DOI:
10.1039/C9RA00653B
(Paper)
RSC Adv., 2019,
9, 5158-5163
Retracted Article: An efficient one pot three-component synthesis of 2,4,6-triarylpyridines using triflimide as a metal-free catalyst under solvent-free conditions†
Received
25th January 2019
, Accepted 6th February 2019
First published on 12th February 2019
Abstract
A simple and efficient protocol developed for one pot three-component synthesis of 2,4,6-triarylpyridines from aromatic aldehydes, substituted acetophenones and ammonium acetate using the versatile super Brønsted acid triflimide (HNTf2) as an effective catalyst is described. The reactions proceed well in the presence of 1 mol% of HNTf2 at 80 °C under solvent-free conditions and provide the corresponding triarylpyridines in good to excellent yields. The method reported has several advantages such as a metal-free and commercially available catalyst, mild reaction conditions and lower loading of catalyst.
Introduction
Substituted 2,4,6-triarylpyridines have been extensively exploited as chemosensors,1 photosensitizers,2 and intermediates in the synthesis of therapeutic drugs, insecticides, herbicides, and surfactants.3 Consequently, various synthetic methods have been developed for the synthesis of 2,4,6-triarylpyridines,4 such as the coupling of aryl aldehydes with aromatic keto-oxime acetates,5 oxidative cleavage of C–N bonds of benzyl amines with aromatic ketones,6 and the reactions of aromatic ketones with benzyl amines,7 acetophenoneoximes with aldehydes8 or epoxy styrenes,9 benzyl halides with acetophenones,10 and chalcones with enaminones.11 The cyclo-condensation reaction of acetophenones, benzaldehydes and ammonium acetate is known as one of the most conventional pathways for the synthesis of 2,4,6-triarylpyridines in the presence of various catalysts such as PEG1000-DAIL,12 ionic liquids,13 PFPAT,14 TrCl,15 MIL-101-SO3H,16 DPTA,17 ZrOCl2,18 TiO2–SO3H,19 TCT,20 or Fe3O4@TiO2@O2PO2(CH2)2NHSO3H,21 or without a catalyst.22 Although each of the above methods has its own merits, most of these methods are associated with certain drawbacks such as commercially unavailable catalysts, use of metals as catalysts and high reaction temperatures. To avoid such drawbacks, development of more simple and efficient protocols is still in demand.
Nowadays, one pot multicomponent reactions (MCRs) have received much attention for the synthesis of diverse compounds and contribute to sustainability by simplifying the synthetic route.23 MCRs combine three or more starting reagents at a time in the same pot to create the target molecule since there is no need of separating intermediate which help to reduce the energy consumption, solvent waste and reaction time and thus have the advantages of synthetic efficiency, simplicity, atom economy.
Triflimide (HNTf2), also known as bis(trifluoromethanesulfonyl)imide, is a commercially available white crystalline solid and highly versatile super Brønsted acid. Owing to its strong acidity as well as good compatibility with organic solvents, it has been widely employed as an exceptional catalyst in a wide range of organic reactions.24 Because of the presence of two strongly electron-withdrawing trifluoromethanesulfonyl groups, triflimide belongs to the superacid family. In recent years, specifically, triflimide has been extensively demonstrated as a superb catalyst in miscellaneous cycloaddition reactions such as [2 + 2],25 [3 + 2],26 [4 + 2],27 [3 + 3],28 [4 + 3],29 [6 + 2] (ref. 30) and [2 + 2 + 2] (ref. 31) cycloadditions. It is found that triflimide has also been used as a catalyst in numerous other organic reactions such as formation of oxime ethers,32 Mukaiyama aldol reaction,33 alkylation,34 synthesis of indanes and tetralins,35 allyl–allyl cross-coupling,36 C–C bond formation37 and Nazarov reaction.38 In view of the efforts toward development of the potential of HNTf2 as a catalyst, herein, we report an efficient one pot three-component synthesis of 2,4,6-triarylpyridines (Scheme 1).
 |
| Scheme 1 HNTf2 catalyzed one pot three-component synthesis of 2,4,6-triarylpyridines. | |
Results and discussion
In order to optimize the reaction conditions, the reaction of acetophenone, benzaldehyde and ammonium acetate was selected as a model reaction. The effect of solvent (Table 1), catalyst loading (Table 2), and temperature (Table 3) were monitored. The model reaction was examined in various solvent such as DMF, DMSO, PEG-400, toluene and under solvent-free conditions. Studies of solvent effect on the activity of catalyst reveal that the best yield was achieved under solvent-free conditions (entry 8, Table 1). Only 21% yield of the product was obtained in the absence of the catalyst (entry 1, Table 2). When 0.1 mol% of catalyst was used in model reaction, the corresponding 2,4,6-triphenylpyridine product was isolated in 43% yield. When the amount of catalyst has been increased from 0.1 to 0.5 mol%, 2,4,6-triphenylpyridine was obtained in 79% yield and the best performance was obtained when 1 mol% of catalyst was used (entry 4, Table 2). Further increase in catalyst concentration did not show any significant effect on yield (entry 5, Table 2). At room temperature a useful conversion was not observed (entry 1, Table 3). However, the productivity increased on raising the temperature. The best results were obtained at 80 °C (entry 4, Table 3).
Table 1 Effect of solvent on the synthesis of 2,4,6-triphenylpyridinea
Entry |
Solvent |
Yieldb (%) |
Reaction condition: benzaldehyde (1 mmol), acetophenone (2 mmol), ammonium acetate (1.5 mmol), solvent (3 mL), HNTf2 (1 mol%) at 80 °C for 50 min. Isolated yield. Reaction was performed under reflux condition. |
1 |
Acetonitrile |
23 |
2 |
DMF |
64 |
3 |
DMSO |
56 |
4c |
CH2Cl2 |
57 |
5 |
PEG-400 |
66 |
6 |
Toluene |
52 |
7 |
H2O |
62 |
8 |
None |
93 |
Table 2 Effect of catalyst loading on synthesis of 2,4,6-triphenylpyridinea
Entry |
Catalyst loading (mol%) |
Yieldb (%) |
Reaction condition: benzaldehyde (1 mmol), acetophenone (2 mmol), ammonium acetate (1.5 mmol), HNTf2 catalyst at 80 °C for 50 min under solvent-free conditions. Isolated yield. |
1 |
None |
21 |
2 |
0.1 |
43 |
3 |
0.5 |
79 |
4 |
1 |
93 |
5 |
1.5 |
93 |
Table 3 Effect of temperature on the synthesis of 2,4,6-triphenylpyridinea
Entry |
Temp. (°C) |
Yieldb (%) |
Reaction condition: benzaldehyde (1 mmol), acetophenone (2 mmol), ammonium acetate (1.5 mmol), HNTf2 (1 mol%) at different temperature for 50 min under solvent-free conditions. Isolated yield. |
1 |
Room temp. |
— |
2 |
45 |
36 |
3 |
60 |
67 |
4 |
80 |
93 |
5 |
85 |
93 |
On the basis of the above results, the catalytic activity of HNTf2 was further explored for various aromatic aldehydes and substituted acetophenones in the presence of 1 mol% of catalyst at 80 °C under solvent-free conditions. The results are summarized in Table 4. These results show that the reactions are equally facile with both electron-donating and electron-withdrawing substituents on the aromatic ring, and all products are obtained in good yields.
Table 4 Synthesis of 2,4,6-triarylpyridines catalysed by HNTf2a,b
A probable mechanism for the synthesis of 2,4,6-triarylpyridines has been proposed in Scheme 2. HNTf2 acts as an efficient Brønsted acid catalyst by activating the carbonyl group of an aromatic aldehyde and a substituted acetophenone to undergo aldol condensation to form a 1,3-diaryl-2-propen-1-one A, which further underwent Michael addition with substituted acetophenone to yield an intermediate B. Intermediate B is then cyclized and dehydrogenated to afford the title product 3.
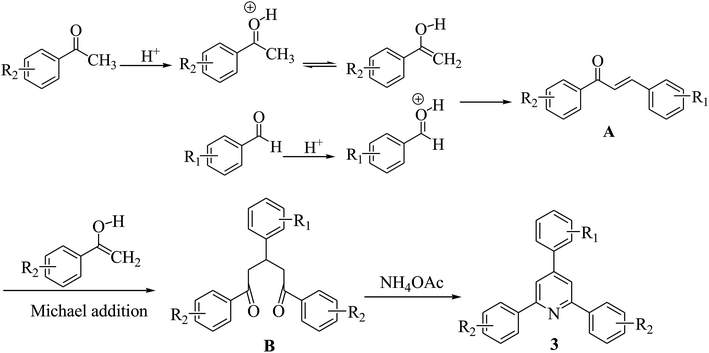 |
| Scheme 2 A plausible reaction mechanism. | |
The efficiency of the catalyst was determined by comparison with other catalytic systems. It gives a better yield at lower temperature (Table 5).
Table 5 Comparison of efficiency of HNTf2 with reported catalytic methods under solvent-free conditions
Entry |
Conditions |
Yield (%) |
Ref. |
1 |
120 °C, 3.5–7 h, H14[NaP5W30O110] |
50–98 |
39 |
2 |
120 °C, 4–6 h, HClO4/SiO2 |
68–88 |
40 |
3 |
120 °C, 15–120 min, BaCl2/nano-SiO2 |
70–94 |
41 |
4 |
120 °C, 6 h, I2 (20 mol%) |
48–61 |
42 |
5 |
120 °C, 1.5–3.5 h, [HO3S(CH2)4mim]HSO4 (20 mol%) |
82–93 |
13a |
6 |
130 °C, 4–7.5 h, wet TCT (5 mol%) |
58–86 |
20 |
7 |
120 °C, 4–7 h, DPAT (2 mol%) |
51–96 |
17 |
8 |
130 °C, 1–6 h, without catalyst |
60–86 |
22 |
9 |
110 °C, 5–9 min, MW, without catalyst |
80–95 |
43 |
10 |
110 °C, 80–150 min, n-TSA |
82–96 |
19 |
11 |
80 °C, 30–60 min, HNTf2 (1 mol%) |
81–96 |
This work |
Conclusions
In summary, we have disclosed that HNTf2 works as a powerful Brønsted acid catalyst for the one pot three-component synthesis of 2,4,6-triarylpyridines from aromatic aldehydes, substituted acetophenones and ammonium acetate under solvent-free conditions. Advantages of this methodology are use of metal-free and commercially available catalyst, simple experimental and work up procedure, high yields, mild reaction conditions and lower loading of catalyst compared with other methods. The methodology requires 1 mol% of HNTf2 catalyst, but it is not reused. This is a huge limitation.
Experimental
Materials and instrumentation
Materials obtained from commercial suppliers were used as received unless mentioned otherwise. All solvents were dried by standard procedures. Melting points were determined on an XT4A electrothermal apparatus equipped with a microscope and are uncorrected. NMR spectra were recorded on a Bruker Avance 400 spectrometer in CDCl3.
General procedure for the synthesis of 2,4,6-triarylpyridine
A mixture of aromatic aldehyde (1 mmol), acetophenone (2 mmol), ammonium acetate (1.5 mmol) and HNTf2 (1 mol%) was stirred at 80 °C for 30–60 min. The reaction was monitored by TLC (petroleum ether
:
ethyl acetate = 7
:
3, v/v). After completion, the reaction mixture was poured in ice water (5 mL) and the precipitated solid was collected by filtration, washed with distilled water (20 mL) and dried. The crude product was recrystallized from 95% ethanol (5 mL) to give the corresponding pure product. All the products were characterized by 1H NMR and 13C NMR spectroscopy.
Conflicts of interest
There are no conflicts to declare.
Acknowledgements
The authors gratefully acknowledge the financial support provided by Shaanxi Key Laboratory for Phytochemistry (17JS008) and Baoji University of Arts and Sciences (No. ZK14008).
Notes and references
- A. G. Fang, J. V. Mello and N. S. Finney, Tetrahedron, 2004, 60, 11075 CrossRef CAS.
- A. Islam, H. Sugihara and H. Arakawa, J. Photochem. Photobiol., A, 2003, 158, 131 CrossRef CAS.
- C. Doebelin, P. Wagner, F. Bihel, N. Humbert, C. A. Kenfack, Y. Mely, J. J. Bourguignon and M. Schmitt, J. Org. Chem., 2014, 79, 908 CrossRef CAS PubMed.
-
(a) C. Allais, J. M. Grassot, J. Rodriguez and T. Constantieux, Chem. Rev., 2014, 114, 10829 CrossRef CAS PubMed;
(b) J. Shen, D. Cai, C. Kuai, Y. Liu, M. Wei, G. Cheng and X. Cui, J. Org. Chem., 2015, 80, 6584 CrossRef CAS PubMed.
- Z. H. Ren, Z. Y. Zhang, B. Q. Yang, Y. Y. Wang and Z. H. Guan, Org. Lett., 2011, 13, 5394 CrossRef CAS PubMed.
-
(a) H. Huang, X. Ji, W. Wu, L. Huang and H. Jiang, J. Org. Chem., 2013, 78, 3774 CrossRef CAS PubMed;
(b) K. Gopalaiah, D. C. Rao, K. Mahiya and A. Tiwari, Asian J. Org. Chem., 2018, 7, 1872 CrossRef CAS.
- R. S. Rohokale, B. Koenig and D. D. Dhavale, J. Org. Chem., 2016, 81, 7121 CrossRef CAS PubMed.
- S. Mahernia, M. Mahdavi and M. Adib, Synlett, 2014, 25, 1299 CrossRef.
- S. Mahernia, M. Adib, M. Mahdavi and M. Nosrati, Tetrahedron Lett., 2014, 55, 3844 CrossRef CAS.
- M. Adib, N. Ayashi and P. Mirzaei, Synlett, 2016, 27, 417 CrossRef CAS.
- H. Zhang, J. Shen, G. Cheng, B. Wu and X. Cui, Asian J. Org. Chem., 2018, 7, 1089 CrossRef CAS.
- Y. M. Ren, Z. Zhang and S. Jin, Synth. Commun., 2016, 46, 528 CrossRef CAS.
-
(a) A. Davoodnia, M. Bakavoli, R. Moloudi, N. Tavakoli-Hoseini and M. Khashi, Monatsh. Chem., 2010, 141, 867 CrossRef CAS;
(b) S. P. Satasia, P. N. Kalaria and D. K. Raval, RSC Adv., 2013, 3, 3184 RSC;
(c) H. Behmadi, S. Naderipour, S. M. Saadati, M. Barghamadi, M. Shaker and N. Tavakoli-Hoseinia, J. Heterocycl. Chem., 2011, 48, 1117 CrossRef CAS.
- N. Montazeri and S. Mahjoob, Chin. Chem. Lett., 2012, 23, 419 CrossRef CAS.
- A. R. Moosavi-Zare, M. A. Zolfigol and Z. Rezanejad, Can. J. Chem., 2016, 94, 626 CrossRef CAS.
- M. B. Boroujeni, A. Hashemzadeh, M. T. Faroughi, A. Shaabani and M. M. Amini, RSC Adv., 2016, 6, 100195 RSC.
- J. Li, P. He and C. Yu, Tetrahedron, 2012, 68, 4138 CrossRef CAS.
- A. R. Moosavi-Zare, M. A. Zolfigol, S. Farahmand, A. Zare, A. R. Pourali and R. Ayazi-Nasrabadi, Synlett, 2014, 25, 193 CrossRef CAS.
- E. Tabrizian, A. Amoozadeh, S. Rahmani, E. Imanifar, S. Azhari and S. Malmir, Chin. Chem. Lett., 2015, 26, 1278 CrossRef CAS.
- B. Maleki, D. Azarifar, H. Veisi, S. F. Hojati, H. Salehabadi and R. N. Yami, Chin. Chem. Lett., 2010, 21, 1346 CrossRef CAS.
- M. A. Zolfigol, F. Karimi, M. Yarie and M. Torabi, Appl. Organomet. Chem., 2018, 32, 4063 CrossRef.
- M. Wang, Z. Yang, Z. Song and Q. Wanga, J. Heterocycl. Chem., 2015, 52, 907 CrossRef CAS.
-
(a) A. Magyar and Z. Hell, Synlett, 2019, 30, 89 CrossRef CAS;
(b) Y. Horino, M. Sugata, I. Mutsuura, K. Tomohara and H. Abe, Org. Lett., 2017, 19, 5968 CrossRef CAS PubMed;
(c) H. G. O. Alvim, J. R. Correa, J. A. F. Assumpcão, W. A. da Silva, M. O. Rodrigues, J. L. de Macedo, M. Fioramonte, F. C. Gozzo, C. C. Gatto and B. A. D. Neto, J. Org. Chem., 2018, 83, 4044 CrossRef CAS PubMed;
(d) L. S. Longo Jr and M. V. Craveiro, J. Braz. Chem. Soc., 2018, 29, 1999 Search PubMed;
(e) G. Kumar, E. Nikolla, S. Linic, J. W. Medlin and M. J. Janik, ACS Catal., 2018, 8, 3202 CrossRef CAS;
(f) Z. Long, L. Mao, M. Liu, Q. Wan, Y. Wan, X. Zhang and Y. Wei, Polym. Chem., 2017, 8, 5644 RSC;
(g) G. L. Wu and Q. P. Wu, Adv. Synth. Catal., 2018, 360, 1949 CrossRef CAS.
- W. Zhao and J. Sun, Chem. Rev., 2018, 118, 10349 CrossRef CAS PubMed.
-
(a) N. Shindoh, K. Kitaura, Y. Takemoto and K. Takasu, J. Am. Chem. Soc., 2011, 133, 8470 CrossRef CAS PubMed;
(b) K. Inanaga, K. Takasu and M. Ihara, J. Am. Chem. Soc., 2005, 127, 3668 CrossRef CAS PubMed;
(c) Y. Yoshii, T. Otsu, N. Hosokawa, K. Takasu, K. Okano and H. Tokuyama, Chem. Commun., 2015, 51, 1070 RSC.
-
(a) Y. Zhao, Y. Hu, C. Wang, X. Li and B. Wan, J. Org. Chem., 2017, 82, 3935 CrossRef CAS PubMed;
(b) Y. Zhao, Y. Hu, X. Li and B. Wan, Org. Biomol. Chem., 2017, 15, 3413 RSC.
-
(a) K. M. Reddy, E. Bhimireddy, B. Thirupathi, S. Breitler, S. Yu and E. J. Corey, J. Am. Chem. Soc., 2016, 138, 2443 CrossRef PubMed;
(b) B. Thirupathi, S. Breitler, K. M. Reddy and E. J. Corey, J. Am. Chem. Soc., 2016, 138, 10842 CrossRef CAS PubMed;
(c) S. Ponra, M. R. Vitale, V. Michelet and V. Ratovelomanana-Vidal, J. Org. Chem., 2015, 80, 3250 CrossRef CAS PubMed.
- T. Azuma, Y. Takemoto and K. Takasu, Chem. Pharm. Bull., 2011, 59, 1190 CrossRef CAS PubMed.
- R. Fuchigami, K. Namba and K. Tanino, Tetrahedron Lett., 2012, 53, 5725 CrossRef CAS.
- W. Zhao, Z. Wang and J. Sun, Angew. Chem., Int. Ed., 2012, 51, 6209 CrossRef CAS PubMed.
- Y. Wang, L. J. Song, X. Zhang and J. Sun, Angew. Chem., Int. Ed., 2016, 55, 9704 CrossRef CAS PubMed.
- M. S. Azizi and J. Cossy, Synlett, 2018, 29, 2417 CrossRef CAS.
-
(a) W. Gati and H. Yamamoto, Chem. Sci., 2016, 7, 394 RSC;
(b) H. Y. Bae and B. List, Chem.–Eur. J., 2018, 24, 13767 CrossRef CAS PubMed.
-
(a) S. Nomiyama and T. Tsuchimoto, Adv. Synth. Catal., 2014, 356, 3881 CrossRef CAS;
(b) T. E. Rose, B. H. Curtin, K. V. Lawson, A. Simon, K. N. Houk and P. G. Harran, Chem. Sci., 2016, 7, 4158 RSC.
- J. C. T. Reddel, W. Wang, K. Koukounas and R. J. Thomson, Chem. Sci., 2017, 8, 2156 RSC.
- F. Ding, R. William, F. Wang and X. W. Liu, Chem. Commun., 2012, 48, 8709 RSC.
- W. Zhao, H. Qian, Z. Li and J. Sun, Angew. Chem., Int. Ed., 2015, 54, 10005 CrossRef CAS PubMed.
- A. Jolit, S. Vazquez-Rodriguez, G. P. A. Yap and M. A. Tius, Angew. Chem., Int. Ed., 2013, 52, 11102 CrossRef CAS PubMed.
- M. M. Heravi, K. Bakhtiari, Z. Daroogheha and F. F. Bamoharram, Catal. Commun., 2007, 8, 1991 CrossRef CAS.
- L. Nagarapu, Aneesa, R. Peddiraju and S. Apuri, Catal. Commun., 2007, 8, 1973 CrossRef CAS.
- M. R. M. Shafiee and R. Moloudi, J. Chem. Res., 2011, 35, 294 CrossRef CAS.
- Y. M. Ren and C. Cai, Monatsh. Chem., 2009, 140, 49 CrossRef CAS.
- S. Tu, T. Li, F. Shi, F. Fang, S. Zhu, X. Wei and Z. Zong, Chem. Lett., 2005, 34, 732 CrossRef CAS.
Footnote |
† Electronic supplementary information (ESI) available. See DOI: 10.1039/c9ra00653b |
|
This journal is © The Royal Society of Chemistry 2019 |
Click here to see how this site uses Cookies. View our privacy policy here.