DOI:
10.1039/C8RA10004G
(Paper)
RSC Adv., 2019,
9, 6717-6723
Development of a HPLC-FL method to determine benzaldehyde after derivatization with N-acetylhydrazine acridone and its application for determination of semicarbazide-sensitive amine oxidase activity in human serum
Received
5th December 2018
, Accepted 15th February 2019
First published on 26th February 2019
Abstract
A novel fluorescence labeling reagent N-acetylhydrazine acridone (AHAD) was designed and synthesized. A highly sensitive high performance liquid chromatography (HPLC) method coupled with fluorescence detection to determine benzaldehyde after derivatization with AHAD was developed. Optimum derivatization was obtained at 40 °C for 30 min with trichloroacetic acid as catalyst. Benzaldehyde derivative was separated on a reversed-phase SB-C18 column in conjunction with a gradient elution and detected by fluorescence detection at excitation and emission wavelengths of 371 nm and 421 nm. The established method exhibited excellent linearity over the injected amount of benzaldehyde of 0.003 to 5 nmol mL−1. The method was successfully applied to the determination of serum semicarbazide-sensitive amine oxidase (SSAO) activity in humans. SSAO is a significant biomarker because serum SSAO activity is elevated in patients with Alzheimer's disease, vascular disorders, heart disease and diabetes mellitus. It was demonstrated that the SSAO activity of the hyperglycemic group (60 ± 4 nmol mL−1 h−1) was significantly higher than that of normal blood sugar group (44 ± 4 nmol mL−1 h−1) with P < 0.05.
1. Introduction
Benzaldehyde widely exists in Rosaceae plants. It has a special odor of bitter almond and is used as an additive in food industry. Benzaldehyde also has been used in the determination of semicarbazide-sensitive amine oxidase (SSAO) activity, because it is an important product of benzylamine oxidized and deaminated by the enzyme. SSAO activity could be indirectly measured by determinating the content of benzaldehyde with benzylamine as the substrate of enzyme reaction. SSAO is a group of copper-containing enzymes which are sensitive to semicarbazide and other hydrazines.1 These enzymes exist in vascular smooth muscle cells, cartilage, kidney, liver and brown fat cell of mammalian,2,3 and they are also found in serum.4 Various amine compounds including methylamine, allylamine, aminoacetone, dihydroxybenzylamine and benzylamine could be oxidized and deaminated by SSAO to generate corresponding aldehydes, ammonia and hydrogen peroxide1,2 which are all potentially cytotoxic and commonly associated with numerous diseases.5 It has been reported that serum SSAO activity is elevated in patients with Alzheimer's disease,6,7 vascular disorders and heart disease.8–10 Serum SSAO activity of diabetics has also been found to be obviously higher than those with normal blood sugar.11–13 Therefore, SSAO activity could be used as an important biomarker for diabetic angiopathy and other diseases.14
Numerous methods for the determination of SSAO activity have been reported. In these methods, Radio-enzymatic assay (REA) was used by many studies.15–20 Radiometric method can give accurate data about SSAO activity, but radioactive compound [14C]-benzylamine is used in the method. LC-MS can determine the content of SSAO directly in samples,21,22 but the instrument of the method is sophisticated and expensive. Other methods employed to the determination of SSAO activity are light scattering (LS)23 and HPLC.24–27 In these methods, benzylamine is usually used as the preferred substrate that is oxidized and deaminated by SSAO into benzaldehyde, and then benzaldehyde reacted with labeling reagent to form the corresponding hydrazone. In the method of LS, benzaldehyde was labeled by 2,4-dinitrophenylhydrazine (DNPH) and the derivative can enhance light scattering intensity. The method based on the change of light scattering facilitated the assessment of SSAO activity in vitro sensitively. But for blood plasma sample, the complex matrix would interfere with the determination of benzaldehyde for the poor selectivity of the method. Furthermore, the accuracy of SSAO activity measurement would be decreased. To overcome the disadvantage, HPLC with UV detection was utilized for the activity determination of SSAO.24 HPLC is the method with best selectivity, and it can effectively eliminate the influence of coexisting substances in biological samples to the determination of benzaldehyde. In order to further improve method sensitivity, benzaldehyde was derivatized by fluorescence labeling reagent such as dimedone25–27 and the derivative was determined by HPLC with fluorescence detection. However, the reaction of benzaldehyde with dimedone was performed at a higher temperature (95 °C) for 45 min.25 As a result, a fast, safe and sensitive method for the determination of serum SSAO activity is needed.
In this study, we designed and synthesized a novel fluorescence labeling reagent N-acetylhydrazine acridone (AHAD) which can emit strong fluorescence (λem = 421 nm) when exposed to excitation light (λem = 371 nm). AHAD can react with carbonyl compounds in the presence of a acid catalyst, and a highly sensitive method for the analysis of benzaldehyde using AHAD as labeling reagent was developed. The optimum derivatization of benzaldehyde with AHAD was obtained at 40 °C for 30 min with trichloroacetic acid as catalyst. Benzaldehyde reacted with AHAD to form the corresponding hydrazone quantitatively. AHAD is stable enough for derivatization and the derivative was also stable enough for chromatographic analysis. The developed method was successfully applied to determine SSAO activity in human serum with benzylamine as substrate.
2. Experimental sections
2.1 Chemicals and reagents
Benzylamine and benzaldehyde were purchased from Shanghai Aladdin Bio-Chem Technology Co. Ltd. (Shanghai, China). Benzaldehyde was acquired from China National Pharmaceutical Group Corporation (Shanghai, China). Clorgiline was obtained from Sigma-Aldrich (St. Louis, MO, USA). AHAD was synthesized in our laboratory. MilliQ water was used throughout the experiment after filtering through a 0.22 μm nylon membrane. Chromatography-grade acetonitrile was obtained from Yucheng Chemical Reagent Co. (Shandong Province, China). Other reagents such as hydrochloric acid, sodium hydroxide, dimethylformamide, potassium carbonate, phosphoric acid, acetic acid, sulfuric acid, ethyl alcohol, hydrazine hydrate and trichloroacetic acid of analytical grade were supplied by Sinopharm Chemical Reagent Co. Ltd. (Shanghai, China).
2.2 Instrumentation and conditions
Experiments were performed using Agilent 1260 series high performance liquid chromatography (Agilent Technologies, Waldbronn, Germany). The HPLC system was equipped with a quaternary pump (model G1311B), an autosampler (model G1329B), a thermostated column compartment (model G1316A), and a fluorescence detector (FLD) (model G1321B). The HPLC system was controlled by Agilent Chemstation software. Derivatives were separated on a SB-C18 column (150 mm × 4.6 mm, 5 μm i.d., Agilent, USA).
The chromatographic separation of the derivative was accomplished using gradient elution. Solvent A was water and B was 100% acetonitrile. The gradient condition of the mobile phase was as follows: 30–100% B from 0 to 15 min and then held for 5 min. The flow rate was constant at 1.0 mL min−1 and the column temperature was kept at 30 °C. The injection volume was 20 μL and the excitation and emission wavelengths were set at 371 nm and 421 nm, respectively.
2.3 Synthesis of AHAD
2.3.1 Synthesis of 2-(9-acridone)-acetic acid. Acridone (9.76 g, 50 mmol) was dissolved in dimethylformamide (150 mL) and anhydrous potassium carbonate (30 g) was added, the mixture was stirred at 90 °C for 30 min. Then, ethyl chloroacetate (10.6 mL, 200 mmol) dissolved in dimethylformamide (20 mL) was added dropwise within 2 h. The mixture solution was stirred at 90 °C for 20 h. After cooled to room temperature, the solution was filtered and potassium hydroxide solution (200 mL, 10%) was added to the filter liquor. Then the solution was heated to boiling for 2 h. At the end of the reaction, the pH of the solution was adjusted to 2–3 using HCl and the precipitate was filtered off and washed with water-methanol (20 mL, 9
:
1) for 3 times. The crude product was recrystallized from acetic acid to yield brown crystal (9.12 g, 72.1% yield).
2.3.2 Synthesis of 2-(9-acridone)-ethyl acetate. Ethyl alcohol (100 mL), 2-(9-acridone)-acetic acid (6 g) and concentrated sulfuric acid (5 mL) were added to a 250 mL flask successively. The mixture was heated to reflux with stirring for 5 h. The solution was poured into ice water (400 mL) with shaking for 5 min and filtered using HPLC filter paper. The crude product was recrystallized from methanol to yield yellow crystal (5.8 g, 85.6% yield).
2.3.3 Synthesis of N-acetylhydrazine acridone. Ethyl alcohol (30 mL), 2-(9-acridone)-ethyl acetate (2 g) and hydrazine hydrate (10 mL, 85%) were added to a 100 mL flask successively. The mixture was heated to reflux with stirring for 3 h. The solution was poured into ice water (200 mL) with shaking for 5 min and filtered using HPLC filter paper. The crude product was recrystallized from methanol to obtain yellow crystal (1.2 g, 65.9% yield). Found (%): C 67.41, H 4.88, N 15.77, O 11.94; calculated (%): C 67.40, H 4.90, N 15.72, O 11.97.1H NMR (500 MHz, DMSO) δ 8.37 (d, J = 7.8 Hz, 1H), 7.82 (t, J = 7.5 Hz, 1H), 7.65 (d, J = 8.7 Hz, 1H), 7.37 (t, J = 7.4 Hz, 1H), 5.18 (s, 1H), 4.40 (s, 1H). m/z [M + H]: 268.19.
2.4 Preparation of solutions
The standard stock solution (1 × 10−2 mol L−1) of benzaldehyde was prepared by dissolving 102 μL of benzaldehyde with acetonitrile up to 100 mL. The standard solutions for derivatization and HPLC analysis were prepared by diluting the stock solution with acetonitrile. Phosphate buffer solution (PBS, 1 × 10−4 mol L−1, pH 7.8) was prepared by dissolving 0.13 g of Na2HPO4 and 0.11 g of NaH2PO4 in 100 mL water and a Mettler-Toledo FE20 pH-meter was used to check the pH of the buffer. Stock solutions of clorgiline (2 × 10−3 mol L−1) and benzylamine (5.6 × 10−3 mol L−1) were prepared in PBS. The AHAD solution (1 × 10−2 mol L−1) was prepared by dissolving 26.8 mg AHAD with acetonitrile up to 10 mL. The corresponding low concentration of AHAD solution (5 × 10−4 mol L−1) was diluted by acetonitrile. All solutions were sealed and stored at 4 °C until analysis.
2.5 Serum samples
Twelve human serum samples were supplied by Yantaishan Hospital and stored at −80 °C before analyzed. All serum samples were divided into two groups which were matched by blood glucose level. One was normal blood sugar group (6 samples) and another was hyperglycemia group (6 samples). Blood glucose level was determined by the supplier of serum samples. All experiments were performed in accordance with the ethical standards in the Helsinki declaration, and approved by the ethics committee at Binzhou Medical university. Informed consents were obtained from human participants of this study.
2.6 Sample preparation procedure for SSAO enzyme activity
Serum sample was prepared according to a previous procedure28 with a minor modification. An aliquot of 50 μL human serum was pipetted into a 1.5 mL centrifuge tube. Then, 100 μL of clorgiline (2 × 10−3 mol L−1) was added to inhibit the monoamine oxidases (MAO-A and MAO-B) activity in serum. After vortexed for 1 min, the mixture was incubated in a thermostatically controlled water bath at 37 °C for 30 min, then. 250 μL of benzylamine (5.6 × 10−3 mol L−1) was added to the tube and the final concentration of benzylamine was 3.5 × 10−3 mol L−1, then the tube was vortexed for 1 min and incubated at 37 °C for 1 h. Then, 400 μL acetonitrile was added to the mixture, then the tube was vortexed for 1 min and centrifuged at 5000 rpm for 10 min. The supernatant was collected into a 2 mL ampoule and evaporated to dryness at 40 °C under a gentle stream of nitrogen. The residue was dissolved in 50 μL acetonitrile and derivatized by AHAD.
2.7 Derivatization procedure of AHAD with benzaldehyde
50 μL of standard benzaldehyde solution or supernatant, 350 μL of 5 × 10−4 mol L−1 AHAD solution and 60 μL of trichloroacetic acid (0.1 mol L−1) were added into a 2 mL ampoule, successively. The ampoule was sealed and bathed in a thermostatically controlled water bath at 40 °C for 30 min. After derivatization, 40 μL of 50% acetonitrile was added to dilute the derivatization solution to 500 μL. The final solution was injected directly for HPLC analysis without any other operations. The derivatization scheme of AHAD with benzaldehyde is shown in Fig. 1.
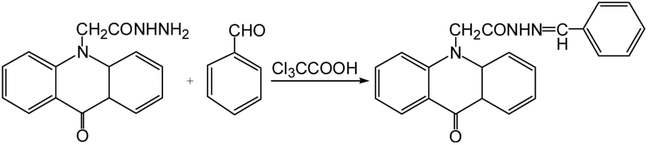 |
| Fig. 1 Derivatization scheme of AHAD with benzaldehyde. | |
3. Results and discussion
3.1 Fluorescence spectra AHAD-benzaldehyde derivative
The fluorescence spectra of AHAD-benzaldehyde derivative was determined at the wavelength range of 250 to 550 nm to find fluorometric detector wavelength. As was shown in Fig. 2, the maximum wavelengths of AHAD-benzaldehyde derivative in purity acetonitrile were 371 nm for excitation and 409 nm for emission, respectively. There was no obvious wavelength shift for the excitation spectra with the decreasing of the volume ratio of acetonitrile in the mixed solvents. But for emission spectra, the maximum emission wavelength in 90% acetonitrile was 417 nm, and a continuous red-shift was observed with the decreasing of the volume ratio of acetonitrile. In the study, when AHAD-benzaldehyde derivative flowed into the flow cell, the volume ratio of acetonitrile in the mobile phase was about 60%, so 371 nm and 421 nm was select as detection wavelengths for further experiments. The fluorescence intensity of AHAD-benzaldehyde derivative increased with the decrease of acetonitrile ratio, and a 70% increase was found when acetonitrile ratio varied from 100% to 50%.
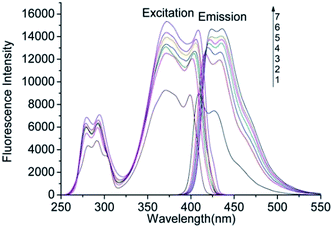 |
| Fig. 2 Excitation and emission spectra of AHAD-benzaldehyde derivative in different mixed solvents. (1) 100% acetonitrile, (2) 90% acetonitrile, (3) 80% acetonitrile, (4) 70% acetonitrile, (5) 60% acetonitrile, (6) 50% acetonitrile, (7) 40% acetonitrile. | |
3.2 Optimization of derivatization conditions
In order to obtain the highest yield for the derivatization of benzaldehyde with AHAD, effects of species and amount of catalyst, reaction temperature, reaction time, amount of AHAD on the reaction were investigated.
AHAD can react with benzaldehyde in the presence of acid. Effect of different acids on the reaction was investigated. These acids include formic acid, oxalic acid, acetic acid, citric acid, and trichloroacetic acid. The concentration of all acids was 0.10 mol L−1. The result (Fig. 3a) showed that the highest detection response was achieved when trichloroacetic acid was used as the catalyst of the derivatization. For oxalic acid and citric acid, the peak area was slightly smaller than trichloroacetic acid, so trichloroacetic acid was selected as the catalyst for subsequent tests. Then, the effect of trichloroacetic acid volume on derivatization was studied over the range of 20–100 μL. The maximum peak area was obtained when 60 μL of trichloroacetic acid was used in the derivatization (Fig. 3b).
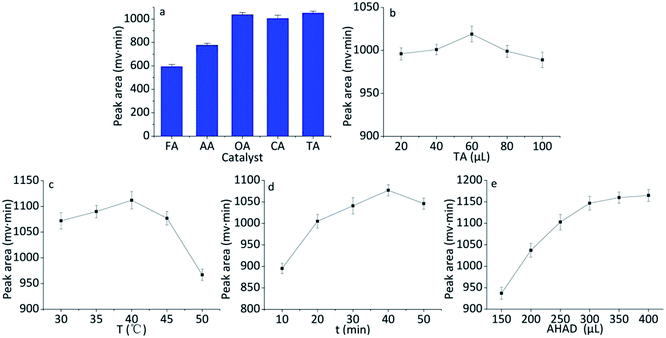 |
| Fig. 3 Effect of different reaction conditions on peak area of the reaction product of AHAD with benzaldehyde. (a) Effect of catalyst species, (b) effect of amount of catalyst, (c) effect of reaction time, (d) effect of reaction temperature, (e) effect of amount of AHAD. | |
The effect of temperature on derivatization of benzaldehyde with AHAD was examined over the range of 30–50 °C. When raising reaction temperature, the detection response of derivative increased, and the maximum peak area of benzaldehyde derivative was obtained at 40 °C (Fig. 3c). There was an obvious change for detection response over 40 °C which could be caused by the decomposition of derivative. The effect of time (over the range of 10–50 min) on the derivatization reaction was also investigated. The maximum detection response was obtained at 40 min, and the rise of reaction time lead to a slight loss of peak intensity (Fig. 3d). So reaction time was controlled at 40 min.
The amount of AHAD was tested to ensure the sufficient reaction of benzaldehyde with AHAD. The result (Fig. 3e) indicated that maximum detection response was obtained when 350 μL AHAD was used in the derivatization reaction, and further increasing AHAD beyond this volume had no significant change on detection response. Therefore, 350 μL AHAD was chosen for subsequent experiments and the derivatization action was completed in 40 min.
3.3 Stability of AHAD and AHAD-benzaldehyde
Solution of AHAD in acetonitrile was stable enough when it was stored at 4 °C, and there was no obvious change for the derivatization yield after seven days. The stability of the AHAD-benzaldehyde derivative was tested by re-analysis of the same sample stored at 4 °C for 24 h and at room temperature for 12 h. The percent change of the peak area was less than 3.1% at 4 °C and less than 3.9% at room temperature. This results indicated that the derivative was stable enough for at least 24 h when stored at 4 °C and for 12 h when stored at room temperature.
3.4 Selectivity of the method
In order to evaluate the selectivity of the developed method and apply the method to determine serum SSAO activity, some substances which maybe disturb the separation and quantization of benzaldehyde were investigated. These substances included byproducts in derivatization, endogenous components in serum, and regents used in sample preparation. The derivatization reaction of benzaldehyde with AHAD was occurred in the presence of trichloroacetic acid, and some by-products were found in derivatization procedure besides derivatization product. In addition, excess labeling reagent used in derivatization may also be a possible interference to separation. A derivatization procedure was carried out with 50 μL of acetonitrile instead of standard benzaldehyde solution, and the chromatographic isolation result (Fig. 4a) was compared with the chromatogram of standard benzaldehyde solution (Fig. 4b). The result showed that the retention time of benzaldehyde derivative was 7.82 min, and no peak was found at this time in Fig. 4a. Other peaks including excess AHAD in the two samples' chromatograms were almost the same, and they did not disturb the isolation and determination of benzaldehyde derivative. A blank sample was prepared according to the procedure described above with 250 μL PBS instead of benzylamine and analyzed on the same chromatographic conditions. The result (Fig. 4c) was compared with serum sample (Fig. 4d), and no interference was found. As a result, the byproducts in derivatization, endogenous components in serum, and regents used in sample preparation did not disturb the isolation and determination of AHAD-benzaldehyde derivative, and the proposed method was of highly selectivity.
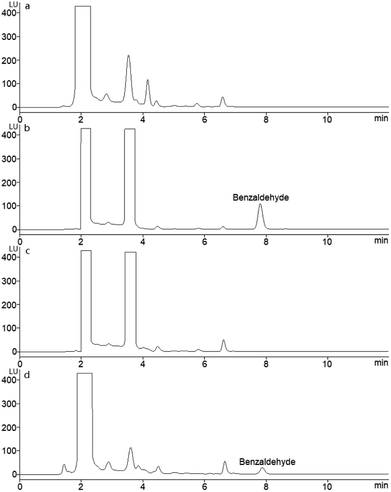 |
| Fig. 4 HPLC chromatograms after applying the procedure described in Section 2.7 (a) acetonitrile blank; (b) standard benzaldehyde (40.0 nmol mL−1); (c) blank serum sample; (d) serum sample. | |
3.5 Linearity and correlation coefficient
The calibration curve was established by the analysis of blank serum samples spiked with standard benzaldehyde. The spiked samples were prepared and derivatized according to the procedure described above. The injected concentration of benzaldehyde varied from 0.003 to 5 nmol mL−1. AHAD-benzaldehyde derivative showed excellent linear relation with correlation coefficient of 0.9997 and the linear regression equation was Y = 238.8X − 0.28 (X: injected concentration per nmol per mL, Y: peak area per mV per min).
3.6 Sensitivity
The calculated LOD with fluorescence detection (at a signal-to-noise ratio of 3
:
1) was 8.9 × 10−3 nmol mL−1 h−1, and LOQ (at a signal-to-noise ratio of 10
:
1) was 0.03 nmol mL−1 h−1. AHAD provides lower LOD values than other derivatizing agents used in UV or fluorescence detection, such as 2,4-dinitrophenylhydrazine24 and dimedone,25 which permit us to determine lower content than methods involving other reagents.
3.7 Precision and accuracy
Instrumental precision and accuracy were investigated by analyzing standard benzaldehyde solution at three concentrations (0.1, 2.0, and 40.0 nmol mL−1), and five determinations were performed for each concentraion. The instrumental show good precision in the range of 0.036–0.059% for retention time and 0.69–0.97% for peak area of benzaldehyde, respectively (see Table 1). The accuracy was calculated according to the equation: accuracy (%) = Ca/Cb × 100%, where Ca is the concentration of benzaldehyde calculated by linear regression equation, and Cb is the concentration of standard benzaldehyde solution. The accuracy was found in the range of 95.8–97.2%. Precision (intra-day and inter-day) of the method were investigated by spiking benzaldehyde at three different concentrations (0.1, 2.0, and 40.0 nmol mL−1) into serum sample. The serum sample was prepared according to the procedure described above using 250 μL of PBS instead of the SSAO's substrate benzylamine before benzaldehyde was spiked. The intra-day precision ranged from 2.5% to 4.9%, while the inter-day precision ranged from 4.2% to 6.9%. The results showed good accuracy and adequate precision for the determination of benzaldehyde in the serum sample.
Table 1 Instrument precision, accuracy, method precision and recovery for the determination of benzaldehyde
Benzaldehyde (nmol mL−1) |
Instrument precision (RSD, %) |
Accuracy (%) |
Method precision (RSD, %) |
Recovery (%) |
Retention time |
Peak area |
Intra-day |
Inter-day |
0.1 |
0.036 |
0.97 |
95.8 |
4.9 |
6.9 |
93.5 |
2.0 |
0.052 |
0.82 |
96.9 |
2.5 |
5.0 |
95.2 |
40.0 |
0.059 |
0.69 |
97.2 |
3.6 |
4.2 |
96.1 |
3.8 Recovery
Recovery experiments were performed by analyzing serum samples spiked with three concentrations of benzaldehyde (0.1, 2.0, and 40.0 nmol mL−1) before sample preparation and after sample preparation according to the procedure described in Section 2.6 with benzylamine replaced by 250 μL of PBS. The recovery was calculated according to the equation: recovery (%) = Aa/Ab × 100%, where Aa and Ab are the peak area values of each spiked concentration before and after sample preparation. The analyses were carried out in triplicate for each concentration, and the experimental recoveries obtained were ranged from 93.5 to 96.1% (Table 1).
3.9 Advantage of the proposed method
Among these methods mentioned above, REA was mostly used by some researchers, however, radioactive compound [14C]-benzylamine was used as substrate for the enzymatic reaction which made researchers exposed to radiological hazards. HPLC24,25 was more sensitive and with higher selectivity. To determine SSAO activity, benzylamine was used as the preferred substrate. Benzylamine was deaminated and oxidized by SSAO into benzaldehyde, and benzaldehyde was labeled by UV or fluorescent labeling reagents. AHAD was used to label benzaldehyde in this work, and the comparison of AHAD with other labeling reagents was listed in Table 2. The main advantage of the method developed using AHAD as labeling reagent was the higher sensitivity compared with other methods. The LOD for SSAO in this study was 8.9 × 10−3 nmol mL−1 h−1 which is lower than DNPH24 and dimedone.25 The proposed method was also more sensitivity than LS23 and FIA-FL28 with 1,2-diaminoanthraqui-none (DAAQ) as labeling reagent. Besides higher sensitivity, there were several other advantages including mild reaction conditions of low temperature and weak acid, broad linear range and high recovery. LC-MS can determine the SSAO activity in samples with higher sensitivity,21,29 but the instrument of this method is sophisticated and expensive.
Table 2 Comparison of the proposed method with other methods for the determination of SSAO activity
Method |
Substrate |
Reagent |
Reaction conditions |
LOD (nmol mL−1 h−1) |
Recovery (%) |
Remarks |
Ref. |
N/A: data is not available. The unit is fmol μL−1. |
REA |
[14C]-benzylamine |
— |
— |
N/Aa |
N/Aa |
Using radioactive compound |
19 |
LS |
Benzylamine |
DNPH |
40 °C, 30 min |
1.92 × 10−3 |
68 |
Poor selectivity and recovery |
23 |
HPLC-UV |
Benzylamine |
DNPH |
40 °C, 15 min, 20% trichloroacetic acid |
0.1 |
63.5–68.2 |
Low sensitivity and recovery |
24 |
HPLC-FL |
Benzylamine |
Dimedone |
95 °C, 45 min, 9 mol L−1 sulphuric acid |
1.5 |
97 |
Harsh reaction conditions |
25 |
FIA-FL |
Benzylamine |
DAAQ |
100 °C, 5 min, 100% acetic acid |
0.06 |
94–98 |
Harsh reaction conditions |
28 |
LC-MS |
— |
— |
— |
1.99b |
75–110 |
Using sophisticated and expensive instrument |
21 |
LC-MS |
Methylamine |
Dopamine |
37 °C, 30 min |
1 |
96–112 |
Using sophisticated and expensive instrument |
29 |
HPLC-FL |
Benzylamine |
AHAD |
40 °C, 30 min, 0.1 mol L−1 trichloroacetic acid |
8.9 × 10−3 |
93–97 |
Mild reaction conditions and high sensitivity |
This work |
3.10 Application
The developed method was utilized to determine the serum SSAO activity of 12 human subjects. Serum SSAO activity is defined as benzaldehyde (nmol) formed per milliliter serum per hour.28 The results of human serum SSAO activity were listed in Table 3. The average SSAO activity (mean ± SE) of normal blood sugar group was 44 ± 4 nmol mL−1 h−1 and hyperglycemia group was 60 ± 4 nmol mL−1 h−1. SE (standard error) is defined as
.30 Statistical analysis between the two groups was performed using “SPSS 19.0” with the independent samples t-test. F-test was performed before t-test, and the result showed that variances of two groups have no significant difference (P = 0.574). Statistical result of t-test revealed that SSAO activity of hyperglycemia group was significantly higher than normal blood sugar group (P < 0.05). These results proved that the SSAO activity may be used as a valuable biomarker for hyperglycemia. SSAO activity value could help doctors to diagnose whether hyperglycemia is present. However, hyperglycemia could not be diagnosed only by SSAO activity value and hardly a disease could be diagnosed only by one factor. SSAO could be used as a useful biomarker for hyperglycemia, but how is it used as biomarker should be further studied.
Table 3 The results for determination of the SSAO activity
Normal blood sugar group |
Hyperglycemia group |
Blood sugar level (mmol L−1) |
SSAO activity (nmol mL−1 h−1) |
Mean activity (nmol mL−1 h−1) |
Blood sugar level (mmol L−1) |
SSAO activity (nmol mL−1 h−1) |
Mean activity (nmol mL−1 h−1) |
(n = sample size). |
5.2 |
49.6 |
44 ± 4a |
7.8 |
68.4 |
60 ± 4a |
4.9 |
35.7 |
8.1 |
72.1 |
4.1 |
29.4 |
6.9 |
57.2 |
5.7 |
56.3 |
9.2 |
50.5 |
5.1 |
38.9 |
8.3 |
61.3 |
4.6 |
51.8 |
9.0 |
48.7 |
4. Conclusions
A novel fluorescence labeling reagent N-acetylhydrazine acridone (AHAD) was designed and synthesized. AHAD which can emit strong fluorescence when exposed to excitation light is an excellent labeling reagent for carbonyl compounds A highly sensitive HPLC-FL method to determine benzaldehyde after derivatization with AHAD was developed. The optimum derivatization conditions of benzaldehyde with AHAD was obtained at 40 °C for 30 min with trichloroacetic acid as catalyst. The developed method showed good correlation, precision and accuracy. The proposed method was utilized successfully to determinate SSAO activity in human serum with a satisfactory result. It is also a accurate and sensitive method to be used for routine analysis of the SSAO activity in serum of diabetic human subjects. AHAD also has the potential to be used for the derivatization and analysis of other carbonyl compounds such as aliphatic aldehydes, aromatic aldehydes and ketones. Further application of AHAD will be carried out in the next work of our laboratory.
Conflicts of interest
There are no conflicts to declare.
References
- V. Castillo, J. M. Lizcano, J. Visa and M. Unzeta, Neurochem. Int., 1998, 33, 415–423 CrossRef CAS PubMed
. - A. Gella, M. Solé, I. Bolea, M. Ventriglia, M. Siotto, N. Durany, R. Squitti and M. Unzeta, J. Neural Transm., 2013, 120, 1015–1018 CrossRef CAS PubMed
. - F. Boomsma, U. M. Bhaggoe, A. M. B. van der Houwen and A. H. van den Meiracker, Biochim. Biophys. Acta, 2003, 1647, 48–54 CrossRef CAS
. - S. F. Nunes, I. V. Figueiredo, P. J. Soares, N. E. Costa, M. C. Lopes and M. M. Caramona, Acta Diabetol., 2009, 46, 135–140 CrossRef CAS PubMed
. - A. S. Januszewski, N. Mason, C. S. Karschimkus, K. G. Rowley, J. D. Best, N. O'Neal David and A. J. Jenkins, Diabetes Vasc. Dis. Res., 2014, 4, 262–269 CrossRef PubMed
. - M. del Mar Hernandez, M. Esteban, P. Szabo, M. Boada and M. Unzeta, Neurosci. Lett., 2005, 384, 183–187 CrossRef PubMed
. - Z. J. Jiang, J. S. Richardson and P. H. Yu, Neuropathol. Appl. Neurobiol., 2008, 34, 194–204 CrossRef CAS PubMed
. - M. Exner, M. Hermann, R. Hofbauer, S. Kapiotis, P. Quehenberger, W. Speiser, I. Held and B. M. K. Gmeiner, Cardiovasc. Res., 2001, 50, 583–588 CrossRef CAS PubMed
. - I. Karádi, Z. Meszáros, A. Csányi, T. Szombathy, N. Hosszúfalusi, L. Romics and K. Magyar, Clin. Chim. Acta, 2002, 323, 139–146 CrossRef
. - S. Becchi, A. Buson, J. Foot, W. Jarolimek and B. W. Balleine, Br. J. Pharmacol., 2017, 174, 2302–2317 CrossRef CAS PubMed
. - H. Garpenstrand, J. Ekblom, L. B. Bäcklund, L. Oreland and U. Rosenqvist, Diabetic Med., 1999, 16, 514–521 CrossRef CAS PubMed
. - S. F. Nunes, I. V. Figueiredo, J. S. Pereira, P. J. Soares, M. M. Caramona and B. Callingham, Acta Diabetol., 2010, 47, 179–182 CrossRef CAS PubMed
. - T. Obata, Life Sciences, 2006, 79, 417–422 CrossRef CAS PubMed
. - P. Mátyus, B. Dajka-halász, Á. Földi, N. Haider, D. Barlocco and K. Magyar, Curr. Med. Chem., 2004, 11, 1285–1298 CrossRef
. - C. Gokturk, J. Nordquist, H. Sugimoto, K. Forsberg-Nilsson, J. Nilsson and L. Oreland, Biochem. Biophys. Res. Commun., 2004, 325, 1013–1020 CrossRef CAS PubMed
. - J. L. E. Nordquist, C. Göktürk and L. Oreland, Mol. Med., 2002, 8, 824–829 CAS
. - A. Abella, S. García-Vicente, N. Viguerie, A. Ros-Baró, M. Camps, M. Palacín, A. Zorzano and L. Marti, Diabetologia, 2004, 47, 429–438 CrossRef CAS PubMed
. - S. García-vicente, A. Abella, N. Viguerie, A. Rosbaró, M. Camps, X. Testar, M. Palacín, A. Zorzano and L. Marti, J. Physiol. Biochem., 2005, 61, 395–402 CrossRef
. - Z. Mészáros, I. Karádi, A. Csányi, T. Szombathy, L. Romics and K. Magyar, Eur. J. Drug Metab. Pharmacokinet., 1999, 24, 299–302 CrossRef PubMed
. - Z. Iffiú-Soltész, D. Prévot and C. Carpéné, J. Physiol. Biochem., 2009, 65, 11–23 CrossRef
. - Y. Zhang, S. Xiao, L. Wang, H. Wang, Y. Zhu, Y. Li and Y. Deng, Anal. Bioanal. Chem., 2010, 397, 709–715 CrossRef CAS PubMed
. - Y. Zhang, C. Lai, J. Duan, N. Guan, K. Ullah and Y. Deng, Appl. Microbiol. Biotechnol., 2012, 94, 809–816 CrossRef CAS PubMed
. - X. Tan, W. Rang, Y. Wang, H. Yang, J. Xue, L. Shi, H. Yang, L. Liu and B. Zhou, Anal. Lett., 2012, 45, 2774–2784 CrossRef CAS
. - H. Li, W. Luo, J. Lin, Z. Lin and Y. Zhang, J. Chromatogr. B: Anal. Technol. Biomed. Life Sci., 2004, 810, 277–282 CrossRef CAS
. - J. van Dijk, F. Boomsma, G. Alberts, A. J. Man in‘t Veld and M. A. D. H. Schalekamp, J. Chromatogr. B: Anal. Technol. Biomed. Life Sci., 1995, 663, 43–50 CrossRef CAS PubMed
. - V. Roessner, A. Weber, A. Becker, G. Beck, J. Kornhuber, H. Frieling and S. Bleich, Prog. Neuro-Psychopharmacol., 2006, 30, 906–909 CrossRef CAS PubMed
. - V. Roessner, A. Weber, A. Becker, G. Beck, H. Frieling and S. Bleich, Eur. J. Clin. Pharmacol., 2007, 63, 425–429 CrossRef CAS PubMed
. - M. H. Elmaghrabey, N. Kishikawa, K. Ohyama, T. Imazato, Y. Ueki and N. Kuroda, Anal. Chim. Acta, 2015, 881, 139–147 CrossRef CAS PubMed
. - L. Wang, Y. Zhang, S. Xiao, G. Hu, B. Che, H. Qing, Y. Li, L. Zhang and Y. Deng, Anal. Methods, 2012, 4, 1383–1388 RSC
. - D. G. Altman and J. M. Bland, BMJ, 2005, 331, 903 CrossRef PubMed
.
|
This journal is © The Royal Society of Chemistry 2019 |
Click here to see how this site uses Cookies. View our privacy policy here.