DOI:
10.1039/C9RA01122F
(Paper)
RSC Adv., 2019,
9, 12675-12680
A fluorescent and colorimetric probe based on naphthalene diimide and its high sensitivity towards copper ions when used as test strips†
Received
13th February 2019
, Accepted 8th April 2019
First published on 24th April 2019
Abstract
Herein, a red fluorescent and colorimetric probe (NDI-Py) based on naphthalene diimide was designed and synthesized, which exhibited rapid response, high sensitivity and selectivity towards copper ions, and the detection limit was as low as 0.97 μM in solution. Furthermore, NDI-Py demonstrated a strong red emission in the aggregated state because of its non-planar structure. Thus, it can act as a test strip to conveniently monitor copper ions with the detection limit as low as 2.0 μM.
Introduction
In the past few decades, extensive attention has been devoted to the detection of heavy metal ions due to their important biological and ecological relevance.1 For instance, overloading of copper ions in the human body can induce many serious diseases such as Parkinson's and prion diseases,2 Alzheimer's disease,3 and liver and kidney damage;4 according to the U.S. Environmental Protection Agency (EPA), the upper limit of copper ions in drinking water is 1.3 ppm (about 20 μM); thus, the development of copper-selective probes with high sensitivity is of great importance. To date, various probes have been constructed based on different conjugated systems, such as coumarin,5 1,8-naphthalimide,6 fluorescein,7 perylene8 and rhodamine derivatives9 and others,10 which are mainly related to fluorescence or colorimetric detection.11 Normally, colorimetric detection can be achieved by naked eyes, which has been proven to be more convenient and easier; on the other hand, fluorescent probes usually exhibit higher sensitivity due to fluorescence emission;12 thus, the combination of these two detection methods should be an excellent choice to realize more efficient monitoring of metal ions.
Accordingly, to realize the dual functional detection of copper ions (Chart 1), a strong electron pull–push system (NDI-Py) was constructed, in which naphthalene diimide (NDI) with a typical electron-deficient structure acted as an electron acceptor13 and 2-methylamino pyridine (Py) was employed as an electron donor and a receptor towards copper ions. The conjugation mode of these two moieties resulted in a strong intramolecular charge transfer (ICT) through the entire molecule; accordingly, the incorporation of an ester unit and an ether linkage into R1 was conducted to improve the solubility of the probe in water, and the branched unit in R2 could suppress the π–π stacking by steric hindrance, which was beneficial for the application of the proposed probe as an aggregated molecule. The resultant probe NDI-Py exhibited a strong red fluorescence with the maximum emission wavelength of 638 nm. This deep-red emission can be favourable for the possible applications of this probe in bioimaging. Upon the addition of trace copper ions, the fluorescence rapidly disappeared mainly due to the inherent paramagnetism of Cu2+, and the absorption spectrum red-shifted by about 60 nm with an obvious color change of solution from blue-purple to bluish-green. Furthermore, the probe NDI-Py could be applied into the silica gel plates to achieve the more convenient monitoring, and the detection limit was as low as 2.0 μM.
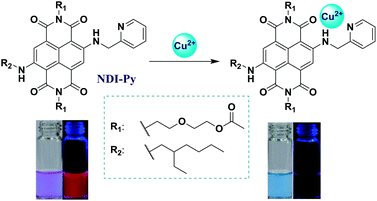 |
| Chart 1 The fluorescent and colorimetric responses of NDI-Py towards copper ions. | |
Results and discussion
Response of NDI-Py towards Cu2+
With the aim to investigate the optical properties of NDI-Py and its response towards copper ions, the absorption and fluorescence spectra of NDI-Py in the absence and presence of copper ions were obtained. As shown in Fig. 1, the solution of the probe NDI-Py in acetone (10 μM) exhibited lavender color with the maximum absorption wavelength at 603 nm. A strong red fluorescence could be observed with the maximum emission wavelength of 638 nm. Upon the addition of copper ions, the absorption spectrum red-shifted by about 60 nm along with a change in the solution color to bluish-green (Fig. 1A, inset). This indicated that NDI-Py could serve as a naked-eye indicator towards copper ions. Moreover, the fluorescence intensity of NDI-Py was quenched significantly (about 64-fold) and quickly (about 30 seconds) by the copper ions in trace amounts (Fig. 1B and S1†). These results suggested that NDI-Py could serve as a real-time colorimetric and fluorescent probe.
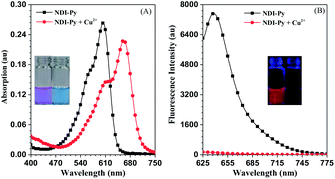 |
| Fig. 1 (A) The UV-visible spectra of NDI-Py in acetone (20 μM) in the absence and presence of 1.0 equiv. of copper ions. Inset: images of NDI-Py in the absence and presence of Cu2+; (B) the fluorescence spectra of NDI-Py in acetone (10 μM) in the absence and presence of 1.0 equiv. of copper ions. Inset: the fluorescence images of NDI-Py in the absence and presence of Cu2+. | |
Selectivity of NDI-Py towards copper ions
High selectivity of a probe for copper ions over other competing metal ions is of great significance for the practical applications of the probe, especially in biological systems and complex environmental sources. Thus, the selectivity of NDI-Py towards copper ions in the presence of various metal ions was examined by UV-visible absorption and fluorescence spectra. As shown in Fig. 2A and S3,† the copper ions could induce a red-shift in the absorption spectrum, whereas other metal ions, such as Li+, Ba2+, Co2+, Fe2+, Zn2+, Mg2+, Na+, Ca2+, K+, Pb2+, Cd2+, Cr3+, Al3+, Ag+, Mn2+ and Fe3+, showed almost no obvious interference. Although Hg2+ could induce a slight blue shift (16 nm) in the absorption spectrum, it still showed a negligible effect on the detection of Cu2+. The corresponding fluorescence responses are shown in Fig. 2B. After the addition of copper ions, a dramatic fluorescence quenching of about 64-fold was immediately observed. Nearly no obvious change in the fluorescence spectra was noted in the presence of other metal ions. In addition, the effect of various involved anions has been investigated (Fig. S4†), and nearly no fluorescence change can be observed upon the addition of sulfate, nitrate and hydrochloride. These results obtained by colorimetric and fluorescence detection demonstrated that NDI-Py exhibited high selectivity towards copper ions.
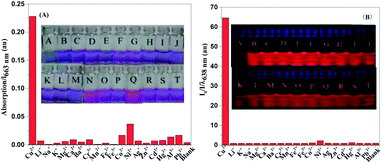 |
| Fig. 2 (A) The changes in the absorption of NDI-Py in acetone (20 μM) at 663 nm towards 1.0 equiv. of various metal ions. Inset: the images of NDI-Py with various metal ions: (A) Cu2+, (B) Li+, (C) Na+, (D) K+, (E) Mg2+, (F) Ca2+, (G) Ba2+, (H) Cr3+, (I) Mn2+, (J) Fe2+, (K) Fe3+, (L) Co2+, (M) Ni2+, (N) Ag+, (O) Zn2+, (P) Cd2+, (Q) Hg2+, (R) Al3+, (S) Pb2+, and (T) blank; (B) the profiles of fluorescence spectra of NDI-Py in acetone (10 μM) towards 1.0 equiv. of various metal ions. Inset: fluorescence images of NDI-Py with various metal ions under a UV lamp: (A) Cu2+, (B) Li+, (C) Na+, (D) K+, (E) Mg2+, (F) Ca2+, (G) Ba2+, (H) Cr3+, (I) Mn2+, (J) Fe2+, (K) Fe3+, (L) Co2+, (M) Ni2+, (N) Ag+, (O) Zn2+, (P) Cd2+, (Q) Hg2+, (R) Al3+, (S) Pb2+, and (T) blank. | |
The anti-interference ability is also an important factor for real applications. Contrast experiments in the presence of competing metal ions were conducted (Fig. 3). Copper ions could induce similar color and fluorescence changes in the presence of other competing metal ions as those caused by copper only. That is, other metal ions did not interfere with the detection of copper ions under competitive conditions. Furthermore, the fluorescence response of NDI-Py towards copper ions with the simultaneous existence of competing metal ions was investigated (Fig. S5†); upon the addition of various metals ions except for copper ions, the fluorescence intensity of NDI-Py exhibited a negligible change. When the copper ions were introduced, the fluorescence was significantly quenched even when the competing metal ions existed at the same time. These results further confirmed that NDI-Py could monitor copper ions with high selectivity in practical applications. Moreover, the sensing behavior of NDI-Py in an aqueous solution was investigated, and N-2-hydroxyethylpiperazine-N′-2-ethanesulfonic acid (HEPES) was chosen as a buffer in the neutral pH range. Since the addition of water could cause quenching of the fluorescence emission and red-shift of the fluorescence spectra (Fig. S6†) due to the larger polarity of water, an acetone: HEPES solution at the ratio of 7
:
3 was chosen as a suitable solvent system. Similarly, only copper ions could reduce the fluorescent intensity of NDI-Py by about 8-fold, whereas other metal ions had a negligible effect (Fig. S7†), and the high selectivity could also be proved by competitive experiments (Fig. S8†).
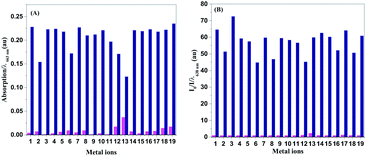 |
| Fig. 3 (A) The profiles of the UV-visible spectra of NDI-Py (20 μM) towards copper ions (1.0 equiv.) in the presence of other competing metal ions (1.0 equiv.); (B) the changes in the fluorescence intensity of NDI-Py (10 μM) towards copper ions (1.0 equiv.) in the presence of other competing metal ions (1.0 equiv.) (1) blank, (2) Li+, (3) Na+, (4) K+, (5) Mg2+, (6) Ca2+, (7) Ba2+, (8) Cr3+, (9) Mn2+, (10) Fe2+, (11) Fe3+, (12) Co2+, (13) Ni2+, (14) Ag+, (15) Zn2+, (16) Cd+, (17) Hg2+, (18) Al3+, and (19) Pb2+. The magenta bars represent the data obtained upon the addition of various metal ions, and the navy bars represent the data obtained upon the addition of copper ions in the presence of other competing metal ions. | |
Sensitivity of NDI-Py towards copper ions
The titration curve towards copper ions is shown in Fig. 4. At first, the absorption peak of NDI-Py in solution was located at 603 nm. With an increase in the amount of copper ions, absorption at 603 nm decreased gradually with the appearance of a new band at 663 nm (Fig. S9†). It was mainly attributed to the deprotonation of the N–H group in NDI-Py by copper ions, which could strengthen the ICT effect through the entire molecule. A similar phenomenon has also been reported in the literature.14 The absorption at 663 nm reached maximum when the concentration of copper ions was the same as that of NDI-Py; this suggested a 1
:
1 coordination in the complex NDI-Py-Cu2+. This was further confirmed by the Job's plot (Fig. S10†) and the ESI-MS spectrum (Fig. S11†). In the fluorescence spectra, the strong red fluorescence of NDI-Py with the peak at 638 nm could be decreased gradually with the dropwise addition of copper ions; this was mainly caused by the inherent paramagnetism of cooper ions, as reported in many studies.15 The detection limit of NDI-Py towards copper ions was calculated to be 7.1 μM, and it could be further improved to 0.96 μM by decreasing the concentration of the NDI-Py solution to 2.0 μM (Fig. S12 and S13†); this indicated high sensitivity of the probe towards copper ions. Note that this is much lower than the standard level of copper ions in the drinking water (20 μM) permitted by the U.S. EPA.
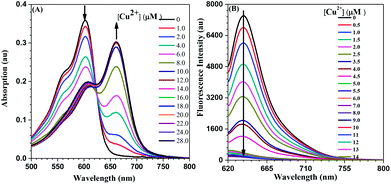 |
| Fig. 4 (A) The UV-visible spectra of NDI-Py (20 μM) in acetone with Cu2+ at different concentrations; (B) the fluorescence spectra of NDI-Py (10 μM) in acetone with Cu2+ at different concentrations. | |
Effect of pH on the fluorescence response of NDI-Py
To obtain insights into the influence of various pH values on the probe function, the fluorescence behaviors of NDI-Py and NDI-Py-Cu2+ under acidic or basic conditions were studied. The fluorescence intensity of NDI-Py showed negligible changes when the pH values varied from 1.03 to 12.77 (Fig. S14†). Upon the addition of copper ions, the corresponding fluorescence intensities of NDI-Py-Cu2+ decreased sharply when the pH values increased from 1.0 to 4.0 and remained stable at pH values ranging from 4.0 to 8.0. These results demonstrate that NDI-Py can monitor Cu2+ in a wide pH range and is suitable for practical applications under biological conditions.
The sensing behavior of NDI-Py in silica gel plates
In addition to the high sensitivity and selectivity of NDI-Py towards copper ions, it presented excellent photostability, as proved by the negligible fluorescence change under continuous irradiation (Fig. S2†). These excellent performances inspired us to further investigate the possible application of NDI-Py as test strips. Silica gel plates are a good molecular carrier, which exhibit non-toxicity, tastelessness, strong elasticity, and high thermal stability. After being immersed in the solution of NDI-Py (2.0 × 10−4 mol L−1) for 20 minutes and dried in air, the test strips were obtained by physical absorption. When they were exposed to UV light (365 nm), a strong red fluorescence in the aggregated state could be observed, revealing that the common aggregation-caused quenching (ACQ) effect as in many NDI-based molecules has been inhibited in NDI-Py.16 It is mainly due to the non-planar structure of NDI-Py, which can suppress the strong π–π stacking in the aggregated state. Then, solutions of copper ions with different concentrations were placed on the silica gel plates to form the word “NDI”. As shown in Fig. 5, with the increasing concentration of copper ions, dose-dependent turn-off progress of fluorescence was observed. Even when the concentration of copper ions was only 2.0 μM, the quenching effect could be recognized; when it further increased to 100 μM, the fluorescence was completely quenched; this proved that NDI-Py could detect copper ions in the test strips with high sensitivity.
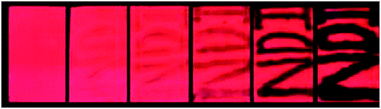 |
| Fig. 5 Images of silica gel plates with copper ions (“NDI” pattern) at different concentrations under a UV-lamp. From left to right, the concentrations of copper ions were 0, 2.0 × 10−6, 2.0 × 10−5, 1.0 × 10−4, 1.0 × 10−3, and 1.0 × 10−2 mol L−1. | |
Conclusions
In summary, we successfully synthesized a colorimetric and fluorescent probe, NDI-Py, based on naphthalene diimide for the fast detection of copper ions. The strong red fluorescence of NDI-Py could be sharply quenched by copper ions selectively with the detection limit as low as 0.97 μM along with the change of solution from blue-purple to bluish-green. Furthermore, it could be applied to silica gel plates to achieve a more convenient analysis for real samples.
Experimental
Materials and instrumentation
All the chemicals were used without further purification. Compound 1 was synthesized according to a method described in the literature.17 Tetrahydrofuran (THF) was dried over and distilled from the K–Na alloy under an atmosphere of dry argon. 1H and 13C NMR spectroscopy were conducted using the Varian Mercury 300 spectrometer, in which tetramethylsilane (TMS; δ = 0 ppm) served as the internal standard. The ultraviolet-visible (UV-visible) spectra were obtained using the Shimadzu UV-2550 spectrometer. The fluorescence spectra were acquired using the Hitachi F-4600 fluorescence spectrophotometer. The mass spectrum was obtained by Thermo DSQ II. Elemental analysis was performed by the CARLOERBA-1106 microelemental analyzer. ESI determination was carried out using the Finnigan LCQ advantage mass spectrometer. The HR-MS spectrum was obtained using the GCT premier CAB048 mass spectrometer (Scheme 1).
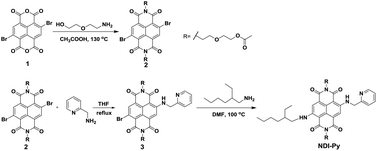 |
| Scheme 1 The synthetic routes of NDI-Py. | |
Synthesis of compound 2
Compound 1 (2.30 g, 5.3 mmol) was mixed with glacial acetic acid (80 mL) in a flask, and 2-(2-aminoethoxy)ethanol (2 mL) was added under stirring; the mixture was stirred at 130 °C for 6 hours under a nitrogen atmosphere; after cooling the abovementioned solution down to room temperature, a red solid was obtained by filtration under reduced pressure. At last, the red solid was further purified by column chromatography to obtain compound 2 as a slight-yellow solid (500 mg, 13.8%) with dichloromethane/acetone (40
:
1) as the eluent. 1H NMR (300 MHz, CDCl3, 25 °C) δ (ppm): 9.00 (s, 2H, ArH), 4.48 (t, J = 5.1 Hz, 4H, –O–CH2–), 4.19 (t, J = 4.5 Hz, 4H, –O–CH2–), 3.87 (t, J = 5.4 Hz, 4H, –O–CH2–), 3.75 (t, J = 7.8 Hz, 4H, –N–CH2–), 2.00 (s, 6H, –CH3).
Synthesis of compound 3
After compound 2 (0.27 g, 0.40 mmol) was dissolved in anhydrous THF (15 mL) in a flask, 2-methylamino pyridine (0.062 mL, 0.60 mmol) and K2CO3 (607 mg, 0.44 mmol) in anhydrous THF (15 mL) were added. The mixture was stirred at room temperature for 30 min and then refluxed for 6 h under a nitrogen atmosphere. THF was evaporated using a rotary evaporator, and a red solid was obtained. The crude product was further purified by column chromatography with dichloromethane/ethyl acetate (5
:
1) as the eluent to obtain compound 3 as a red solid (200 mg, 70.4%). 1H NMR (300 MHz, CDCl3, 25 °C) δ (ppm): 8.86 (s, 1H, ArH), 8.72 (br, 1H, ArH), 8.32 (s, 1H, ArH), 7.74 (t, J = 6.0 Hz, 1H, ArH), 7.38–7.26 (m, 2H, ArH), 4.94 (br, 2H, –N–CH2–), 4.50–4.42 (m, 4H,–O–CH2–), 4.19 (br, 4H, –O–CH2–), 3.85 (t, J = 5.7 Hz, 4H, –O–CH2–), 3.75 (br, 4H, –N–CH2–), 2.00 (s, 6H, –CH3). 13C NMR (75 MHz, CDCl3, 25 °C) δ (ppm): 171.03, 165.70, 161.94, 161.36, 155.15, 151.34, 149.89, 138.32, 137.13, 128.55, 127.26, 123.49, 121.67, 120.74, 100.69, 68.57, 67.76, 63.55, 48.31, 39.72, 20.94. MS (EI), m/z [M+]: calcd. 711.52, found: 711.57.
Synthesis of the compound NDI-Py
To compound 3 in DMF (15 mL) in a Schlenk tube (100 mL), 2-ethylhexylamine (0.4 mL, 0.30 mmol) was added dropwise. Then, the mixture was stirred at 100 °C for 4 hours under a nitrogen atmosphere. After being cooled down to room temperature, the resultant mixture was poured into water (100 mL). The mixture was extracted several times with dichloromethane and dried with anhydrous Na2SO4. After the solvent was removed by a rotary evaporator, the crude product was further purified by column chromatography with ethyl acetate/petroleum ether (v/v, 2
:
1) as the eluent to obtain NDI-Py as a blue solid (30 mg, 26.3%). 1H NMR (300 MHz, CDCl3, 25 °C) δ (ppm): 8.69 (s, 1H, ArH), 8.18 (d, J = 9.0 Hz, 2H, ArH), 7.70 (t, J = 7.5 Hz, 1H, ArH), 7.37–7.26 (m, 2H, ArH), 4.87 (br, 2H, –N–CH2–), 4.46 (t, J = 6.9 Hz, 4H, –O–CH2–), 4.19 (br, 4H, –O–CH2–), 3.86 (br, 4H, –O–CH2–), 3.75 (br, 4H, –N–CH2–), 2.00 (s, 6H, –CH3), 1.76 (br, 2H, –CH2–). 13C NMR (75 MHz, CDCl3, 25 °C) δ (ppm): 170.89, 165.77, 162.74, 156.23, 149.62, 149.32, 148.19, 136.82, 125.48, 125.22, 122.51, 121.54, 121.13, 120.71, 118.02, 102.33, 101.09, 68.39, 67.75, 63.54, 48.23, 46.06, 38.90, 31.13, 29.59, 28.77, 24.39, 22.92, 20.79, 13.99, 10.86. MS (EI), m/z [M+]: 759.35, found: 759.54. Anal. calcd. for C40H49N5O10: C 63.23, H 6.50, N 9.22, found: C 63.02, H 6.38, N 9.11. HRMS, m/z [M + 1]+ calcd. 760.3552, found: 760.3558.
Solution preparation and optical measurements
NDI-Py (3.0 mg, 4.0 μmol) was dissolved in acetone (10 mL) to obtain a stock solution with the concentration of 4.0 × 10−4 mol L−1, which could be diluted to desired concentrations with an appropriate solvent medium. The stock solutions of various metal ions were prepared by dissolving corresponding metal salts, such as NaNO3, KNO3, LiCl, Ba(NO3)2, AgNO3, Cr(NO3)3·9H2O, CoCl2·6H2O, Pb(NO3)2, Ni(NO3)2·6H2O, Zn(NO3)2·6H2O, Cu(NO3)2·3H2O, Al(NO3)3·9H2O, Fe(NO3)3·9H2O, MnSO4·2H2O, Cd(NO3)2·4H2O, FeSO4·7H2O, MgSO4, CaCl2, NiCl2·6H2O and Hg(ClO4)2·3H2O, in deionized water. The absorption and fluorescence spectra were obtained after the corresponding solutions were shaken well and incubated for 1 min. The excitation wavelength was 605 nm, and the excitation and emission slits were 5 nm each. The fluorescence emission was observed from 620 nm to 800 nm.
Conflicts of interest
The authors declare no competing financial interest.
Acknowledgements
We are grateful to the National Science Foundation of China (51673151) and the Fundamental Research Funds for the Central Universities (2042018kf0014) for providing the financial support.
Notes and references
-
(a) L. Li, B. Yu and T. You, Biosens. Bioelectron., 2015, 74, 263 CrossRef CAS PubMed;
(b) H. Cheng and Y. Qian, Sens. Actuators, B, 2015, 219, 57 CrossRef CAS;
(c) J. Ru, X. Mi, L. Guan, X. Tang, Z. Ju, G. Zhang, C. Wang and W. Liu, J. Mater. Chem. B, 2015, 3, 6205 RSC;
(d) H. Chang, Y. Chang, N. Fan and J. A. Ho, ACS Appl. Mater. Interfaces, 2014, 6, 18824 CrossRef CAS PubMed;
(e) N. Kumari, N. Dey and S. Bhattacharya, RSC Adv., 2014, 4, 4230 RSC;
(f) M. P. Algi, Z. Öztas and F. Algi, Chem. Commun., 2012, 48, 10219 RSC;
(g) J. Liu and Y. Lu, J. Am. Chem. Soc., 2003, 125, 6642 CrossRef CAS PubMed;
(h) A. Coskun and E. U. Akkaya, J. Am. Chem. Soc., 2006, 128, 14474 CrossRef CAS PubMed;
(i) H. Li, J. Fan and X. Peng, Chem. Soc. Rev., 2013, 42, 7943 RSC;
(j) Q. He, E. W. Miller, A. P. Wong and C. J. Chang, J. Am. Chem. Soc., 2006, 128, 9316 CrossRef CAS PubMed.
-
(a) D. R. Brown and H. Kozlowski, Dalton Trans., 2004, 1907 RSC;
(b) G. L. Millhauser, Acc. Chem. Res., 2004, 37, 79 CrossRef CAS PubMed;
(c) E. Gaggelli, H. Kozlowski, D. Valensin and G. Valensin, Chem. Rev., 2006, 106, 1995 CrossRef CAS PubMed.
- K. C. Ko, J. Wu, H. J. Kim, P. S. Kwon, J. W. Kim, R. A. Bartsch, J. Y. Lee and J. S. Kim, Chem. Commun., 2011, 47, 3165–3167 RSC.
-
(a) M. Taki, S. Iyoshi, A. Ojida, I. Hamachi and Y. Yamamoto, J. Am. Chem. Soc., 2010, 132, 5938 CrossRef CAS PubMed;
(b) Y. H. Hung, A. I. Bush and R. A. Chern, J. Biol. Inorg Chem., 2010, 15, 61 CrossRef CAS PubMed.
-
(a) S. N. K. Elmas, F. Ozen, K. Koran, I. Yilmaz, A. O. Gorgulu and S. Erdemir, J. Fluoresc., 2017, 27, 463 CrossRef PubMed;
(b) O. García-Beltrán, N. Mena, L. C. Friedrich, J. C. Netto-Ferreira, V. Vargas, F. H. Quina, M. T. Núñez and B. K. Cassels, Tetrahedron Lett., 2012, 53, 5280 CrossRef;
(c) H. S. Jung, P. S. Kwon, J. W. Lee, J. I. Kim, C. S. Hong, J. W. Kim, S. Yan, J. Y. Lee, J. H. Lee, T. Joo and J. S. Kim, J. Am. Chem. Soc., 2009, 131, 2008 CrossRef CAS PubMed.
-
(a) C. Liu, X. Jiao, S. He, L. Zhao and X. Zeng, Org. Biomol. Chem., 2017, 15, 3947 RSC;
(b) Y. Fu, C. Fan, G. Liu and S. Pu, Sens. Actuators, B, 2017, 239, 295 CrossRef CAS.
-
(a) K. Huang, Y. Yue, X. Jiao, C. Liu, Q. Wang, S. He, L. Zhao and X. Zeng, Dyes Pigm., 2017, 143, 379 CrossRef CAS;
(b) L. E. McQuade and S. J. Lippard, Inorg. Chem., 2010, 49, 7464 CrossRef CAS PubMed;
(c) Z. Fu, L. Yan, X. Zhang, F. Zhu, X. Han, J. Fang, Y. Wang and Y. Peng, Org. Biomol. Chem., 2017, 15, 4115 RSC.
-
(a) S. Goswami, S. Chakraborty, S. Paul, S. Halder, S. Panja and S. K. Mukhopadhyay, Org. Biomol. Chem., 2014, 12, 3037 RSC;
(b) Y. Wang, L. Zhang, G. Zhang, Y. Wu, S. Wu and L. Wang, Tetrahedron Lett., 2014, 55, 3218 CrossRef CAS.
-
(a) Y. Mi, Z. Cao, Y. Chen, Q. Xie, Y. Xu, Y. Luo, J. Shi and J. Xiang, Analyst, 2013, 138, 5274 RSC;
(b) J. Sun, H. Mei and F. Gao, Biosens. Bioelectron., 2017, 91, 70 CrossRef CAS PubMed;
(c) B. Zhang, Q. Diao, P. Ma, X. Liu, D. Song and X. Wang, Sens. Actuators, B, 2016, 225, 579 CrossRef CAS;
(d) L. Huang, F. Chen, P. Xi, G. Xie, Z. Li, Y. Shi, M. Xu, H. Liu, Z. Ma, D. Bai and Z. Zeng, Dyes Pigm., 2011, 90, 265 CrossRef CAS.
-
(a) L. Zeng, E. W. Miller, A. Pralle, E. Y. Isacoff and C. J. Chang, J. Am. Chem. Soc., 2006, 128, 10 CrossRef CAS PubMed;
(b) K. M. K. Swamy, S.-K. Ko, S. K. Kwon, H. N. Lee, C. Mao, J.-M. Kim, K.-H. Lee, J. Kim, I. Shin and J. Yoon, Chem. Commun., 2008, 5915 RSC;
(c) G. J. Park, G. R. You, Y. W. Choi and C. Kim, Sens. Actuators, B, 2016, 229, 257 CrossRef CAS.
-
(a) X. Zhu, Y. Duan, P. Li, H. Fan, T. Han and X. Huang, Anal. Methods, 2019, 11, 642 RSC;
(b) H. Ye, F. Ge, Y. Zhou, J. Liu and B. Zhao, Spectrochim. Acta, Part A, 2013, 112, 132 CrossRef CAS PubMed;
(c) Z. Zhang, Y. Liu and E. Wang, Dyes Pigm., 2019, 163, 533 CrossRef CAS;
(d) X. Meng, D. Cao, Z. Hua, X. Han, Z. Li, D. Liang and W. Ma, Tetrahedron Lett., 2018, 59, 4299 CrossRef CAS;
(e) M. Wang, D. Zhang, M. Li, M. Fan, Y. Ye and Y. Zhao, J. Fluoresc., 2013, 23, 417 CrossRef CAS PubMed;
(f) B. Gu, L. Huang, Z. Xu, Z. Tan, M. Hu, Z. Yang, Y. Chen, C. Peng, W. Xiao, D. Yu and H. Li, Sens. Actuators, B, 2018, 273, 118 CrossRef CAS.
-
(a) D. Zhu, Y. Luo, X. Yan, W. Xie, W. Cai and X. Zhong, RSC Adv., 2016, 6, 87110 RSC;
(b) X. Wang, X. Ma, Z. Yang, Z. Zhang, J. Wen, Z. Geng and Z. Wang, Chem. Commun., 2013, 49, 11263 RSC.
-
(a) Z. Yuan, Y. Ma, T. Geßner, M. Li, L. Chen, M. Eustachi, R. T. Weitz, C. Li and K. Müllen, Org. Lett., 2016, 18, 456 CrossRef CAS PubMed;
(b) Z. Zhao, Z. Wang, C. Ge, X. Zhang, X. Yang and X. Gao, Polym. Chem., 2016, 7, 573 RSC;
(c) C. R. DeBlase, K. Hernández-Burgos, J. M. Rotter, D. J. Fortman, D. S. Abreu, R. A. Timm, I. C. N. Diógenes, L. T. Kubota, H. D. Abruña and W. R. Dichte, Angew. Chem., Int. Ed., 2015, 54, 13225 CrossRef CAS PubMed;
(d) B. Xiao, G. Ding, Z. Tan and E. Zhou, Polym. Chem., 2015, 6, 7594 RSC;
(e) H. Kar, M. R. Molla and S. Ghosh, Chem. Commun., 2013, 49, 4220 RSC;
(f) D. Asthana, J. Shukla, S. Dana, V. Rani, M. R. Ajayakumar, K. Rawat, K. Mandal, P. Yadav, S. Ghosh and P. Mukhopadhyay, Chem. Commun., 2015, 51, 15237 RSC;
(g) M. A. Kobaisi, S. V. Bhosale, K. Latham, A. M. Raynor and S. V. Bhosale, Chem. Rev., 2016, 116, 11685 CrossRef CAS PubMed;
(h) L. Zong, Y. Song, Q. Li and Z. Li, Sens. Actuators, B, 2016, 226, 239 CrossRef CAS;
(i) L. Zong, Y. Xie, Q. Li and Z. Li, Sens. Actuators, B, 2017, 238, 735 CrossRef CAS;
(j) N. Li, L. Zong, Q. Li and Z. Li, Sens. Actuators, B, 2015, 207, 827 CrossRef CAS.
-
(a) F. Doria, V. Amendola, V. Grande, G. Bergamaschi and M. Freccer, Sens. Actuators, B, 2015, 212, 137 CrossRef CAS;
(b) Z. Xu, X. Qian and J. Cui, Org. Lett., 2005, 7, 3029 CrossRef CAS PubMed;
(c) J. Wang, Y. Xie, Z. Wang and Q. Song, Sens. Actuators, B, 2014, 194, 149 CrossRef CAS.
-
(a) Q. Li, Y. Guo and S. Shao, Sens. Actuators, B, 2012, 171–172, 872 CrossRef CAS;
(b) X. Wu, Z. Guo, Y. Wu, S. Zhu, T. D. James and W. Zhu, ACS Appl. Mater. Interfaces, 2013, 5, 12215 CrossRef CAS;
(c) J. H. Jang, S. Bhuniya, J. Kang, A. Yeom, K. S. Hong and J. S. Kim, Org. Lett., 2013, 15, 4702 CrossRef CAS PubMed.
-
(a) Q. Zhao, S. Zhang, Y. Liu, J. Mei, S. Chen, P. Lu, A. Qin, Y. Ma, J. Sun and B. Tang, J. Mater. Chem., 2012, 22, 7387 RSC;
(b) G. Zhang, Z. Chen, M. P. Aldred, Z. Hu, T. Chen, Z. Huang, X. Meng and M. Zhu, Chem. Commun., 2014, 50, 12058 RSC;
(c) L. Zong, Y. Xie, C. Wang, J.-R. Li, Q. Li and Z. Li, Chem. Commun., 2016, 52, 11496 RSC.
- Q. Li, M. Peng, H. Li, C. Zhong, L. Zhang, X. Cheng, X. Peng, Q. Wang, J. Qin and Z. Li, Org. Lett., 2012, 14, 2094 CrossRef CAS PubMed.
Footnote |
† Electronic supplementary information (ESI) available: The UV-visible, fluorescence spectra, the job's plot and the characteristic of the structure of NDI-Py. See DOI: 10.1039/c9ra01122f |
|
This journal is © The Royal Society of Chemistry 2019 |