DOI:
10.1039/C9RA00630C
(Review Article)
RSC Adv., 2019,
9, 14477-14502
Nitroketene N,S-acetals: synergistic building blocks for the synthesis of heterocycles
Received
24th January 2019
, Accepted 17th April 2019
First published on 9th May 2019
Abstract
The development of novel heterocyclic compounds from simple and easily accessible starting components is of significant importance in medicinal chemistry. Due to the presence of active chromophores and potent pharmacological activities, nitroketene N,S-acetals have emerged as a fascinating building block in organic synthesis. The synergistic skeleton of these acetals and the presence of electron-donating as well as electron-withdrawing groups lead to the generation of distinctive structural features and are highly useful for building diverse heterocyclic rings. This review highlights the preparation of different nitroketene N,S-acetals and their applications in the synthesis of diverse heterocyclic compounds.
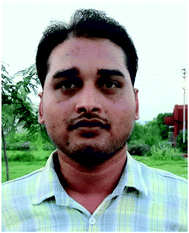 Saigal | Saigal was born in Berhampore in the district of Murshidabad, West Bengal, India, in 1992. He obtained a B.Sc. (Chemistry) in 2012 and an M.Sc. (Organic Chemistry) degree in 2014 from Aligarh Muslim University (AMU). At present, he is pursuing his Ph.D. as a CSIR-SRF research fellow under the supervision of Dr Md. Musawwer Khan at the laboratory of MCR and Carbohydrate Chemistry, Department of Chemistry, AMU, Aligarh, India. His research interest focuses on the green synthesis of nitrogen- and oxygen-containing heterocyclic compounds using different building blocks. |
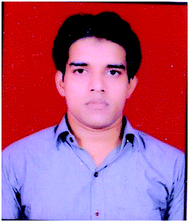 Sarfaraz Khan | Sarfaraz Khan was born in Delhi, India in 1991. He received his B.Sc. (Hons.) in Chemistry from the University of Delhi, India in 2012, and in 2014, he obtained his M.Sc. degree with Organic Chemistry specialization from the same university. Currently, he is pursuing his Ph.D. under the supervision of Dr Md. Musawwer Khan as a UGC-SRF research fellow in the Department of Chemistry, Aligarh Muslim University, India. His research interest focuses on the synthesis of small heterocyclic compounds through multicomponent reactions (MCRs) using different electron-deficient alkyne moieties. |
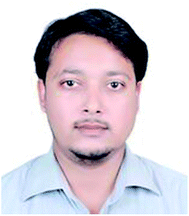 Habibur Rahman | Dr. Habibur Rahman received his Ph.D. degree in Chemistry in 2006 from AMU, Aligarh, India. Afterward, he joined a material testing laboratory in the Kingdom of Saudi Arabia. In 2009, he moved to join academics in India and abroad and taught different courses in chemistry. At present, he is working as an Assistant Professor in Chemistry in the Department of General Studies, Jubail Industrial College, Saudi Arabia. He has expertise in pharmaceuticals and its characterization using modern and sophisticated instrumentations. |
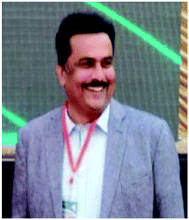 Shafiullah | Dr. Shafiullah was born in 1966 and grew up in the district of West Champaran in Bihar, India. He received his M.Sc. degree from Aligarh Muslim University (AMU), India in 1988 with Organic Chemistry specialization. After completing his M.Sc., he successfully completed his Ph.D. degree under the supervision of Professor M. Ilyas in 1994 from AMU. Presently, he is working as an Assistant Professor in Department of Chemistry, AMU. His research interests are mainly centred on the isolation and characterisation of natural products from different medicinal plants and the biological evaluation of the medicinal plant extract. |
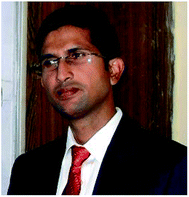 Md. Musawwer Khan | Dr. Md. Musawwer Khan was born and grew up in the district of Deoria, Uttar Pradesh in India. He obtained his B.Sc. (Chemistry) and M.Sc. (Organic Chemistry) in 2001 and 2003, respectively, from Aligarh Muslim University (AMU), India. He also completed a B.Ed. in 2004 from AMU, Aligarh. He joined Professor Abu T. Khan's group as a Junior Research Fellow and successfully completed his Ph.D. degree in 2011 from IIT, Guwahati. Presently, he is working as an Assistant Professor in the Department of Chemistry, AMU, India. He worked as IAS-SRF postdoctoral research under Professor Y. D. Vankar at IIT Kanpur in 2012. His research interests are mainly centred on synthetic methodologies, heterocyclic chemistry, green chemistry, diversity-oriented synthesis and the synthesis of biologically active molecules. |
1. Introduction
The novel inventions of small drug-like heterocycles are a challenging task for an organic and medicinal researcher. Among all known organic compounds, heterocycles occupy a central place because of their enormous presence in natural products, hormones, vitamins, antibiotics, dyes, agrochemicals, pharmaceuticals, etc.;1,2 moreover, due to their well-established therapeutic properties, heterocycles are significantly needed for counteracting various infectious diseases to achieve a healthy human life. They show a broad spectrum of biological activities such as antitumor,3 antibiotic,4 antidepressant,5 antimalarial,6 anti-HIV,7 antimicrobial,8 antiviral,9 anticancer,10 antitubercular,11 antidiabetic12 and anti-inflammatory activities.13 Some examples of pharmacologically and biologically potent heterocycles are depicted in Fig. 1.14–16 In addition, the molecules having a heterocycle as a subunit exhibit enhanced solubility and salt formation; this enables their oral absorption and bioavailability;17 therefore, both the development of a novel methodology and the strategic replacement of known methodology for the preparation of these heterocycles remain the active areas of research. The development of an innovative, environmentally benign, sustainable and practical method to carry out organic reactions demands certain regulations and guidelines.18 The common tactics to improve the synthetic methods for the synthesis of heterocycles are either to design a distinctive precursor or to develop novel catalysts.19 However, organic building blocks are important in a number of chemical protocols and play a key role in the reaction medium for chemical transformation to create molecular diversity.
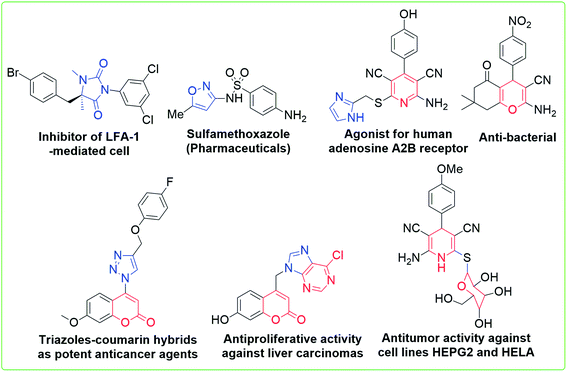 |
| Fig. 1 Some biologically active heterocycle-containing compounds. | |
In the present scenario, different kinds of ketene N,S-acetals, which contain electron-releasing and electron-withdrawing substituents, lead to unique structural features and are of significant interest to synthetic chemists due to their utility as useful starting materials in organic chemistry.20,21
In addition, ketene N,S-acetal derivatives are as such used as drugs for the treatment of hypertension diseases and usually employed as probes for nucleic acids to investigate the interaction between G4 (G-quadruplex) and its ligands (Fig. 2).22
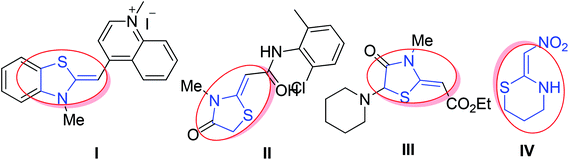 |
| Fig. 2 Examples of drugs, insecticides and probes having the ketene N,S-acetal structure. | |
Interestingly, the cyclic nitroketene N,S-acetal nithiazine IV was the first reported compound of neonicotinoid insecticides23 and is currently used as a common insecticide all over the world. Recently, Rao and his co-workers have described the in silico evaluation of acyclic nitroketene N,S-acetals, i.e., (E)-N-methyl-1-(methylthio)-2-nitroethenamine (NMSM) derivatives, against Alzheimer's disease.24
Presently, five types of ketene acetals are mainly known: S,S-acetals (V), O,O-acetals (VI), N,S-acetals (VII), N,N-acetals (VIII) and silyl acetals (IX), as depicted in Fig. 3. Among these, ketene N,S-acetals are the most abundant family in the world of ketene acetal chemistry. Furthermore, the ketene O,O; N,N; S,S; and N,S and ketene silyl acetals have been well studied and reviewed.25,26 However, according to our survey, to date, a review article summarizing the preparation and synthetic utilities of nitroketene N,S-acetals has not been reported in the literature.
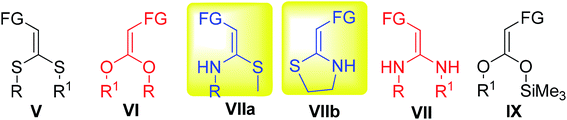 |
| Fig. 3 The structures of different kinds of ketene acetals. | |
In the present review, we tried to cover the chemistry of nitroketene N,S-acetals in terms of their interesting structures, reactivity patterns, general and special methods of preparation and applications in the synthesis of heterocycles; moreover, we focused on the actions and reactions related to their exceptional behaviour and the unique arrangement of their reactive sites towards attack by nucleophiles and electrophiles. These features of nitroketene N,S-acetals make them highly flexible and easy to use in the Michael addition, cyclization, annulation and multicomponent reactions. Nowadays, multicomponent reactions (MCRs) have become a prominent strategy and are chosen over stepwise synthesis due to the following reasons: reduced waste, minimal usage of toxic and harmful chemicals, short synthetic time period, simple workup of compounds, high yields, operational simplicity and economic viability;27 therefore, MCRs are a powerful tactic to attain the aim of green and sustainable chemistry by reducing the formation of vast quantities of waste for the promotion of green chemistry.
2. Nitroketene N,S-acetal chemistry
2.1 Characteristic structure and reactive sites
The acyclic simple nitroketene N,S-acetals, such as (E)-N-methyl-1-(methylthio)-2-nitroethenamine (NMSM) and its derivatives, are flexible molecules. On the other hand, the cyclic nitroketene N,S-acetals have a rigid structure and behave as a Michael donor-type 1,3-N,C di-nucleophilic precursor for the construction of N-heterocyclic compounds. They contain three specific functional groups, i.e. nitro, methylamine and sulfanyl, on the ethylene skeleton, and each of these functional groups bonds well and play a significant role in the reaction media for the formation of products; the acyclic nitroketene N,S-acetals possess a distinguished feature: the methylsulfanyl group acts as a good leaving group and also possesses electron releasing capacity. Moreover, this methylsulfanyl group can be substituted with a different kind of nucleophile following the substitution nucleophilic vinyl (SNV) mechanism. The ethylene motif has a polarized push–pull type of alkene, and due to this, the one end develops an electrophilic character, whereas the other end develops a nucleophilic character with electron movement stemming from the methylsulfanyl-/methyl amino group towards the nitro group. The interesting structural features of these two types of nitroketene N,S-acetals are shown in Fig. 4.
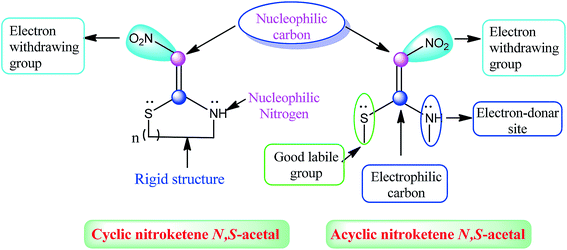 |
| Fig. 4 Characteristic structural features of cyclic and acyclic nitroketene N,S-acetals. | |
These features of NMSMs make them a multi-faceted precursor that can be used to explore the creation of more diverse and interesting complex molecules.
2.2 General methods for the preparation of acyclic and cyclic nitroketene N,S-acetals
This section covers the general methods used for the preparation of cyclic/acyclic ketene N,S-acetals possessing a –NO2 group at the acceptor end. In 1967, Gompper and Schaefer synthesized the nitroketene N,S-acetal derivatives 4 via the one-pot reaction of phenyl isothiocyanate 1 with nitromethane 2 followed by S-methylation by MeI using sodium hydride (NaH) as a base in DMF to afford good yields.
This synthetic method provides efficient access to 4 on a gram scale under mild conditions from simple starting materials (Scheme 1).28 In 1988, Young and his co-workers developed a new approach for the synthesis of the unsymmetrical nitroketene N,S-acetal 7 via the amination of monosulfoxide 5 of l,l-bis(methylthio)-2-nitroethene (Scheme 2).29
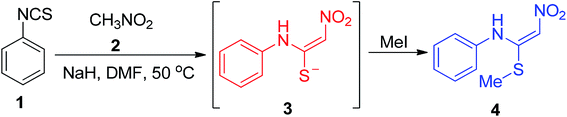 |
| Scheme 1 One-pot synthesis of nitroketene N,S-acetals. | |
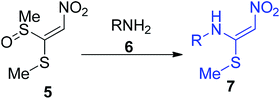 |
| Scheme 2 Synthesis of nitroketene N,S-acetals by the amination of 5. | |
On the other hand, a new tactic was adopted for the synthesis of the compounds 9 and 11 by Deshmukh et al., where nitromethane 2 was allowed to react with dimethyl methylcarbonimidodithioate 8 and 10 under catalysis by a rare-earth (La, Pr and Sm)-exchanged NaY zeolite (Scheme 3).30,31
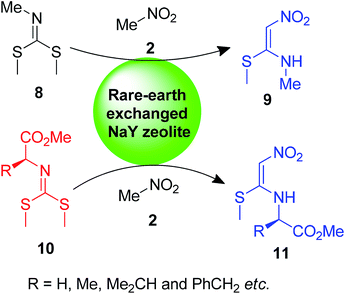 |
| Scheme 3 New approach for the synthesis of the compounds 9/11 using zeolite. | |
Maybhate and his group treated N-sulfonyl carbonimidodithioates 12 with the nitroethane derivatives 13 in the presence of anhydrous K2CO3 in DMSO to obtain 1-methylthio-l-sulfonamido-2-nitroethenes 14 (Scheme 4).32 Then, the same group utilized 15 for the synthesis of compound 17 in the presence of different primary and secondary amines 16 at 30 °C (Scheme 5).33
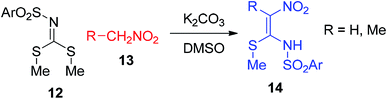 |
| Scheme 4 New kinds of nitroketene N,S-acetal derivatives 14. | |
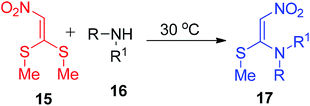 |
| Scheme 5 Synthesis of the nitroketene N,S-acetal derivatives from 15. | |
Contreras and his co-workers synthesized a new analogue of nitroketene N,S-acetals 19 by the reaction between 15 (l,l-bis(methylthio)-2-nitroethene) and aminoethyl morpholine 18 (Scheme 6).34
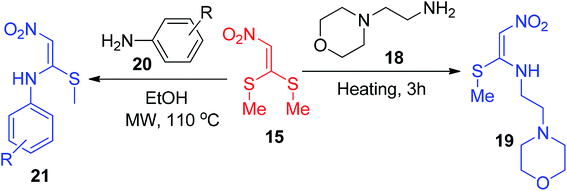 |
| Scheme 6 Synthesis of the nitroketene N,S-acetals 19/21 from l,l-bis (methylthio)-2-nitroethene. | |
The same starting component 15 was explored by Sangi and Correa to generate a short library of nitroketene N,S-acetals 21 via the reaction of 15 with one equivalent of a number of primary amines 20 under microwave irradiation with high yields (Scheme 6).35 Again, similar conditions were utilized for the synthesis of the cyclic and acyclic nitroketene N,S-acetals 23 and 25 by the same group (Scheme 7).36
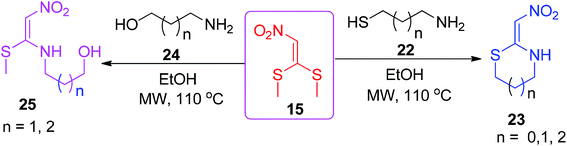 |
| Scheme 7 Synthesis of new cyclic/acyclic nitroketene N,S-acetal derivatives. | |
Dunkern et al. described a slightly modified protocol for the synthesis of the nitroketene N,S-acetal derivatives 28 from 15, as depicted in Scheme 8.37 The precursor 15 was prepared by mixing 26 with MeI in DMF at room temperature.
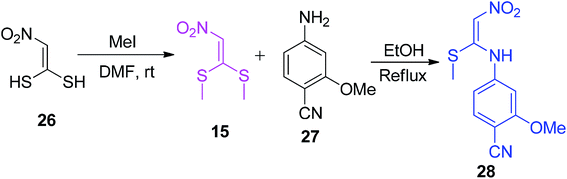 |
| Scheme 8 Synthesis of the nitroketene N,S-acetal derivatives 26. | |
The five-membered cyclic nitroketene N,S-acetal 33 was prepared by Shiokawa et al. via a three-step process involving the S-methylation of 29, followed by condensation with the ethyl nitroacetate 31 to form 32, which generated 33 upon hydrolysis and decarboxylation (Scheme 9).38
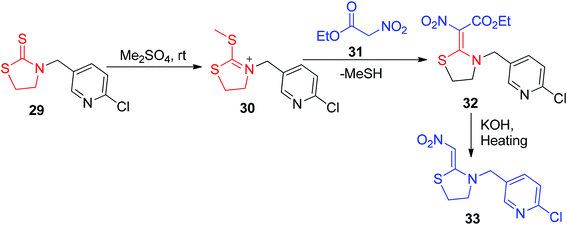 |
| Scheme 9 Synthesis of cyclic nitroketene N,S-acetal derivatives. | |
Huang and Shi described a two-step method for the formation of the five-membered simple nitroketene N,S-acetals 36. In the first step, nitromethane 2 and carbon disulfide 34 were combined in the presence of sodium hydride followed by S-methylation using MeI to form the compound 15. In the next step, 36 was generated by the reaction of 2-aminoethanethiol 35 with 15 under ethanol reflux conditions (Scheme 10).39
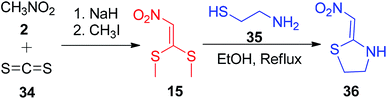 |
| Scheme 10 Synthesis of five-membered cyclic nitroketene N,S-acetals. | |
3. Applications of nitroketene N,S-acetal in the synthesis of heterocycles
Heterocycles are compounds that contain a cyclic ring along with one or more heteroatoms such as nitrogen, sulfur, oxygen, phosphorus, etc. Moreover, acyclic and cyclic nitroketene N,S-acetals have different structural firmness. Thus, depending upon the type of nitroketene-N,S-acetal used, the applications can be classified into two major sections.
3.1 Applications of acyclic nitroketene N,S-acetals
The structural flexibility of acyclic nitroketene-N,S-acetals allow them to be utilized in the construction of various oxygen, nitrogen and sulfur atom-containing cyclic compounds.
3.1.1 Synthesis of oxygen-containing heterocycles. Recently, our group demonstrated the synthesis of a short combinatorial library of 4H-chromen-5-ones 39 and their bis derivatives 41 using catalyst- and solvent-free conditions via a one-pot MCR at 110 °C. The reaction occurred between cyclic β-dicarbonyl 37, aryl aldehydes 38 and NMSM 9 in a short time with high yields. This convenient and green method has several advantages such as the avoidance of expensive catalysts, hazardous solvents and chromatographic purification (Scheme 11).40
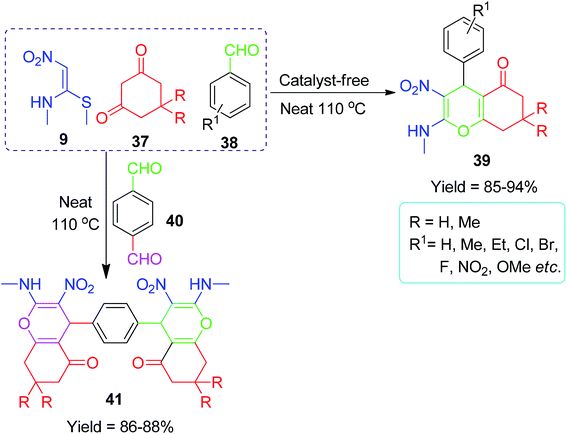 |
| Scheme 11 Synthesis of the 4H-chromen-6-ones 39 and bis-4H-chromen-6-ones 41. | |
Furthermore, Shinde and his co-workers used NMSM for the construction of biologically active 4H-chromenone compounds via a three-component reaction using the 6,6′-thiobis(methylene)-β-cyclodextrin dimer 42 as a reusable promoter in aqueous media.41 They have also described the synthetic procedures of dimeric β-cyclodextrin linked by a thio-methylene bridge as a supramolecular host. The reaction mechanism was described through molecular complexation and proposed based on the 2D ROESY NMR spectroscopic techniques (Scheme 12). Similarly, Perumal et al. developed a convenient method for the regioselective synthesis of chromenones 39 by the one-pot three-component condensation of various aromatic aldehydes 38, dimedone 37 and NMSM 9 in the presence of 20 mol% piperidine in ethanol.42
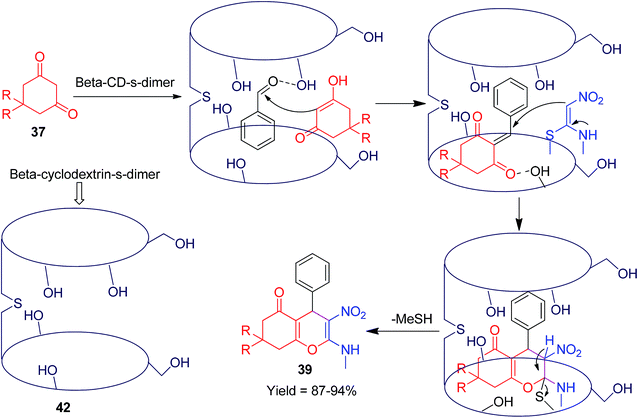 |
| Scheme 12 The proposed reaction mechanism occurring via supramolecular host complexation. | |
Next, Jadhav and his co-workers reported a new approach for the synthesis of coumarin-fused oxygen-containing heterocycles, pyrano[3,2-c]chromen-5-ones 44, using NMSM. The reaction was carried out under solvent-free conditions using 4-hydroxycoumarin 43, aryl aldehydes 38 and NMSM 9, and silica-supported tungstic acid (STA) was employed in a catalytic amount; they showed recyclability of the catalyst up to the 4th cycle and achieved an 89% yield (Scheme 13).43 Similarly, the β-CD-S dimer and piperidine have also been used as catalysts for the synthesis of 44.41,42 Moreover, Mao et al. developed a sustainable method for the synthesis of the new type of coumarin-fused pyrano[4,3-b]pyran-5-one derivatives 46 using 9 as a building block. The compounds were synthesized by irradiating all the starting components (9, 45 and 38) in a microwave oven in the presence of ammonium acetate in EtOH (Scheme 14).44
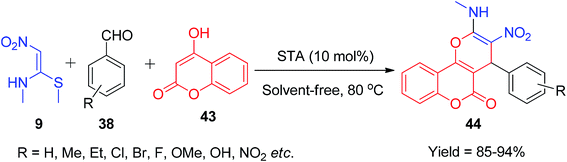 |
| Scheme 13 Synthesis of functionalized pyranochromen-5-one derivatives. | |
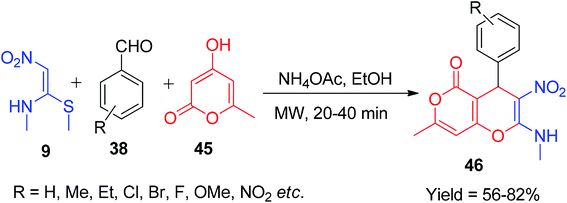 |
| Scheme 14 Synthesis of functionalized coumarin-fused pyran derivatives. | |
After this, Gunasekaran et al. applied NMSM for the construction of quinoline-fused pyran moieties. The pyranoquinolinone framework is a privileged sub-structure widely spread in several natural products.45,46 This fascinating compound was synthesised via the ZnCl2-catalyzed three-component reactions of 4-hydroxy-1-methylquinolin-2(1H)-one 47, NMSM 9, and the aromatic aldehydes 38 in ethanol.
This method afforded the pyrano[3,2-c]quinolin-5(6H)-ones 48 in good yields (85–93%) (Scheme 15).47 Next, the NMSM 9 was also chosen as a starting material by Reddy et al. for the synthesis of benzo[f]chromen-3-amine derivatives under mild aqueous conditions. Via this protocol, the compound 50 was afforded by a one-pot cascade reaction between various aromatic aldehydes 38, naphthalen-2-ol 49 and 9 under catalyst-free conditions using a greener medium (ethanol-water mixture) (Scheme 16).48
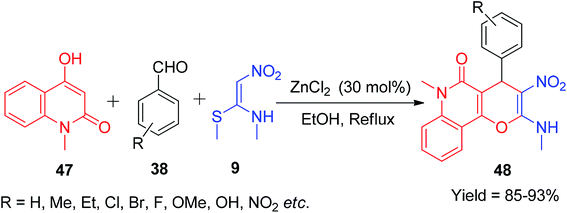 |
| Scheme 15 Synthesis of quinoline-fused pyran derivatives 48. | |
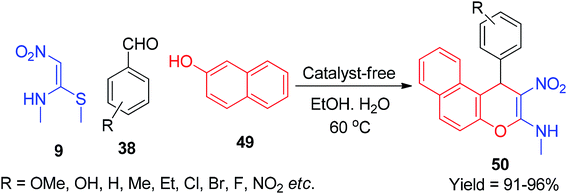 |
| Scheme 16 Synthesis of functionalized benzochromen derivatives. | |
In addition, NMSM was explored by Sivakumar and his co-workers for the synthesis of simple 4H-pyrans. They synthesized a series of novel 2-(1H-indol-3-yl)-6-(methylamino)-5-nitro-4-aryl-4H-pyran-3-carbonitriles 53 and 6-(methylamino)-4-(aryl)-5-nitro-2-phenyl-4H-pyran-3-carbonitriles 54 in the presence of Et3N as a catalyst via the three-component domino reactions of 51 and 52, aryl aldehydes 38 and NMSM 9, respectively (Scheme 17).49
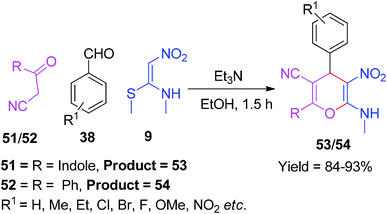 |
| Scheme 17 Synthesis of indole-containing 4H-pyran derivatives. | |
Kamalraja and Perumal demonstrated an environmentally friendly and sustainable method for the synthesis of the indole-containing 4H-pyrans 56 using NMSM 9 as one of the building blocks. The indole ring system is a well-known heterocycle present in nature and an important structural motif in many pharmaceutically active compounds.50 The reaction was conducted in the presence of InCl3 as a catalyst via the microwave irradiation of the aromatic aldehydes 38 and 3-cyanoacetylindoles 55 with NMSM 9 under solvent-free conditions. Furthermore, they described its chemical transformation to indolyltriazolylpyran 59 hybrids, as shown in Scheme 18.51
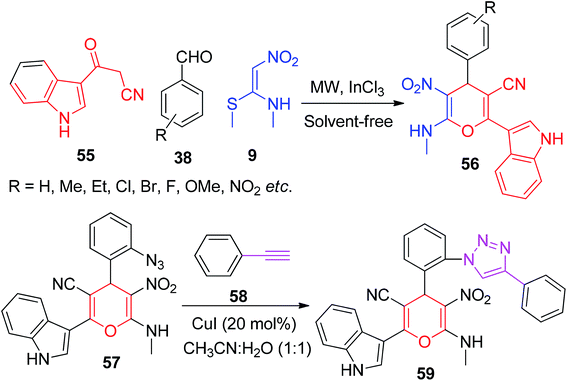 |
| Scheme 18 Synthesis of indole-containing 4H-pyran derivatives and their functionalization. | |
In 2018, Wang and co-workers synthesized the penta-substituted 4H-pyran motifs 61 using NMSM. This multicomponent domino reaction was performed using the aromatic aldehydes 38, pivaloylacetonitrile 60 and NMSM 9 in the presence of NH4OAc under microwave irradiation in ethanol. The synthesized compounds exhibited good fluorescence intensity with the excitation wavelength of 300 nm (Scheme 19).52
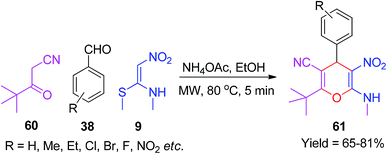 |
| Scheme 19 Synthesis of penta-substituted 4H-pyran derivatives 61. | |
Kanchithalaivan and his group developed an effective one-pot four-component protocol for the synthesis of novel 1,4-dihydropyrano[2,3-c]pyrazol-6-amines 64/66 and benzochromenes 68 using NMSM. They described a simple synthesis of novel 1,4-dihydropyrano[2,3-c]pyrazol-6-amines via the domino condensation reactions of ethyl acetoacetate/ethyl benzoylacetate 65 and diethyl acetylenedicarboxylate 62 with hydrazines 63, aryl aldehydes 38, and NMSM 9 in the presence of diisopropylethylamine (DIPEA) (Scheme 20).53
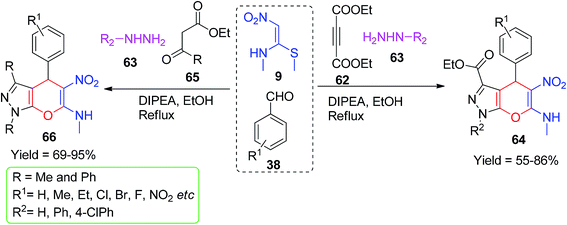 |
| Scheme 20 Synthesis of the functionalized pyrazole-fused 4H-pyrans 64/66. | |
The scope of the abovementioned protocol was also envisaged in the cyclic system (2-hydroxy-1,4-naphthoquinone) 67 with aromatic aldehydes and NMSM using ethanol under reflux conditions. This reaction afforded the corresponding products even in the absence of a base. The benzo[g]chromene-5,10-diones 68 were achieved in excellent yields, as represented in Scheme 21. In 2018, Krishnammagari and his group reported a similar reaction under solvent-free conditions using poly(oligo ethylene glycol methacrylate)-g-supported coated double hydroxides (LDHs-g-POEGMA) as a reusable heterogeneous catalyst.54
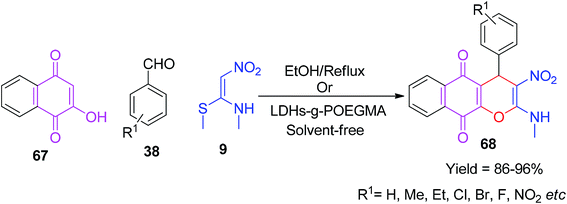 |
| Scheme 21 Synthesis of the functionalized benzo[g]chromene-5,10-diones 68. | |
After this, Poomathi et al. described a new class of spiro 1,4-dihydropyridine derivatives using NMSM as a building block. They developed a high-yielding InCl3-mediated regioselective method for the synthesis of spiro-pyrans 71a via a domino, one-pot reaction of isatins 69, pyrazolones 70 and NMSM 9; on the other hand, Survase et al. also used a similar combination of pyrazolones 70 and NMSM 9 with aromatic aldehydes 38 in the presence of the abovementioned catalyst in aqueous ethanol for the synthesis of pyrazole-fused 4H-pyrans 71b (Scheme 22).55,56
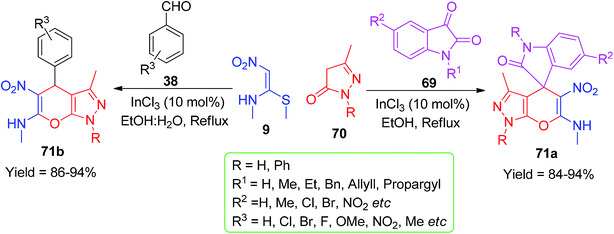 |
| Scheme 22 Synthesis of functionalized pyrazole-fused spiro pyran derivatives. | |
As a continuation of the exploration of NMSM derivatives to develop new type of 4H-chromenes 73/75, Rao et al. described a two-component approach based on Michael-type addition followed by O-cyclization. The synthesis was achieved via a base-catalyzed reaction of 7 with 2-hydroxybenzaldehydes 72, and 2-alkylamino-3-nitro-4-alkylsulfanyl 4H-chromenes 73 were afforded in excellent yields. The subsequent displacement of the –SMe group of 73 with different thiol nucleophiles 74 yielded the 4H-chromenes 75, having more structural diversity in the molecules (Scheme 23).57
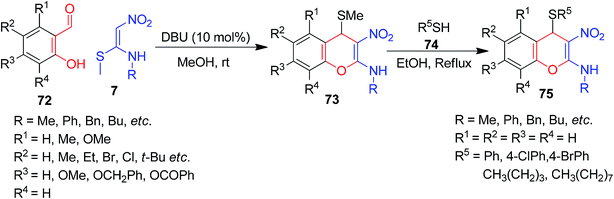 |
| Scheme 23 Synthesis of functionalized 4H-chromenes from nitroketene N,S-acetals. | |
Furthermore, the reaction was carried out between 7 and the simple 2-hydroxybenzaldehyde 72a in the presence of NaH in THF, where they isolated the final products containing 73a (1
:
1 adduct) in major amount and 4H-chromenes 76 (1
:
2 adduct) in minor amount, as depicted in Scheme 24. Next, the same research group extended the utility of 73a to the construction of various chromene- and coumarine-based derivatives (77–81) under suitable reaction conditions in a two-step sequence (Scheme 25).58
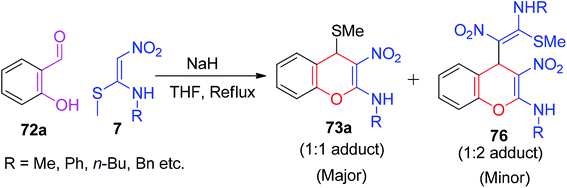 |
| Scheme 24 Synthesis of the major 1 : 1 adduct 4H-chromenes from nitroketene N,S-acetals. | |
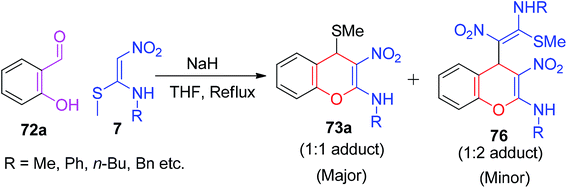 |
| Scheme 25 Synthesis of methylsulfanyl 4H-chromenes and their transformation. | |
After this, 2-hydroxybenzaldehydes were replaced by 2-((E)-2-nitrovinyl)phenol 82 for the construction of new kinds of compounds. A number of N-methyl-3-nitro-4-(nitromethyl)-4H-chromen-2-amines 83 were synthesized by the reaction of 2-((E)-2-nitrovinyl)phenols 82 and NMSM 7 derivatives in the presence of 10 mol% DBU in MeOH (Scheme 26).59 The synthesized compounds were tested for anticancer activity, which displayed moderate to good activity against two cancer cell lines, namely HeLa (cervical cancer) and HEp-2 (epidermoid laryngeal carcinoma).
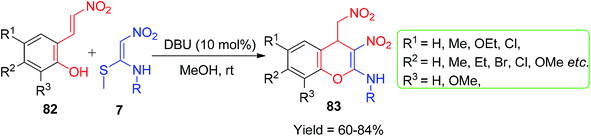 |
| Scheme 26 Synthesis of the functionalized 4H-chromene-2 amines 83. | |
Next, Jayabal and Paramasivan explored the combination of 2-hydroxybenzaldehydes 84 and NMSM 9 for the synthesis of pyrazole-containing chromene 85a rings under SF conditions.60 The one-pot four-component reaction was carried out by heating the mixture of all the starting components at 120 °C in the presence of 25 mol% piperidine for 1 h. They also used the aromatic aldehydes 38 instead of 2-hydroxybenzaldehydes under similar conditions, and the pyrazole-fused chromenes 85b were obtained (Scheme 27).
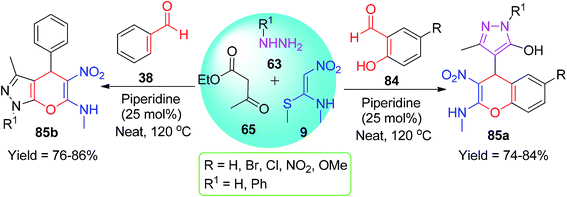 |
| Scheme 27 Synthesis of pyrazole-containing chromene from nitroketene N,S-acetals. | |
3.1.2 Synthesis of nitrogen-containing heterocycles. The research interest in nitrogen-containing heterocyclic compounds can be attributed to their profound medicinal properties. These skeletons are well-known subunits in different alkaloids, pharmaceuticals and agricultural chemicals.61
3.1.2.1 Six-membered N-heterocycles. In 2014, Rao and Parthiban developed a protocol for the synthesis of diverse, functionalized N-methyl-substituted 1,4-dihydropyridines (1,4-DHPs) 86. The pseudo three-component reaction was carried out by the reaction of NMSM 9 (2 mmol) and aromatic aldehydes 38 (1 mmol) using 2-aminopyridine (10 mol%) as a catalyst in ethanol (Scheme 28).62 The product isolated by simple filtration was further subjected to the nucleophilic substitution of the –SMe group by different kinds of primary and secondary amines; this afforded the structurally diverse 1,4-dihydropyridines 87. They also synthesised the neonicotinoid insecticide analogue 89, as shown in Scheme 29. Very recently, our group developed an improved and greener method for the synthesis of 86 using microwave irradiation under catalyst- and solvent-free conditions within 10–15 min, and a yield of 80–94% was obtained, as depicted in Scheme 28.63
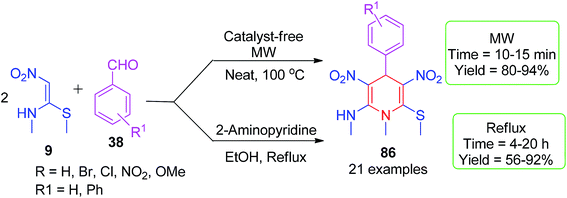 |
| Scheme 28 Synthesis of the highly substituted 1,4-dihydropyridines 86. | |
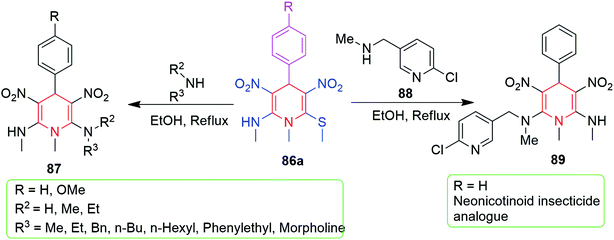 |
| Scheme 29 Functionalized 1,4-dihydropyridines 88/89. | |
Next, Hajiyeva and his co-workers described the synthesis of a new type of N-heterocyclic compounds containing the NMSM scaffold using the Knoevenagel condensation product of malononitrile and aromatic aldehydes. A short library of poly-functionalized 2-amino-1-methyl-6-(methylthio)-5-nitro-4-aryl-1,4-dihydropyridine-3-carbonitriles 91 was synthesised through the multicomponent condensation of aryl aldehydes 38, malononitrile 90, and 9 in the presence of Et3N in acetonitrile, as depicted in Scheme 30.64
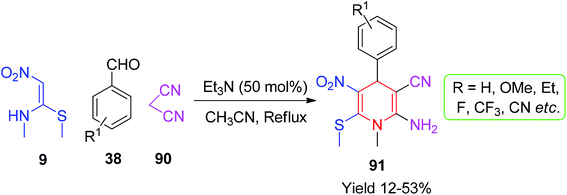 |
| Scheme 30 Synthesis of the substituted 2-amino-1,4-DHPs. | |
In 2014, Gunasekaran and his co-worker used NMSM for the formation of a pyrazolo[3,4-b]pyridine 92 ring system. The synthesis of the compounds 93 was achieved by the reaction of NMSM 9, aryl aldehydes 36 and 3-methyl-1-phenyl-1H-pyrazol-5-amine 92 in the presence of the bio-catalyst L-proline in refluxing ethanol (Scheme 31).65
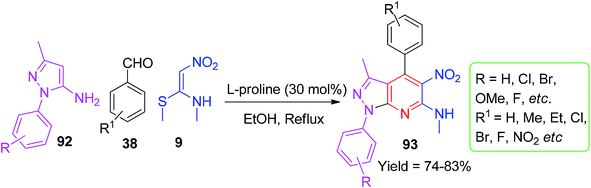 |
| Scheme 31 Synthesis of functionalized pyrazole-fused pyridines. | |
Next, Poomathi et al. synthesized the six-membered nitrogen-containing heterocycles 2-pyridinones 95 using NMSM and chromone derivatives possessing an aldehydic group at an appropriate position.
This well-organized and flexible route provides the functionalized compounds 95 in the presence of indium triflate by the reaction of 3-formylchromones 94 and NMSM 9 in refluxing ethanol. The simple workup, usage of inexpensive and easily available starting materials, high yields, etc. are some of the advantages associated with this method (Scheme 32).66 They have also established and discussed the mechanistic aspects for the formation of products, which are shown in Scheme 33.
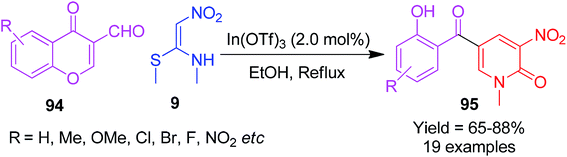 |
| Scheme 32 Synthesis of functionalized 2-pyridinone derivatives from NMSM. | |
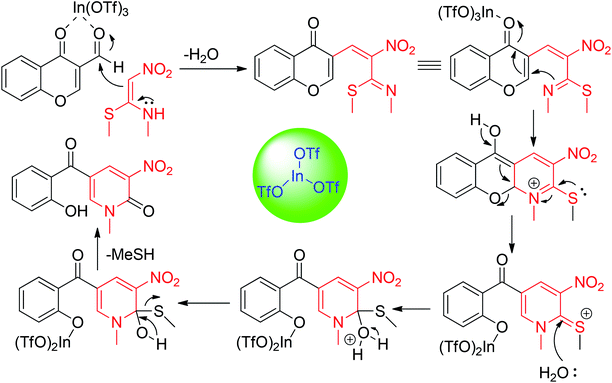 |
| Scheme 33 Synthesis of functionalized 2-pyridinone derivatives from NMSM. | |
Next, the use of a heterocyclic amine and NMSM by Jadhav and his co-workers led to the development of a new protocol for the construction of a novel benzoimidazopyrimidine ring system 97. In this method, 2-aminobenzimidazole 95, aldehydes and NMSM 9 were allowed to react in the presence of the p-TSA catalyst in ethanol under refluxing conditions (Scheme 34).67
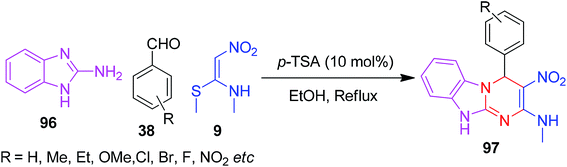 |
| Scheme 34 Synthesis of functionalized benzoimidazole-fused pyrimidines. | |
The plausible mechanism for the formation of N-methyl-3-nitro-aryl-benzo[4,5]imidazo[1,2-a]pyrimidin-2-amine 97 involved Knoevenagel condensation, Michael addition, then N-cyclization and tautomerisation, as shown in Scheme 35.
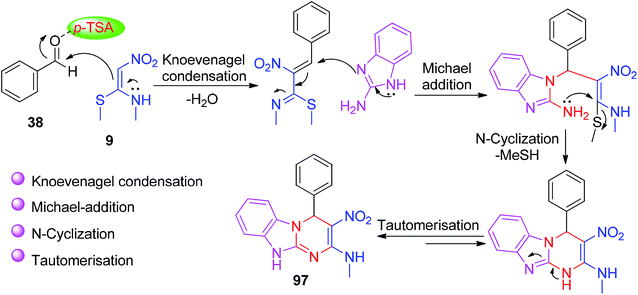 |
| Scheme 35 Plausible mechanism for the formation of benzoimidazole-fused pyrimidines. | |
The next application of NMSM in the construction of N-heterocycles was demonstrated by Chakrabarti et al. based on the substantial reactivity of itaconic anhydride. A new class of pyridinone derivatives 99 having a carboxylic acid group was synthesized by the reaction of itaconic anhydride 98 with the NMSM derivatives 7 in refluxing MeCN (Scheme 36).68
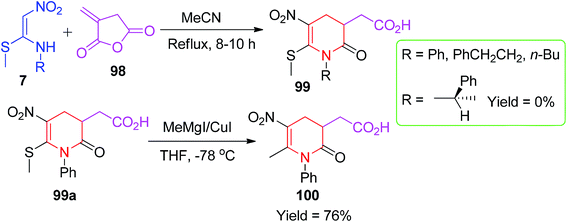 |
| Scheme 36 Synthesis of pyridinone from nitroketene N,S-acetals. | |
Further functionalization of the phenyl derivatives 99a using the MeMgI/CuI reagent afforded the compound 100 in a 76% yield. The proposed mechanism of product formation is outlined in Scheme 37.
 |
| Scheme 37 Plausible mechanistic outline for the formation of pyridinone 99. | |
For the preparation of pyrimidine-2-one derivatives containing NMSM scaffolds, Sukach and his group described the [3 + 3] annulation approach. They synthesized a series of 3,4-dihydropyridine-2-ones 102 by the reaction between 1-chlorobenzyl isocyanates 101 and 7 in DCM under reflux conditions (Scheme 38).69 The methylthio group present in the compound 102 was further substituted by primary and secondary amines.
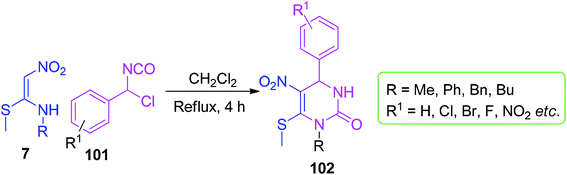 |
| Scheme 38 Synthesis of the pyrimidinones 102 from nitroketene N,S-acetals. | |
A different reaction profile of the NMSM derivative was shown when they were mixed with POCl3 to generate the quinoxaline derivatives 104.
This was carried out by Venkatesh and his co-workers through the hetero-annulation reaction of the corresponding NMSM derivative 103 in the presence of POCl3 in MeCN. They also found that the formation of 104 by the reaction of 103 with POCl3 unexpectedly produced quinoxaline instead of 3-nitroquinoline 105 (Scheme 39).70,71
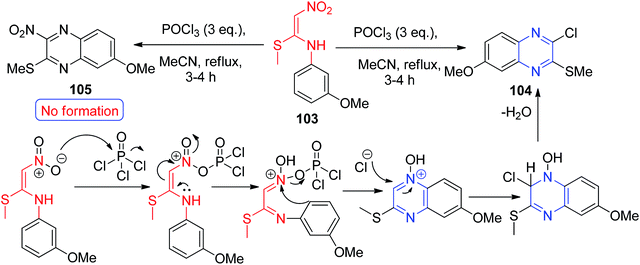 |
| Scheme 39 Synthesis of functionalized quinoxalines from nitroketene N,S-acetals. | |
Using the same tactic, Nakamura and his group prepared 104 in a 54% yield. In the presence of m-CPBA, 104 was further converted to 106, which was tested against Trypanosoma cruzi Y strain (the cause of Chagas disease). The outcome reveals that the compound 106 is active against the abovementioned strain (Scheme 40).72
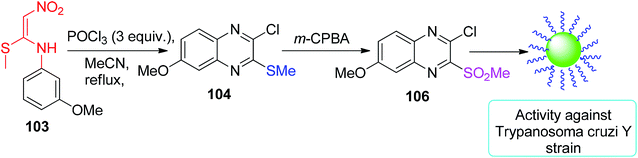 |
| Scheme 40 Synthesis of the functionalized quinoxalines 106. | |
3.1.2.2 Five-membered N-heterocycles. Among the five-membered N-heterocycles, pyrroles are considered as a privileged scaffold as they are found in natural products such as heme, chlorophyll, and vitamin B12.73,74 These are the most likely five-membered rings that can be constructed using NMSM. Therefore, this section has been devoted to the synthetic application of NMSM in the formation of five-membered nitrogen-containing heterocyclic systems.Very recently, Chaudhary et al. described GAP-assisted chemistry for the synthesis of novel pyrroles using NMSM.
The three-component catalyst-free reaction of arylglyoxal monohydrate 108, 9 and the barbituric acid derivatives 107 in one pot was performed in aqueous media. The methanethiol functionality present in the synthesized pyrroles 109 makes them easily accessible to further functionalization, thereby leading to a new type of heterocycles (Scheme 41).75 The substrate scope, time and yields are shown in Scheme 42.
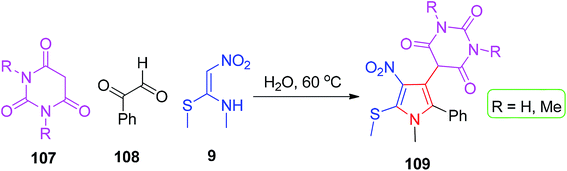 |
| Scheme 41 Synthesis of the functionalized pyrrole derivative 109. | |
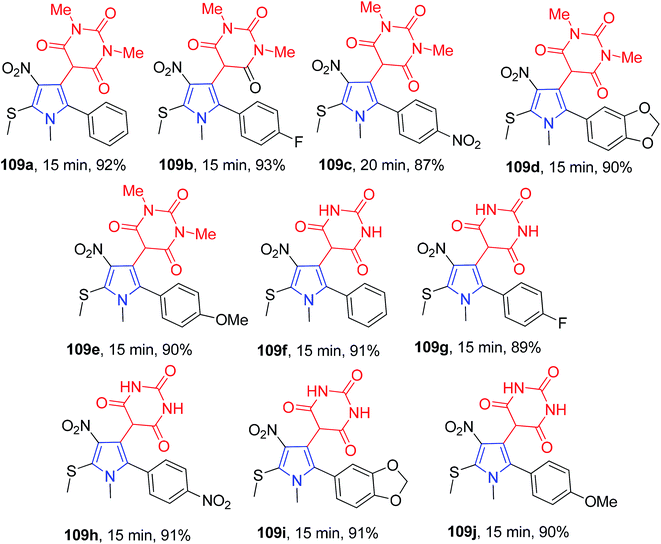 |
| Scheme 42 The substrate scope of the pyrrole derivatives 109. | |
In 2018, Rahimi and co-workers synthesised a new class of polysubstituted heterocyclic ring systems 112 using NMSM 9 with indanetrione 110 and aryl amines 111 in EtOH at ambient temperature via a one-pot MCR (Scheme 43).76
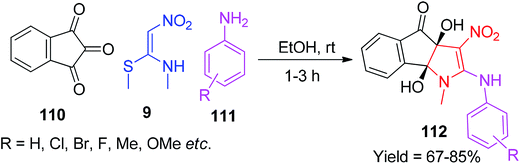 |
| Scheme 43 Synthesis of the functionalized pyrrole derivatives 112. | |
Similarly, by performing the reaction of ninhydrin 113 and 7 in the presence of ethanol at room temperature, Jeyachandran et al. synthesized the novel dihydroindeno[1,2-b]pyrrol-4(3aH)-ones 114 (Scheme 44).77
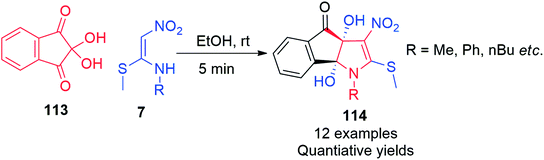 |
| Scheme 44 Synthesis of the functionalized pyrroles 114. | |
In 2015, Balachandra and co-workers reported a protocol for the synthesis of a new class of substituted pyrroles 116. The reaction of 7 and β-nitrostyrenes 115 under SF and metal-free conditions at 55 °C followed the iodine-catalyzed [3 + 2] annulation route.
Again, the pyrrole derivatives 116 were synthesised via an MCR strategy by the reaction of NMSM 9 and aromatic aldehydes 38 with nitromethane 2 in the presence of the same amount of molecular iodine at 90 °C (Scheme 45).78 The synthesized pyrrole derivatives were tested for in vitro antibacterial activity against Gram-negative and Gram-positive infectious pathogens, and most of the compounds displayed good inhibitory properties.
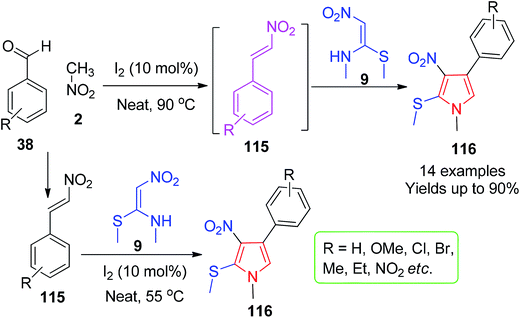 |
| Scheme 45 Synthesis of the functionalized pyrroles 115 from NMSM. | |
Very recently, Balachandra and his co-worker established two different protocols for the synthesis of two different pyrroles 117/119. They synthesized 2-(2-argio-1-methyl-5-(methylthio)-4-nitro-1H-pyrrol-3-yl)-2-cyanoacetamide 117 by the reaction of NMSM 9, arylglyoxals 108 and malononitrile 90 using the H2O/PEG-400 solvent combination.
They also synthesized the pyrrole derivatives 119 under neat conditions using methyl cyanoacetate 118 in place of the malononitrile 90 via the decarboxylative elimination reaction (Scheme 46).79 Cytotoxicity studies of some of the selected newly synthesized compounds were also conducted on the L929 cells, and the test compounds exhibited good cell viability at lower concentrations.
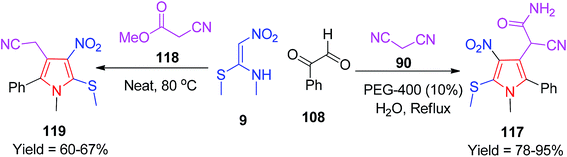 |
| Scheme 46 Synthesis of functionalized pyrroles from NMSM. | |
Misra and his group described an interesting route for the synthesis of the substituted pyrroles 122 by the 1,3-dipolar cycloaddition of the readily available NMSM derivative 120 with the active methylene ethyl isocyanoacetate 121 to provide the desired pyrroles (Scheme 47).80
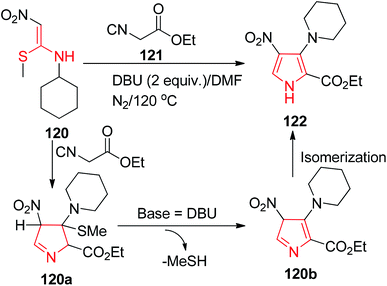 |
| Scheme 47 Synthesis of the functionalized pyrroles 122. | |
3.1.3 Synthesis of sulfur-containing heterocycles. In literature, to date, only one method has been reported for the synthesis of S-heterocycles using NMSM derivatives. In 2015, Kumar and his co-workers developed a protocol for the synthesis of the 2-amino-3-nitrothiophen derivatives 124 by the reaction of 7 with the 1,4-dithiane-2,5-diol 123 using K2CO3 as a base under refluxing ethanol (Scheme 48).81 A plausible mechanism leading to the construction of 124 is outlined in Scheme 49.
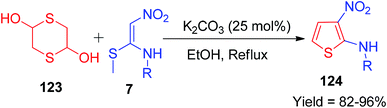 |
| Scheme 48 Synthesis of the functionalized thiophene 124. | |
 |
| Scheme 49 The proposed mechanism for the formation of the 3-nitrothiophen-2-amines 124. | |
3.2 Applications of cyclic nitroketene N,S-acetals
Owing to structural rigidity in cyclic nitroketene N,S-acetals, they behave as 1,3-N,C-dinucleophiles and generate only nitrogen-containing heterocyclic compounds in their reactions. The commercially available six-membered nithiazine and five-membered cyclic nitroketene N,S-acetals have been significantly explored in the literature and act as Michael donors; therefore, their reaction with a Michael acceptor in the reaction medium is most anticipated. In this context, Altug and his group utilized 2-nitromethylenethiazolidine 36 for the formation of the functionalised fused N-substituted 1,4-DHPs 125.
The Et3N-catalyzed one-pot reaction was executed using active methylene compounds (i.e., malononitrile 90), ethyl cyanoacetate 126 and the aryl aldehydes 38 with 36 under mild conditions (Scheme 50).82 When the ethyl cyanoacetate 126 was used, only 1,4-dihydropyridine 127 was formed via grinding using a mortar for 10 min under neat conditions (Scheme 51). Moreover, the ratios of the enamine and imine tautomers 129 and 130, respectively, were observed in an active methylene compound bearing a sulfoxide group, 2-phenyl sulfonylacetonitrile 128 (Scheme 52).
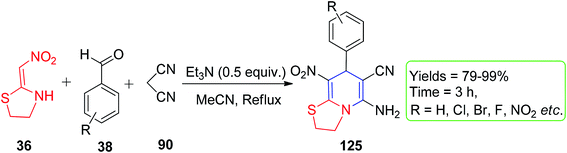 |
| Scheme 50 Synthesis of the functionalized fused dihydropyridines 125. | |
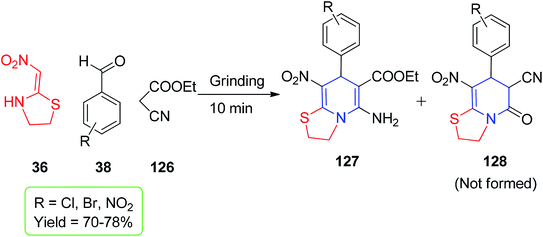 |
| Scheme 51 Synthesis of the highly substituted 1,4-DHPs 127. | |
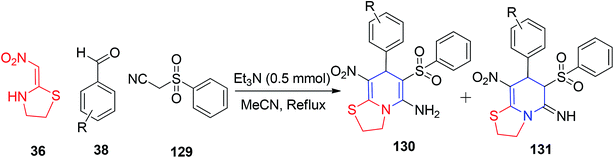 |
| Scheme 52 Synthesis of the functionalized enamine imine tautomers 130 and 131. | |
All the synthesized products were tested for in vitro anticancer activity using the MTT assay towards four human cancer cell lines, i.e. PC3 prostate, LoVo colorectal, A549 lung and MCF-7 breast cancer cell lines, and the compounds showed moderate to good activity.
Similarly, the preparation of the dihydropyridine motif was explored using nithiazine by Tian et al. The six-membered fused N-substituted 1,4-DHPs 134a-j were obtained by mixing the active methylene compounds 133 and the aromatic aldehydes 38 with 132 in the presence of piperidine (10 mol%) under mild conditions (Scheme 53).83 Furthermore, the replacement of malononitrile with the malononitrile dimer 136 was conducted by Sun and his co-workers for the synthesis of pyridine-fused 1,4-DHPs ring system.
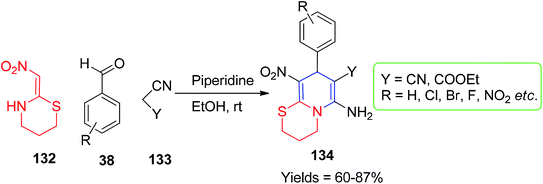 |
| Scheme 53 Synthesis of functionalized six-membered fused 1,4-dihydropyridine. | |
The tricyclic pyridine-fused 1,4-DHPs 137 were obtained by the condensation of the malononitrile dimer 136 with the aromatic aldehydes 38 under the piperidine/AcOH catalytic conditions using ethanol as a solvent (Scheme 54).84
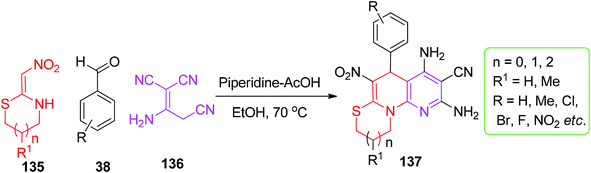 |
| Scheme 54 Synthesis of the tricyclic pyridine-fused 1,4-DHPs 137. | |
On the other hand, Bayat and his group synthesized fused 1,4-DHPs utilizing the five-membered cyclic nitroketene N,S-acetal 36, which was prepared via the one-pot reaction of 15 with cysteamine hydrochloride 138 in the presence of the Et3N catalyst. Then, the 1,4-DHP derivatives 140 were synthesized by refluxing an aqueous ethanolic solution of compound 36, dimedone 37 and different aromatic aldehydes 38 (Scheme 55).85 The reaction proceeded via the Michael addition of the intermediate 139 to afford a short library of the fused 1,4-DHPs 140 in 75–94% yields. The Michael addition approach was also adopted by Yildirim and his group for the synthesis of different kinds of pyridinones (Scheme 56).86
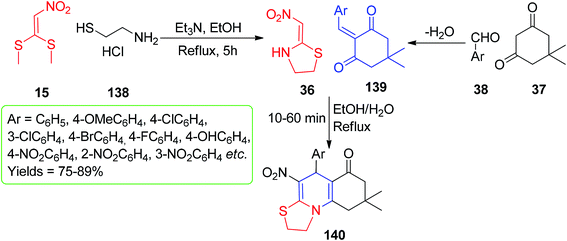 |
| Scheme 55 Synthesis of the fused 1,4-DHPs 140. | |
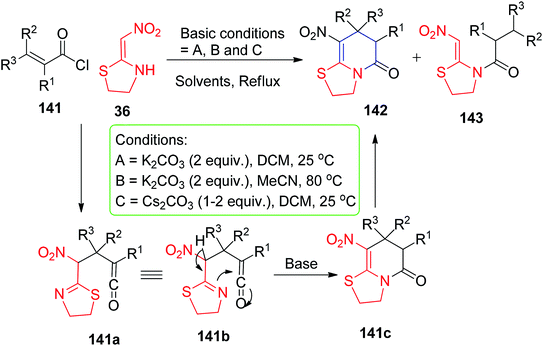 |
| Scheme 56 Synthesis of the functionalized pyridinone 142. | |
The base-promoted [3 + 3] cyclisation of the nitroketene N,S-acetals 36 with acryloyl or cinnamoyl chlorides 141 (1,3-dielectrophiles) provided the compounds 142 and 143 in good to excellent yields. However, they observed that (E)-3-methylbut-2-enoyl chloride and (E)-but-2-enoyl chloride afforded only the N-acylation products 143a and 143b and not the desired cyclic pyridinone compounds shown in Scheme 57.
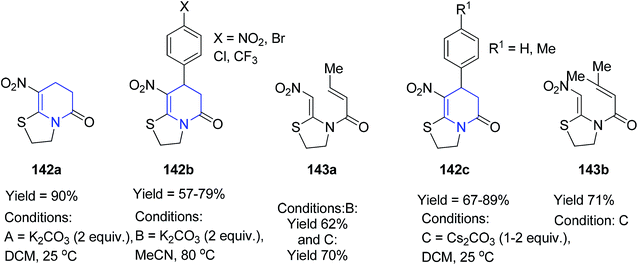 |
| Scheme 57 Diversity-oriented synthesis of functionalized pyridinones. | |
Similarly, a new class of pyridinone derivatives having a carboxylic acid group was constructed by Chakrabarti and his co-workers by the reaction of the itaconic anhydride 98 with the five- and six-membered cyclic nitroketene N,S-acetals 36/145 in MeCN (Scheme 58).68
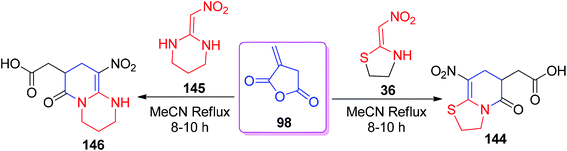 |
| Scheme 58 Synthesis of the functionalized pyridinone 144 from the itaconic anhydride 98. | |
Further investigation on the –COOH group-containing pyridinone derivatives was conducted by Zou and his group via a two-step procedure. In the first step, the compound 148 was synthesized by the reaction of the phthalic anhydride 147 with the ethyl cyanoacetate 126 using diisopropylamine (DIPA) as a catalyst in THF followed by reaction with SOCl2 in DCM.
In the next step, the starting materials 147 were heated in DMF at 70 °C with 36, and the expected cyclic products 149 were obtained in good to excellent yields (Scheme 59).87 Next, Yildirim et al. synthesized fused pyrimidine derivatives by green approaches using the five-membered cyclic nitroketene N,S-acetals 36. These pseudo-four-component reactions were prompted by microwave/conventional heating using 36 with the aromatic/aliphatic amines 151 and formaldehyde 150 in water (Scheme 60).88 In terms of yield and reaction time, MW heating provided better results than thermal heating. The synthesis of the bis pyrimidine 156 was also conducted with the yield of 100% upon MW heating of the aromatic diamine 155, formaldehyde and 36 (Scheme 61).
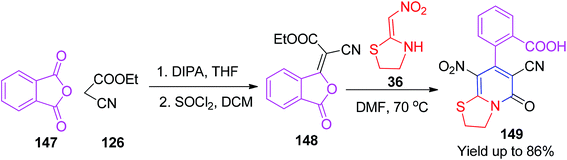 |
| Scheme 59 Synthesis of the functionalized 2-oxo-1,2-dihydropyridinone 149. | |
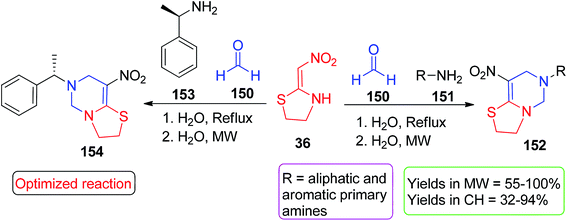 |
| Scheme 60 Synthesis of the functionalized pyrimidine derivatives 152/154. | |
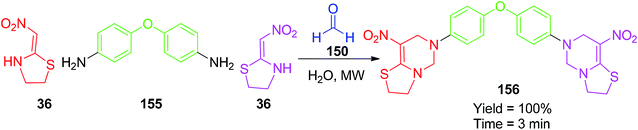 |
| Scheme 61 Synthesis of the functionalized bis pyrimidine derivative. | |
They also described a plausible mechanism, in which the imine 157 was formed by the reaction of formaldehyde 150 with the primary amine 151, and the imine intermediate reacted with 36 via Mannich addition to generate the intermediate 157a. This intermediate upon condensation with formaldehyde and intramolecular aza-Mannich addition reaction afforded the final product (Scheme 62).
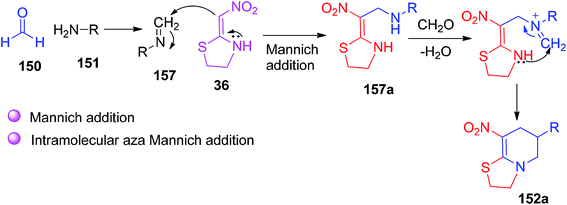 |
| Scheme 62 The proposed mechanism for product formation via Mannich addition. | |
Next, an interesting approach was reported by Fan and his group for the synthesis of the kinetic and thermodynamically fused pyrroles 158/159 by the condensation of the ninhydrin derivatives 113 with the cyclic nitroketene N,S-acetals 36 in ethanol at rt and in DMF at 75 °C, respectively (Scheme 63).89 The kinetic and thermodynamic products were further subjected to oxidation using NaIO4 and Pb(OAc)4 at room temperature to obtain the compounds 161 and 160, respectively.90
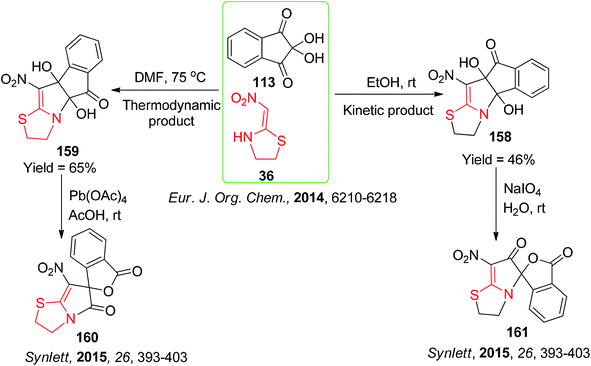 |
| Scheme 63 Synthesis of functionalized fused pyrroles and their functionalization. | |
4. Conclusions
Due to the synthetic and biological importance of nitroketene N,S-acetals, significant attention has been paid to their methods of preparation and applications. They are a robust building block for the construction of simple and fused heterocycles. The construction of highly stereo- and regioselective heterocycles via green synthetic procedures remains a vital challenge to chemists in recent times. Cyclic and acyclic functionalized nitroketene N,S-acetals take part in several commonly known reactions, such as Michael addition, 1,3-dipolar cycloaddition, heteroannulation reaction and also many cascade reactions, to afford novel N-, O- and S-containing heterocycles with high stereo- and regioselectivities. The formation of five-membered aromatic heterocycles, including pyrroles, thiophenes and non-aromatic dihydropyrroles, and six-membered 4H-pyran, chromene, chromenone, pyrazole-containing pyran, 1,4-DHP, pyrazole-fused pyridine, pyridinone and fused N,S-containing heterocycles, etc. from nitroketene N,S-acetals lead to outstanding functional group diversity, and these functional groups can be further functionalized to create complexity in the molecules; the graceful and original transformations have been recently reported along with the development of milder and safer access to heterocycles, and it is apparent that more of the scientific community will be enticed by this multitasking functional group.
We believe that these unique structural features have motivated organic chemists to explore nitroketene N,S-acetals to further heights, and the current overview is profoundly anticipated to boost the ongoing developments in this area of study.
Conflicts of interest
There are no conflicts to declare.
List of abbreviations
1,4-DHP | 1,4-Dihydropyridine |
AIBN | Azobisisobutyronitrile |
β-CD | β-Cyclodextrin |
CH | Conventional heating |
DBU | 1,8-Diazabicyclo[5.4.0]undec-7-ene |
DCM | Dichloromethane |
DIPA | Diisopropylamine |
DIPEA | Diisopropylethylamine |
DMF | N,N-Dimethylformamide |
DMSO | Dimethyl sulfoxide |
GAP | Group-assisted purification |
HIV | Human immunodeficiency viruses |
m-CPBA | m-Chloroperbenzoic acid |
MCR | Multicomponent reaction |
MW | Microwave |
NaH | Sodium hydride |
NMR | Nuclear magnetic resonance |
NMSM | (E)-N-Methyl-1-(methylthio)-2-nitroethenamine |
PEG | Polyethyleneglycol |
ROESY | Rotating-frame nuclear overhauser effect correlation spectroscopy |
SF | Solvent-free |
SNV | Substitution nucleophilic vinyl |
STA | Silica-supported tungstic acid |
TBTH | Tributyltin hydride |
THF | Tetrahydrofuran |
TSA | Toluenesulfonic acid |
Acknowledgements
Saigal and S. K. are grateful to the CSIR and UGC, respectively, for their Senior Research Fellowship (SRF). We are grateful to the referees and editors for their valuable comments and suggestions.
References
-
(a) E. K. Davison and J. Sperry, J. Nat. Prod., 2017, 80, 3060–3079 CrossRef CAS PubMed
;
(b) D. Havrylyuk, O. Roman and R. Lesyk, Eur. J. Med. Chem., 2016, 113, 145–166 CrossRef CAS PubMed
;
(c) Y. Ju and R. S. Varma, J. Org. Chem., 2006, 71, 135–141 CrossRef CAS PubMed
;
(d) G.-J. Mei and F. Shi, J. Org. Chem., 2017, 82, 7695–7707 CrossRef CAS PubMed
. -
(a) S. S. Panda, P. P. Mohapatra, A. R. Jones and P. Bachawala, Mini-Rev. Med. Chem., 2017, 17, 1515–1536 CrossRef CAS PubMed
;
(b) G.-J. Mei and F. Shi, Chem. Commun., 2018, 54, 6607–6621 RSC
. -
(a) M. M. Fouad, E. R. El-Bendary, G. M. Suddek, I. A. Shehata and M. M. El-Kerdawy, Bioorg. Chem., 2018, 81, 587–598 CrossRef CAS PubMed
;
(b) M. Rueping, E. Sugiono and E. Merino, Chem.–Eur. J., 2008, 14, 6329–6332 CrossRef CAS PubMed
. -
(a) M. Kaneda, S. Nakamura, N. Ezaki and Y. Iitaka, J. Antibiot., 1981, 34, 1366–1368 CrossRef CAS PubMed
;
(b) M. Koyama, N. Ezaki, T. Tsuruoka and S. Inouye, J. Antibiot., 1983, 36, 1483–1489 CrossRef CAS PubMed
. - G. Assaf, G. Cansell, D. Critcher, S. Field, S. Hayes, S. Mathew and A. Pettman, Tetrahedron Lett., 2010, 51, 5048–5051 CrossRef CAS
. - P. N. Kalaria, S. C. Karad and D. K. Raval, Eur. J. Med. Chem., 2018, 158, 917–936 CrossRef CAS PubMed
. -
(a) W. Li, S.-J. Zhao, F. Gao, Z.-S. Lv, J.-Y. Tu and Z. Xu, ChemistrySelect, 2018, 3, 10250–10254 CrossRef CAS
;
(b) S. Suzen and E. Buyukbingol, Farmaco, 1998, 53, 525–527 CrossRef CAS PubMed
. -
(a) P. Sharma, N. Rane and V. K. Gurram, Bioorg. Med. Chem. Lett., 2004, 14, 4185–4190 CrossRef CAS PubMed
;
(b) H. S. Basavaraja, G. M. Sreenivasa, E. Jayachandran and L. V. G. Nargund, Indian J. Heterocycl. Chem., 2005, 15, 69–70 Search PubMed
. - A. S. Sokolova, O. I. Yarovaya, N. I. Bormotov, L. N. Shishkina and N. F. Salakhutdinov, MedChemComm, 2018, 9, 1746–1753 RSC
. -
(a) L. W. Mohamed, A. T. Taher, G. S. Rady, M. M. Ali and A. E. Mahmoud, Der Pharma Chem., 2018, 10, 112–120 CAS
;
(b) F. Xie, H. Zhao, L. Zhao, L. Lou and Y. Hu, Bioorg. Med. Chem. Lett., 2009, 19, 275–278 CrossRef CAS PubMed
. - S. D. Joshi, H. M. Vagdevi, V. P. Vaidya and G. S. Gadaginamath, Eur. J. Med. Chem., 2008, 43, 1989–1996 CrossRef CAS PubMed
. - A. Goel, N. Agarwal, F. V. Singh, A. Sharon, P. Tiwari, M. Dixit, R. Pratap, A. K. Srivastava, P. R. Maulik and V. J. Ram, Bioorg. Med. Chem. Lett., 2004, 14, 1089–1092 CrossRef CAS PubMed
. - M. Amir, S. A. Javed and H. Kumar, Indian J. Pharm. Sci., 2007, 69, 337–343 CrossRef CAS
. -
(a) K. Last-Barney, W. Davidson, M. Cardozo, L. L. Frye, C. A. Grygon, J. L. Hopkins, D. D. Jeanfavre, S. Pav, C. G. Qian, J. M. Stevenson, L. Tong, R. Zindell and T. A. Kelly, J. Am. Chem. Soc., 2001, 123, 5643–5650 CrossRef CAS PubMed
;
(b) T. R. Reddy, C. Li, X. Guo, P. M. Fischer and L. V. Dekker, Bioorg. Med. Chem., 2014, 22, 5378–5391 CrossRef CAS PubMed
. -
(a) Y. C. Duan, Y. C. Ma, E. Zhang, X. J. Shi, M. M. Wang, X. W. Ye and H. M. Liu, Eur. J. Med. Chem., 2013, 62, 11–19 CrossRef CAS PubMed
;
(b) K. Benci, L. Mandic, T. Suhina, M. Sedic, M. Klobucar, S. K. Pavelic, K. Pavelic, K. Wittine and M. Mintas, Molecules, 2012, 17, 11010–11025 CrossRef CAS PubMed
;
(c) H. A. S. Abbas, W. A. El-Sayed and N. M. Fathi, Eur. J. Med. Chem., 2010, 45, 973–982 CrossRef CAS PubMed
. - D. Kumar, V. B. Reddy, S. Sharad, U. Dube and S. Kapur, Eur. J. Med. Chem., 2009, 44, 3805–3809 CrossRef CAS PubMed
. - P. D. Leeson and B. Springthorpe, Nat. Rev. Drug Discovery, 2007, 6, 881–890 CrossRef CAS PubMed
. -
(a) C. K. Z. Andrade and A. R. Dar, Tetrahedron, 2016, 72, 7375–7391 CrossRef CAS
;
(b) D. Prat, A. Wells, J. Hayler, H. Sneddon, C. R. McElroy, S. Abou-Shehadad and P. J. Dunne, Green Chem., 2016, 18, 288–296 RSC
. -
(a) L. Amenós, L. Trulli, L. Nóvoa, A. Parra and M. Tortosa, Angew. Chem., Int. Ed., 2019, 58, 3188–3192 CrossRef PubMed
;
(b) X.-Q. Chu, D. Ge, Z.-L. Shen and T.-P. Loh, ACS Catal., 2018, 8, 258–271 CrossRef CAS
;
(c) H.-H. Zhang, C.-S. Wang, C. Li, G.-J. Mei, Y. Li and F. Shi, Angew. Chem., Int. Ed., 2017, 56, 116–121 CrossRef CAS PubMed
;
(d) Z.-L. Shen, V. Dhayalan, A. D. Benischke, R. Greiner, K. Karaghiosoff, P. Mayer and P. Knochel, Angew. Chem., Int. Ed., 2016, 55, 5332–5336 CrossRef CAS PubMed
. -
(a) S. M. McElvain, Chem. Rev., 1949, 45, 453–492 CrossRef CAS
;
(b) H. Junjappa, H. Ila and C. V. Asokan, Tetrahedron, 1990, 46, 5423–5506 CrossRef CAS
. -
(a) M. Kolb, Synthesis, 1990, 171–190 CrossRef CAS
;
(b) K.-M. Wang, S.-J. Yan and J. Lin, Eur. J. Org. Chem., 2014, 1129–1145 CrossRef CAS
. -
(a) W. Fischer, R. Bodewei and G. Satzinger, Naunyn-Schmiedeberg's Arch. Pharmacol., 1992, 346, 442–452 CrossRef CAS
;
(b) X. Fei, Y. Gu, Y. Ban, Z. Liu and B. Zhang, Bioorg. Med. Chem., 2009, 17, 585–591 CrossRef CAS PubMed
;
(c) A. K. Jain, A. Vaidya, V. Ravichandran, S. K. Kashaw and R. K. Agrawal, Bioorg. Med. Chem., 2012, 20, 3378–3395 CrossRef CAS PubMed
;
(d) P. Agarwala, S. Pandey and S. Maiti, Org. Biomol. Chem., 2015, 13, 5570–5585 RSC
. -
(a) P. Jeschke, R. Nauen and M. E. Beck, Angew. Chem., Int. Ed., 2013, 52, 9464–9485 CrossRef CAS PubMed
;
(b) M. Tomizawa and J. E. Casida, J. Agric. Food Chem., 2011, 59, 2883–2886 CrossRef CAS PubMed
. - M. Kannan, P. Manivel, K. Geetha, J. Muthukumaran, H. S. P. Rao and R. Krishna, Journal of Chemical Biology, 2012, 5, 151–166 CrossRef CAS PubMed
. -
(a) L. Pan, X. Bi and Q. Liu, Chem. Soc. Rev., 2013, 42, 1251–1286 RSC
;
(b) L. Wang, W. He and Z. Yu, Chem. Soc. Rev., 2013, 42, 599–621 RSC
;
(c) H. Ila and H. Junjappa, Chimia, 2013, 67, 17–22 CrossRef CAS PubMed
. -
(a) H. Feist and P. Langer, Synthesis, 2007, 327–347 CAS
;
(b) L. Pan and Q. Liu, Synlett, 2011, 8, 1073–1080 Search PubMed
;
(c) L. Zhang, J. Dong, X. Xu and Q. Liu, Chem. Rev., 2016, 116, 287–322 CrossRef CAS PubMed
. -
(a) M. M. Khan, S. Khan, Saigal and S. Iqbal, RSC Adv., 2016, 6, 42045–42061 RSC
;
(b) R. C. Cioc, E. Ruijter and R. V. A. Orru, Green Chem., 2014, 16, 2958–2975 RSC
;
(c) M. M. Khan, R. Yousuf, S. Khan and Shafiullah, RSC Adv., 2015, 5, 57883–57905 RSC
. - R. Gompper and H. Schaefer, Chem. Ber., 1967, 100, 591–604 CrossRef CAS
. - R. C. Young, R. C. Mitchell, T. H. Brown, C. R. Ganellin, R. Griffiths, M. Jones, K. K. Ratm, D. Saundexs, I. R. Smith, N. E. Sore and T. J. Wilks, J. Med. Chem., 1988, 31, 656–671 CrossRef CAS PubMed
. - A. R. A. S. Deshmukh, T. I. Reddy, B. M. Bhawal, V. P. Shiralkar and S. Rajappa, J. Chem. Soc., Perkin Trans. 1, 1990, 1217–1218 RSC
. - T. I. Reddy, B. M. Bhawal and S. Rajappa, Tetrahedron, 1993, 49, 2101–2108 CrossRef CAS
. - S. P. Maybhate, A. R. A. S. Deshmukh and S. Rajappa, Tetrahedron, 1991, 47, 3887–3894 CrossRef CAS
. - S. G. Manjunatha, K. V. Reddy and S. Rajappa, Tetrahedron Lett., 1990, 31, 1327–1330 CrossRef CAS
. - J. M. Contreras, Y. M. Rival, S. Chayer, J. J. Bourguigon and C. G. Wermuth, J. Med. Chem., 1999, 42, 730–741 CrossRef CAS PubMed
. - D. P. Sangi and G. Correa, J. Braz. Chem. Soc., 2010, 21, 795–799 CrossRef CAS
. - D. P. Sangi, J. I. Monteiro, K. I. Vanzolini, Q. B. Cass, M. W. Paixao and A. G. Correa, J. Braz. Chem. Soc., 2014, 25, 887–889 CAS
. - T. Dunkern, S. Chavan, D. Bankar, A. Patil, P. Kulkarni, P. S. Kharkar, A. Prabhu, H. Goebel, E. Rolser, W. Burckhard-Boer, P. Arumugam and M. T. Makhija, J. Enzyme Inhib. Med. Chem., 2014, 29, 408–419 CrossRef CAS PubMed
. - K. Shiokawa, K. Moriya, K. Shibuya, Y. Hattori, S. Tsuboi and S. Kagabu, Biosci., Biotechnol., Biochem., 1992, 56, 1364–1365 CrossRef CAS
. - Z. T. Huang and X. Shi, Synthesis, 1990, 162–167 CrossRef CAS
. - M. M. Khan, B. Saigal, S. Shareef, S. Khan and S. C. Sahoo, Synth. Commun., 2018, 48, 2683–2694 CrossRef CAS
. - V. V. Shinde, D. Jeong, E. Cho and S. Jung, Tetrahedron, 2018, 74, 194–198 CrossRef CAS
. - J. Kamalraja, D. Muralidharan and P. T. Perumal, Synlett, 2012, 23, 2894–2898 CrossRef CAS
. - A. M. Jadhav, S. K. Krishnammagari, J. T. Kim and Y. T. Jeong, Tetrahedron, 2017, 73, 5163–5169 CrossRef CAS
. - J. Mao, J. Wang, W. Zhang, Z. Li, J. Zhu and C. Guo, ARKIVOC, 2016,(iii), 171–186 Search PubMed
. - J. P. Michael, Nat. Prod. Rep., 1999, 16, 697–709 RSC
. - I. S. Chen, S. J. Wu, I. J. Tsai, T. S. Wu, J. M. Pezzuto, M. C. Lu, H. Chai, N. Suh and C. M. Teng, J. Nat. Prod., 1994, 57, 1206–1211 CrossRef CAS
. - P. Gunasekaran, P. Prasanna, S. Perumal and A. I. Almansour, Tetrahedron Lett., 2013, 54, 3248–3252 CrossRef CAS
. - M. V. Reddy, G. D. Reddy, J. T. Kim and Y. T. Jeong, Tetrahedron, 2016, 72, 6484–6491 CrossRef CAS
. - S. Sivakumar, S. Kanchithalaivan and R. R. Kumar, RSC Adv., 2013, 3, 13357–13364 RSC
. - B. Jiang, C. G. Yang, W. N. Xiong and J. Wang, Bioorg. Med. Chem., 2001, 9, 1149–1154 CrossRef CAS PubMed
. - J. Kamalraja and P. T. Perumal, Tetrahedron Lett., 2014, 55, 3561–3564 CrossRef CAS
. - J. Wang, Z. Zhao, Z. Li, J. Mao, W. Zhang and C. Guo, Chem. Heterocycl. Compd., 2018, 54, 25–31 CrossRef CAS
. - S. Kanchithalaivan, S. Sivakumar, R. R. Kumar, P. Elumalai, Q. N. Ahmed and A. K. Padala, ACS Comb. Sci., 2013, 15, 631–638 CrossRef CAS PubMed
. - S. K. Krishnammagari, S. M. Lee and Y. T. Jeong, Res. Chem. Intermed., 2018, 44, 517–533 CrossRef CAS
. - N. Poomathi, J. Kamalraja, S. Mayakrishnan, D. Muralidharan and P. T. Perumal, Synlett, 2014, 25, 0708–0712 CrossRef CAS
. - D. N. Survase, H. V. Chavan, S. B. Dongare, S. D. Ganapure and V. B. Helavi, Iran. Chem. Commun., 2017, 5, 105–114 CAS
. - H. S. P. Rao and K. Geetha, Tetrahedron Lett., 2009, 50, 3836–3839 CrossRef CAS
. - H. S. P. Rao, K. Geetha and M. Kamalraj, RSC Adv., 2011, 1, 1050–1059 RSC
. - A. Parthiban, J. Muthukumaran, A. M. Priya, S. Jayachandran, R. Krishna and H. S. P. Rao, Med. Chem. Res., 2014, 23, 642–659 CrossRef CAS
. - K. Jayabal and T. P. Paramasivan, Tetrahedron Lett., 2014, 55, 2010–2014 CrossRef CAS
. - M. Kawase, A. Shah, H. Gaveriya, N. Motohashi, H. Sakagami and A. Varga, Bioorg. Med. Chem., 2002, 10, 1051–1055 CrossRef CAS PubMed
. - H. S. P. Rao and A. Parthiban, Org. Biomol. Chem., 2014, 12, 6223–6238 RSC
. - M. M. Khan, Saigal, S. Khan, S. Shareef and S. C. Sahoo, RSC Adv., 2018, 8, 41892–41903 RSC
. - K. Hajiyeva, A. Ismiev, M. Franz, M. Schmidtmann, J. Martens and A. Maharramov, Synth. Commun., 2017, 47, 1–5 CrossRef
. - P. Gunasekaran, P. Prasanna and S. Perumal, Tetrahedron Lett., 2014, 55, 329–332 CrossRef CAS
. - N. Poomathi, P. T. Perumal and S. Ramakrishna, Green Chem., 2017, 19, 2524–2529 RSC
. - A. M. Jadhav, Y. Il Kim, K. T. Lim and Y. T. Jeong, Tetrahedron Lett., 2018, 59, 554–557 CrossRef CAS
. - S. Chakrabarti, K. Panda, N. C. Misra, H. Ila and H. Junjappa, Synlett, 2005, 9, 1437–1441 Search PubMed
. - V. A. Sukach, A. V. Bol'but, A. Y. Petin and M. V. Vovk, Synthesis, 2007, 835–844 CAS
. - C. Venkatesh, B. Singh, P. K. Mahata, H. Ila and H. Junjappa, Org. Lett., 2005, 7, 2169–2172 CrossRef CAS PubMed
. - F. Yoneda, Y. Sakuma and K. Shinozuka, J. Chem. Soc., Chem. Commun., 1977, 681–683 RSC
. - J. H. da S. Rodrigues, T. Ueda-Nakamura, A. G. Correa, D. P. Sangi and C. V. Nakamura, PLoS One, 2014, 9, e85706 CrossRef PubMed
. - W. W. Wilkerson, R. A. Copeland, M. Covington and J. M. Trzaskos, J. Med. Chem., 1995, 38, 3895–3901 CrossRef CAS PubMed
. - S. J. Kaspersen, E. Sundby, C. Charnock and B. H. Hoff, Bioorg. Chem., 2012, 44, 35–41 CrossRef CAS PubMed
. - A. Chaudhary, J. M. Khurana, G. Khanna and M. Saroha, ChemistrySelect, 2018, 3, 6334–6337 CrossRef CAS
. - F. Rahimi, H. Hosseini and M. Bayat, Tetrahedron Lett., 2018, 59, 818–822 CrossRef CAS
. - V. Jeyachandran, M. Muthu and R. R. Kumar, Synth. Commun., 2015, 45, 1137–1144 CrossRef CAS
. - B. Balachandra, S. Shanmugam, T. Muneeswaran and M. Ramakritinan, RSC Adv., 2015, 5, 64781–64789 RSC
. - B. Balachandra and S. Shanmugam, ChemistrySelect, 2018, 3, 2037–2044 CrossRef CAS
. - N. C. Misra, K. Panda, H. Ila and H. Junjappa, J. Org. Chem., 2007, 72, 1246–1251 CrossRef CAS PubMed
. - S. V. Kumar, S. Muthusubramanian, J. C. Menéndez and S. Perumal, Beilstein J. Org. Chem., 2015, 11, 1707–1712 CrossRef CAS PubMed
. - C. Altug, A. K. Burnett, E. Caner, Y. Durust, M. C. Elliott, R. P. J. Glanville, C. Guy and A. D. Westwell, Tetrahedron, 2011, 67, 9522–9528 CrossRef CAS
. - Z. Tian, S. Cui and Z. Xu, Res. Chem. Intermed., 2014, 40, 1053–1059 CrossRef CAS
. - F. Sun, F. Zhu, X. Shao and Z. Li, Synlett, 2015, 26, 2306–2312 CrossRef CAS
. - M. Bayat, F. S. Hosseini and S. Nasri, J. Sulfur Chem., 2018, 39, 99–111 CrossRef CAS
. - M. Yildirim, D. Celikel, N. Evis and D. W. Knight, Tetrahedron, 2014, 17, 5674–5681 CrossRef
. - M.-M. Zou, F.-J. Zhu, X. Tian, L.-P. Ren, X.-S. Shao and Z. Li, Chin. Chem. Lett., 2014, 25, 1515–1519 CrossRef CAS
. - M. Yildirim, D. Celikel, Y. Durust and D. W. Knight, Tetrahedron, 2014, 70, 2122–2128 CrossRef CAS
. - N. Chen, M. Zou, X. Tian, F. Zhu, D. Jiang, J. Cheng, X. Shao and Z. Li, Eur. J. Org. Chem., 2014, 6210–6218 CrossRef CAS
. - Y. Fan, S. Liu, N. Chen, X. Shao, X. Xu and Z. Li, Synlett, 2015, 26, 393–403 CrossRef CAS
.
|
This journal is © The Royal Society of Chemistry 2019 |
Click here to see how this site uses Cookies. View our privacy policy here.