were represented as the mean ± SD from three independent experiments. *P < 0.05, **P < 0.01 compared with sham group, #P < 0.05 compared with model group. | |
PD inhibits EMT in pulmonary fibrosis
In vitro experiments were conducted using HFL-1 cells. The toxicological properties of polydatin were detected in HFL-1 cells. The results displayed that the cell viability was strongly reduced under treatment of PD over 200 μM. No significant difference of cell viability was observed in HFL-1 cells treated with PD ≤ 150 μM (Fig. 3A). Thus, we chose low dose (50 μM) and high dose (150 μM) PD for the following experiment. TGF-β plays a crucial role in promoting EMT.20 TGF-β was used to induce fibrosis of HFL-1 cells. The results showed that TGF-β promoted migration of HFL-1 cells compared with control group. PD treatment suppressed the increased migration of HFL-1 cells induced by TGF-β in a dose-dependent manner (Fig. 3B and C, *P < 0.05, #P < 0.05). Besides that, TGF-β decreased the expression of epithelial markers (E-cadherin) and increased the expression of fibronectin, which has been reported serve to localize TGF-β1 signaling to drive EMT.21 While PD treatment had the opposite effects on the expression of E-cadherin and fibronectin compared with TGF-β group dose-dependently (Fig. 3D–F, **P < 0.01, #P < 0.05, ##P < 0.01). Taken together, our results demonstrated that PD inhibited EMT in pulmonary fibrosis.
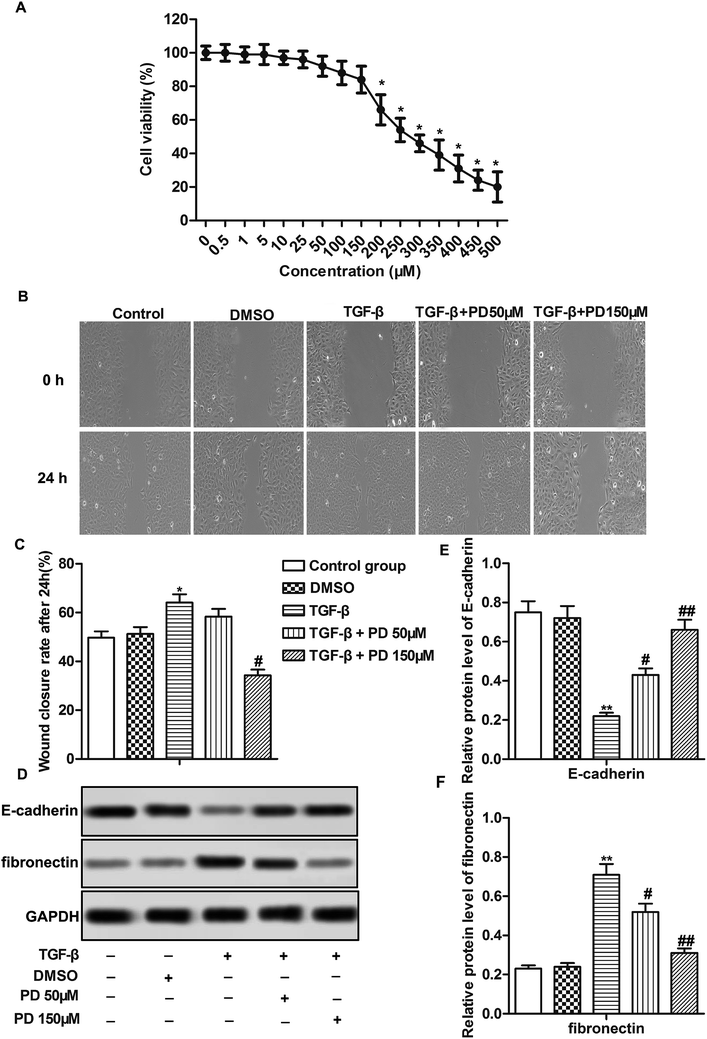 |
| Fig. 3 PD inhibits EMT in pulmonary fibrosis. HLF-1 cells were treated with DMSO, TGF-β, TGF-β + PD 50 μM, TGF-β + PD 150 μM respectively. (A) The toxicological properties of polydatin was detected by CCK-8 assay. (B and C) Cell migration ability was detected by wound healing assay. (D–F) Relative protein level of E-cadherin and fibronectin was detected by western blot. All data were represented as the mean ± SD from three independent experiments. **P < 0.01 compared with control group, #P < 0.05, ##P < 0.01 compared with TGF-β group. | |
PD suppresses TGF-β/Smad signaling pathway in pulmonary fibrosis
TGF-β/Smad signaling pathway is reported to be involved in pulmonary fibrosis.22 Therefore, we investigated relative protein level of Smad2, Smad3 which were key proteins in TGF-β/Smad signaling pathway and their phosphorylation level. The data indicated that TGF-β increased the level of TGF-β and the ratios of p-Smad2/Smad2 and p-Smad3/Smad3, suggesting that TGF-β activated TGF-β/Smad signaling pathway during pulmonary fibrosis. However, PD decreased the level of TGF-β and the ratios of p-Smad2/Smad2 and p-Smad3/Smad3 compared with TGF-β group in a dose-dependent manner (Fig. 4A–C, **P < 0.01, #P < 0.05, ##P < 0.01). These results revealed that PD inhibited TGF-β/Smad signaling pathway in pulmonary fibrosis.
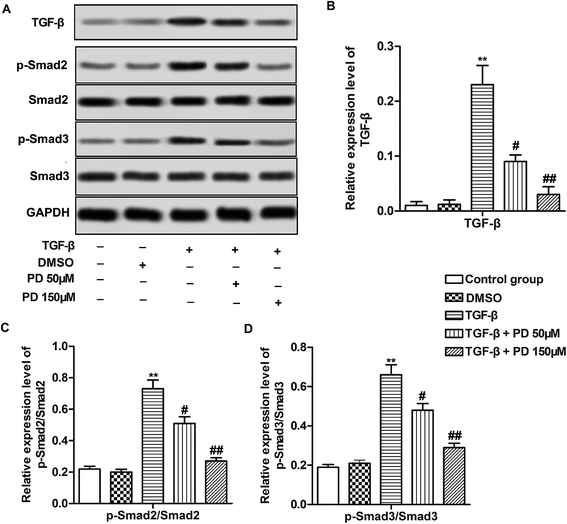 |
| Fig. 4 PD suppresses TGF-β/Smad signaling pathway in pulmonary fibrosis. (A) Relative protein level of TGF-β, p-Smad2, Smad2, p-Smad3, Smad3 was detected by Western blot. (B–D) The ratios of TGF-β, p-Smad2/Smad2 and p-Smad3/Smad3 in different groups. All data were represented as the mean ± SD from three independent experiments. **P < 0.01 compared with control group, #P < 0.05, ##P < 0.01 compared with TGF-β group. | |
Discussion
Pulmonary fibrosis is a chronic and progressive lung disease around the world. Patients with pulmonary fibrosis are often treated with immune suppressive agents combined with cytotoxic agents. However, these treatments can also cause numerous side effects including myelotoxicity and diffuse alveolar hemorrhage.23 Therefore, to develop new drugs with better efficacy and tolerability against pulmonary fibrosis is urgently needed. In our present study, we found PD, a traditional Chinese herbal medicine, can ameliorate pulmonary fibrosis through suppressing inflammation and EMT via inhibiting TGF-β/Smad signaling pathway.
Although there are several pulmonary fibrosis animal models induced by drag, radiation and bronchiolitis obliterans, it is still unclear whether these models can simulate the pulmonary fibrosis which is commonly seen in humans. However, the mouse model of bleomycin-induced pulmonary fibrosis which can represent human pulmonary fibrosis pathogenesis has gained much attention. The intratracheal bleomycin application promotes the release of pro-inflammatory cytokines including TNF-α, IL-1β and IL-6 and induces acute lung inflammation.24 Hence, we used bleomycin-induced pulmonary fibrosis mouse model in our present study.
Human pulmonary fibrosis is characterized by fibroblast proliferation, excessive collagen accumulation and other deposition of ECM proteins in the pulmonary interstitial. Here we observed bleomycin treatment resulted in alveolar wall thickening and massive infiltration of leukocytes in the interstitium, resulting in high alveolitis scores. Up-regulation of TGF-β1 contributes to the pathogenesis of bleomycin-induced pulmonary fibrosis.25,26 During pulmonary fibrosis, fibroblasts and myofibroblasts were activated and could secrete collagens including collagen I and III, resulting in tissue remodeling and fibrosis formation.27 PICP and PIIINP are widely recognized as biomarkers of fibrotic disease and are elevated in pulmonary fibrosis.28 In agreement with previous studies, we found that bleomycin treatment increased the expression of TGF-β1, collagen I, collagen III and production of PICP and PIIINP significantly, suggesting that bleomycin-induced pulmonary fibrosis animal model was conducted successfully in our study.
PD, which is a traditional Chinese herbal medicine abound in grape juices, has been found to exert multiple biological activities including anti-inflammation, anti-oxidation and multiple-organ protection. Xu reported that PD attenuated D-galactose-induced liver injury.29 PD also alleviated radiation-induced lung injury through activation of Sirt3 and inhibition of EMT.12 Here, we observed that PD treatment ameliorated bleomycin-induced pulmonary fibrosis through reducing alveolitis scores, the expression of TGF-β1, collagen I, collagen III and production of PICP and PIIINP compared with model group, indicating that PD might be an effective agent to relieve pulmonary fibrosis.
The development of lung fibrosis is preceded by an increase accumulation of inflammatory cells within the lung parenchyma and inflammation cytokines play important roles in the initiation and progression of pulmonary fibrosis. Pulmonary fibrosis is associated with expression of inflammatory mediators such as TNF-α, IL-1β and IL-17.30–32 Similar to these researches, we observed increased TNF-α, IL-1β, IL-6 and IL-17 production in bleomycin-induced pulmonary fibrosis while PD inhibited the increased production of these inflammation cytokines induced by bleomycin. Specially, the level of pro-inflammatory factor (TNF-α) was strongly reduced by PD, this was in accordance with many other reports.10,33,34 Besides, the adding of TNF-α counteracted PD-induced NLRP3 inflammasome inactivation via enhancing NF-κB pathway.35 These researches hent that PD may interact with TNF-α. This speculation will be examined in our next research. Our results demonstrated that PD relieved pulmonary fibrosis through suppressing inflammation.
Previous study demonstrated that alveolar epithelial cells acquire the phenotype of fibroblasts through EMT to promote the development of pulmonary fibrosis.9,36 EMT occurred in the lung during fibrogenesis and suppressing EMT can attenuate pulmonary fibrosis.37 TGF-β is considered as one of the most potent inducers of fibroblast activation and pulmonary fibrosis pathogenesis.38 In our in vitro experiments, TGF-β was used to induce fibrosis in HFL-1 cells. We found that TGF-β induced EMT in HFL-1 cells through promoting cell migration, decreasing E-cadherin expression and increasing Vimentin expression. However, PD suppressed TGF-β induced EMT in HFL-1 cells in a dose-dependent manner, indicating that PD relieved pulmonary fibrosis through suppressing EMT.
TGF-β initiates tissue fibrosis progression mainly via the Smad2 and Smad3 signaling pathways.39 TGF-β can promote Smad2/3 phosphorylation, which then enhances the combination with Smad4. The Smad2/3/4 complex moves to the nucleus and regulates the transcription of ECM genes, thus enhancing collagen and fibronectin synthesis and promoting pulmonary fibrosis development.40 In the present study, we also observed that TGF-β increased the ratios of p-Smad2/Smad2 and p-Smad3/Smad3, suggesting that TGF-β induces fibrosis in HFL-1 cells through activating TGF-β/Smad signaling pathway. PD was found to block TGF-β/Smad signaling pathway in TGF-β treated HFL-1 cells dose-dependently. Apart from TGF-β pathway, the inhibition of PERK signaling and NF-κB signaling pathway were also involved in the suppression of inflammation and fibrosis in lung.41 The expression of these pathway proteins treated by PD will be measured in our next research.
Conclusion
Taken together, the present study demonstrated that PD treatment attenuated bleomycin-induced pulmonary fibrosis in a dose-dependant manner. The beneficial effect of PD was possibly related to the inhibition of inflammation and EMT through suppressing of TGF-β/Smad signaling pathway. Our finding suggested that PD might be a potential therapeutic candidate in the treatment of pulmonary fibrosis.
Conflicts of interest
There are no conflicts to declare.
References
- G. Raghu, H. R. Collard, J. J. Egan, F. J. Martinez, J. Behr, K. K. Brown, T. V. Colby, J. F. Cordier, K. R. Flaherty and J. A. Lasky, et al., An official ATS/ERS/JRS/ALAT statement: idiopathic pulmonary fibrosis: evidence-based guidelines for diagnosis and management, Am. J. Respir. Crit. Care Med., 2011, 183(6), 788–824 CrossRef PubMed.
- T. J. Gross and G. W. Hunninghake, Idiopathic pulmonary fibrosis, N. Engl. J. Med., 2001, 345(7), 517–525 CrossRef CAS PubMed.
- C. G. Lee, H. R. Kang, R. J. Homer, G. Chupp and J. A. Elias, Transgenic modeling of transforming growth factor-beta(1): role of apoptosis in fibrosis and alveolar remodeling, Proc. Am. Thorac. Soc., 2006, 3(5), 418–423 CrossRef CAS PubMed.
- I. E. Fernandez and O. Eickelberg, The impact of TGF-beta on lung fibrosis: from targeting to biomarkers, Proc. Am. Thorac. Soc., 2012, 9(3), 111–116 CrossRef CAS PubMed.
- R. Samarakoon, J. M. Overstreet and P. J. Higgins, TGF-beta signaling in tissue fibrosis: redox controls, target genes and therapeutic opportunities, Cell. Signalling, 2013, 25(1), 264–268 CrossRef CAS.
- H. Higashiyama, D. Yoshimoto, T. Kaise, S. Matsubara, M. Fujiwara, H. Kikkawa, S. Asano and M. Kinoshita, Inhibition of activin receptor-like kinase 5 attenuates bleomycin-induced pulmonary fibrosis, Exp. Mol. Pathol., 2007, 83(1), 39–46 CrossRef CAS PubMed.
- E. S. White, M. H. Lazar and V. J. Thannickal, Pathogenetic mechanisms in usual interstitial pneumonia/idiopathic pulmonary fibrosis, J. Pathol., 2003, 201(3), 343–354 CrossRef CAS PubMed.
- X. F. Hua, X. H. Li, M. M. Li, C. Y. Zhang, H. J. Liu, T. Sun, H. G. Zhou and C. Yang, Doxycycline attenuates paraquat-induced pulmonary fibrosis by downregulating the TGF-beta signaling pathway, J. Thorac. Dis., 2017, 9(11), 4376–4386 CrossRef PubMed.
- B. C. Willis, J. M. Liebler, K. Luby-Phelps, A. G. Nicholson, E. D. Crandall, R. M. du Bois and Z. Borok, Induction of epithelial-mesenchymal transition in alveolar epithelial cells by transforming growth factor-beta1: potential role in idiopathic pulmonary fibrosis, Am. J. Pathol., 2005, 166(5), 1321–1332 CrossRef CAS PubMed.
- J. Ye, H. Piao, J. Jiang, G. Jin, M. Zheng, J. Yang, X. Jin, T. Sun, Y. H. Choi and L. Li, et al., Polydatin inhibits mast cell-mediated allergic inflammation by targeting PI3K/Akt, MAPK, NF-kappaB and Nrf2/HO-1 pathways, Sci. Rep., 2017, 7(1), 11895 CrossRef PubMed.
- L. Chen and Z. Lan, Polydatin attenuates potassium oxonate-induced hyperuricemia and kidney inflammation by inhibiting NF-kappaB/NLRP3 inflammasome activation via the AMPK/SIRT1 pathway, Food Funct., 2017, 8(5), 1785–1792 RSC.
- K. Cao, X. Lei, H. Liu, H. Zhao, J. Guo, Y. Chen, Y. Xu, Y. Cheng, C. Liu and J. Cui, et al., Polydatin alleviated radiation-induced lung injury through activation of Sirt3 and inhibition of epithelial-mesenchymal transition, J. Cell. Mol. Med., 2017, 21(12), 3264–3276 CrossRef CAS.
- D. Yang, W. Yuan, C. Lv, N. Li, T. Liu, L. Wang, Y. Sun, X. Qiu and Q. Fu, Dihydroartemisinin suppresses inflammation and fibrosis in bleomycine-induced pulmonary fibrosis in rats, Int. J. Clin. Exp. Pathol., 2015, 8(2), 1270–1281 CAS.
- M. J. Wu, X. Gong, R. Jiang, L. Zhang, X. H. Li and J. Y. Wan, Polydatin protects against lipopolysaccharide-induced fulminant hepatic failure in D-galactosamine-sensitized mice, Int. J. Immunopathol. Pharmacol., 2012, 25(4), 923–934 CrossRef CAS PubMed.
- Q. Fu, Y. Tao, H. Piao, M. R. Du and D. J. Li, Trophoblasts and decidual stromal cells regulate decidual NK cell functions via interaction between collagen and LAIR-1, Am. J. Reprod. Immunol., 2014, 71(4), 368–378 CrossRef CAS PubMed.
- V. Milovic, I. C. Teller, G. M. Murphy, W. F. Caspary and J. Stein, Deoxycholic acid stimulates migration in colon cancer cells, Eur. J. Gastroenterol. Hepatol., 2001, 13(8), 945–949 CrossRef CAS PubMed.
- X. Li, G. An, Y. Wang, D. Liang, Z. Zhu and L. Tian, Targeted migration of bone marrow mesenchymal stem cells inhibits silica-induced pulmonary fibrosis in rats, Stem Cell Res. Ther., 2018, 9(1), 335 CrossRef PubMed.
- H. Liu, Y. He, Z. Jiang, S. Shen, J. Mei and M. Tang, Prodigiosin Alleviates Pulmonary Fibrosis Through Inhibiting miRNA-410 and TGF-beta1/ADAMTS-1 Signaling Pathway, Cell. Physiol. Biochem., 2018, 49(2), 501–511 CrossRef CAS PubMed.
- N. C. Mishra, J. Rir-sima-ah, G. R. Grotendorst, R. J. Langley, S. P. Singh, S. Gundavarapu, W. M. Weber, J. C. Pena-Philippides, M. R. Duncan and M. L. Sopori, Inhalation of sulfur mustard causes long-term T cell-dependent inflammation: possible role of Th17 cells in chronic lung pathology, Int. Immunopharmacol., 2012, 13(1), 101–108 CrossRef CAS PubMed.
- S. Turini and L. Bergandi, Epithelial to Mesenchymal Transition in Human Mesothelial Cells Exposed to Asbestos Fibers: Role of TGF-beta as Mediator of Malignant Mesothelioma Development or Metastasis via EMT Event, Int. J. Mol. Sci., 2019, 20(1), E150 CrossRef PubMed.
- L. A. Griggs, N. T. Hassan, R. S. Malik, B. P. Griffin, B. A. Martinez, L. W. Elmore and C. A. Lemmon, Fibronectin fibrils regulate TGF-beta1-induced Epithelial-Mesenchymal Transition, Matrix Biol., 2017, 60–61, 157–175 CrossRef CAS PubMed.
- D. Liang, Y. Wang, Z. Zhu, G. Yang, G. An, X. Li, P. Niu, L. Chen and L. Tian, BMP-7 attenuated silica-induced pulmonary fibrosis through modulation of the balance between TGF-beta/Smad and BMP-7/Smad signaling pathway, Chem.-Biol. Interact., 2016, 243, 72–81 CrossRef CAS PubMed.
- D. Perri, D. E. Cole, O. Friedman, E. Piliotis, S. Mintz and N. K. Adhikari, Azathioprine and diffuse alveolar haemorrhage: the pharmacogenetics of thiopurine methyltransferase, Eur. Respir. J., 2007, 30(5), 1014–1017 CrossRef CAS PubMed.
- Y. Shimizu, K. Dobashi, K. Iizuka, T. Horie, K. Suzuki, H. Tukagoshi, T. Nakazawa, Y. Nakazato and M. Mori, Contribution of small GTPase Rho and its target protein rock in a murine model of lung fibrosis, Am. J. Respir. Crit. Care Med., 2001, 163(1), 210–217 CrossRef CAS PubMed.
- A. Bergeron, P. Soler, M. Kambouchner, P. Loiseau, B. Milleron, D. Valeyre, A. J. Hance and A. Tazi, Cytokine profiles in idiopathic pulmonary fibrosis suggest an important role for TGF-beta and IL-10, Eur. Respir. J., 2003, 22(1), 69–76 CrossRef CAS PubMed.
- N. J. Kenyon, R. W. Ward, G. McGrew and J. A. Last, TGF-beta1 causes airway fibrosis and increased collagen I and III mRNA in mice, Thorax, 2003, 58(9), 772–777 CrossRef CAS.
- S. Ghatak, R. R. Markwald, V. C. Hascall, W. Dowling, R. G. Lottes, J. E. Baatz, G. Beeson, C. C. Beeson, M. A. Perrella and V. J. Thannickal, et al., Transforming growth factor beta1 (TGFbeta1) regulates CD44V6 expression and activity through extracellular signal-regulated kinase (ERK)-induced EGR1 in pulmonary fibrogenic fibroblasts, J. Biol. Chem., 2017, 292(25), 10465–10489 CrossRef CAS PubMed.
- L. Lammi, L. Ryhanen, E. Lakari, J. Risteli, P. Paakko, K. Kahlos, S. Lahde and V. Kinnula, Type III and type I procollagen markers in fibrosing alveolitis, Am. J. Respir. Crit. Care Med., 1999, 159(3), 818–823 CrossRef PubMed.
- L. Q. Xu, Y. L. Xie, S. H. Gui, X. Zhang, Z. Z. Mo, C. Y. Sun, C. L. Li, D. D. Luo, Z. B. Zhang and Z. R. Su, et al., Polydatin attenuates d-galactose-induced liver and brain damage through its anti-oxidative, anti-inflammatory and anti-apoptotic effects in mice, Food Funct., 2016, 7(11), 4545–4555 RSC.
- P. F. Piguet, C. Ribaux, V. Karpuz, G. E. Grau and Y. Kapanci, Expression and localization of tumor necrosis factor-alpha and its mRNA in idiopathic pulmonary fibrosis, Am. J. Pathol., 1993, 143(3), 651–655 CAS.
- M. Kolb, P. J. Margetts, D. C. Anthony, F. Pitossi and J. Gauldie, Transient expression of IL-1beta induces acute lung injury and chronic repair leading to pulmonary fibrosis, J. Clin. Invest., 2001, 107(12), 1529–1536 CrossRef CAS PubMed.
- M. S. Wilson, S. K. Madala, T. R. Ramalingam, B. R. Gochuico, I. O. Rosas, A. W. Cheever and T. A. Wynn, Bleomycin and IL-1beta-mediated pulmonary fibrosis is IL-17A dependent, J. Exp. Med., 2010, 207(3), 535–552 CrossRef CAS PubMed.
- G. Zhao, K. Jiang, H. Wu, C. Qiu, G. Deng and X. Peng, Polydatin reduces Staphylococcus aureus lipoteichoic acid-induced injury by attenuating reactive oxygen species generation and TLR2-NFkappaB signalling, J. Cell. Mol. Med., 2017, 21(11), 2796–2808 CrossRef CAS.
- E. Gugliandolo, R. Fusco, F. Biundo, R. D'Amico, F. Benedetto, R. Di Paola and S. Cuzzocrea, Palmitoylethanolamide and Polydatin combination reduces inflammation and oxidative stress in vascular injury, Pharmacol. Res., 2017, 123, 83–92 CrossRef CAS.
- J. Zou, Y. Yang, Y. Yang and X. Liu, Polydatin suppresses proliferation and metastasis of non-small cell lung cancer cells by inhibiting NLRP3 inflammasome activation via NF-kappaB pathway, Biomed. Pharmacother., 2018, 108, 130–136 CrossRef CAS PubMed.
- K. K. Kim, M. C. Kugler, P. J. Wolters, L. Robillard, M. G. Galvez, A. N. Brumwell, D. Sheppard and H. A. Chapman, Alveolar epithelial cell mesenchymal transition develops in vivo during pulmonary fibrosis and is regulated by the extracellular matrix, Proc. Natl. Acad. Sci. U. S. A., 2006, 103(35), 13180–13185 CrossRef CAS PubMed.
- Y. C. Wang, J. S. Liu, H. K. Tang, J. Nie, J. X. Zhu, L. L. Wen and Q. L. Guo, miR221 targets HMGA2 to inhibit bleomycin induced pulmonary fibrosis by regulating TGFbeta1/Smad3-induced EMT, Int. J. Mol. Med., 2016, 38(4), 1208–1216 CrossRef CAS.
- Q. Liu, H. Chu, Y. Ma, T. Wu, F. Qian, X. Ren, W. Tu, X. Zhou, L. Jin and W. Wu, et al., Salvianolic Acid B Attenuates Experimental Pulmonary Fibrosis through Inhibition of the TGF-beta Signaling Pathway, Sci. Rep., 2016, 6, 27610 CrossRef CAS PubMed.
- F. Qian, M. He, W. Duan, L. Mao, Q. Li, Z. Yu, Z. Zhou and Y. Zhang, Cross regulation between hypoxia-inducible transcription factor-1alpha (HIF-1alpha) and transforming growth factor (TGF)-ss1 mediates nickel oxide nanoparticles (NiONPs)-induced pulmonary fibrosis, Am. J. Transl. Res., 2015, 7(11), 2364–2378 CAS.
- F. Xu, C. Liu, D. Zhou and L. Zhang, TGF-beta/SMAD Pathway and Its Regulation in Hepatic Fibrosis, J. Histochem. Cytochem., 2016, 64(3), 157–167 CrossRef CAS.
- Q. Zheng, M. Tong, B. Ou, C. Liu, C. Hu and Y. Yang, Isorhamnetin protects against bleomycin-induced pulmonary fibrosis by inhibiting endoplasmic reticulum stress and epithelial-mesenchymal
transition, Int. J. Mol. Med., 2019, 43(1), 117–126 Search PubMed.
|
This journal is © The Royal Society of Chemistry 2019 |
Click here to see how this site uses Cookies. View our privacy policy here.