DOI:
10.1039/C9RA04535J
(Paper)
RSC Adv., 2019,
9, 26136-26141
Performance of selenate removal by biochar embedded nano zero-valent iron and the biological toxicity to Escherichia coli†
Received
17th June 2019
, Accepted 14th August 2019
First published on 21st August 2019
Abstract
The application of nano zero-valent iron (nZVI) in water environment was limited by its easily aggregation and potential biological toxicity. In this study, biochar embedded nZVI (BC-nZVI) was prepared by carbon-thermal reduction method, and the SEM-EDX mapping results showed that nZVI was successfully embedded on biochar. Meanwhile, BC-nZVI with the optimal Fe/C of 2/1 showed a similar Se(VI) removal efficiency to pure nZVI. Effects of pH, BC-nZVI loading, and initial Se(VI) concentration were studied. Se(VI) removal rates (at 30 min) by BC-nZVI at pH 4.0 and 5.0 were 98.2% and 95.9%, respectively. But Se(VI) removal rate (at 30 min) was sharply decreased to 25.8% at pH 6.0. With the increase of BC-nZVI loading from 0.5 g L−1 to 1 g L−1, Se(VI) removal rate (at 30 min) significantly increased from 25.5% to 95.9%. And the continuous increase of BC-nZVI loading to 2 g L−1 did not improve Se(VI) removal rate. Se(VI) less than 3 mg L−1 was completely removed by BC-nZVI in 30 min, but Se(VI) more than 6 mg L−1 only was removed about 25.9% at 30 min. Optimal parameters were pH 4.0, 2 g L−1 BC-nZVI, and 1.5 mg L−1 Se(VI). Variation of calculated amount, SOD activity, and protein content of Escherichia coli with nZVI and BC-nZVI indicated that nZVI and BC-nZVI both produced negative effects on the growth of E. coli. But the amount and SOD activity of E. coli with pure nZVI was lower than that with BC-nZVI. Moreover, E. coli with nZVI released more protein than that with BC-nZVI. So modified nZVI by biochar was less harmful to E. coli than nZVI.
Introduction
Selenium (Se) as a non-metallic element is very important to immune systems of human, animals, and plants. The supplement of Se may boost immunity and prevent myelopathy such as Keshan disease,1 Kashin-Beck disease,2 and rheumatoid arthritis.3 And recommended daily Se intakes are 30–40 μg d−1 in China, and 11–70 μg d−1 in some European countries.4 But excess Se (>400 μg d−1) is harmful to organisms.5 In addition, Se and Se compounds have been listed in IRAC group 3 since 1987.6 Sources of Se in water environment mainly include natural processes7 via storm water runoff, inflow, or infiltration from mining operations and arid soils,8 the use of chemicals or medicines containing Se9 such as herbicides, insecticides, anti-fungal medicines, and anti-dandruff shampoos,7 and industrial wastewater10 of electronics, petroleum refining, oxidation of sulfide minerals, and copper smelting.4 Concentrations of Se contaminations in various industrial wastewater resources reach mg L−1,11 and average selenium concentration in groundwater ranges from ng L−1 to μg L−1.12 The maximum contamination level of Se in drinking water has been set at 10, 40, and 50 μg L−1 by European Union,13 WHO14 (World Health Organization), and the USA,15 respectively. Therefore, Se contamination in water has attracted a widespread concern and studies on Se removal from aquatic environment would be necessary.
Generally, element Se exists in various valence states and form including selenide (Se(II)), elemental Se (Se(0)), selenite (Se(IV)), selenate (Se(VI)), and several organic selenium compounds such as selenomethionine and dimethyl selenide. Se(VI) and Se(IV), as main species of Se in water, possesses high solubility, bioavailability, and toxicity.16 Although the acute toxicity of Se(IV) is much higher than that of Se(VI), the conversion between Se(VI) and Se(IV) might occur in aerobic or anaerobic environment. So Se(VI) and Se(IV) might coexist in anaerobic water in comparable concentrations.17 Se(IV) can be more easily absorbed by iron oxide than Se(VI), and Se(VI) with high transfer ability in water is more harmful to various aquatic lives and human. Therefore, it is necessary to develop efficient strategies to remove Se(VI) from water environment.
Compared to Se(VI) removal technologies such as microbiological reduction, chemical coagulation, ion exchange, and reverse osmosis, zero valent iron (ZVI) exhibits more advances in Se(VI) removal for its low cost, easy handling, and ready availability. ZVI as a moderately strong reducing agent could directly reduce Se(VI) to Se(IV) or Se(0), and new generated Se(IV) can be reduced to Se(0) by ZVI or new generated Fe(II) on ZVI surface.18,19 Furthermore, Se(IV) or Se(0) can also be absorbed or co-precipitated by iron oxides or hydroxides generated during the corrosion of ZVI. Thus, Se(VI) can be completely removed by ZVI/H2O systems via reduction, absorption, and co-precipitation. But ZVI in the form of iron turning or powder always exhibits a low reactivity towards contaminants for its low specific surface area. The synthesis of nano ZVI (nZVI) can extensively improve the reactivity of ZVI, and enhances the performance of contaminants removal.20 And many studies of the removal of heavy metals, halogenated organics, and dyes by nZVI have been reported.21 Although nZVI shows more excellent reactivity than micro iron power or iron turning, the safety and aggregation of aquatic nZVI limited the engineering application of nZVI in water treatment.22 In particular, the ecotoxicity of nZVI has aroused many researchers' concern. Lee et al.23 reported that nZVI in water could rapidly inactivated Escherichia coli (E. coli), and the bactericidal effect was also observed in other types of iron based compounds.24 El-Temsah and Joner25 stated that nZVI had acute adverse effects on earthworms and aged or oxidized nZVI could decrease its toxicity to earthworms. Zhou et al.26 found that nZVI generated reactive oxygen species (ROS) to discharge mitigated obviously the membrane fouling of MBR. Thus the potential ecotoxicity of nZVI should be evaluated during Se(VI) removal to increase the potentiality of nZVI in application. Carbon materials were an ideal carrier of nZVI for its stability, high mechanical strength, and good conductivity.27 So the synthesis of biochar embedded nZVI (BC-nZVI) might reduce the aggregation and ecotoxicity of nZVI.28 But few researches study Se(VI) removal by BC-nZVI and the biological toxicity of BC-nZVI is still unclear.
Thus, the objectives of this study were to (1) characterize nZVI and BC-nZVI samples, (2) investigate optimal proportion of iron and carbon (Fe/C) of BC-nZVI, (3) study different solution factors on Se(VI) removal by BC-nZVI, and (4) evaluate biological toxicity of BC-nZVI and nZVI to E. coli.
Experimental
Reagent
Ferrous sulfate, sodium borohydride purchased from Sinopharm Chemical Reagent Co., Ltd. (Shanghai, China) were of analytical grade. Biochar were made by peanut shell at 500 °C. (Particle size distribution and elemental analysis of biochar are shown in Fig. S1 and Table S1,† respectively.) Sodium selenate was supplied by Sigma-Aldrich Co. LLC. (St. Louis, USA). Other chemicals used in this study were obtained from Shanghai Qiangshun Chemical Reagent Company (Shanghai, China). Stock solutions were dissolved in DI (de-ionized) water.
Synthesis of nZVI and BC-nZVI
The BC-nZVI was prepared by carbo-thermal reduction method. Firstly, N2 was purged into 140 mL deionized water for 30 min in a 500 mL three-necked flask. Then a definite amount of FeSO4·7H2O and 35 mL ethanol was added into the flask. And a fixed proportion of biochar was mixed and continuously stirred by a magnetic stirrer at 200 rpm. After 1 h, 50 mL NaBH4 (50 g L−1) was added into the above solution at a uniform rate of 1–2 drops per second under the protection of N2. After aging for 1 h, BC-nZVI was washed a number of times with deoxygenated DI water and ethanol under the protection of N2. Finally, BC-nZVI was sealed after freeze-drying and stored in anaerobic conditions. Meanwhile, nZVI was also prepared by the above procedure but without adding biochar.
Kinetics experiments
Batch experiments were conducted to investigate removal kinetics of Se(VI) by nZVI and BC-nZVI in a wild-mouth glass reactor open to the air at 20 ± 1.0 °C controlled by thermostatic bath. 0.5 L solution containing Se(VI) was completely mixed by digital display electric blender at 300 rpm. The solution pH value was buffered by 0.1 M NaAc and HAc at pH 4.0 and 5.0, the pH value of 6.0 was buffered by 0.1 M MES. And a certain amount of nZVI was quickly added into solution to initial experiments. At fixed interval time, about 5 mL sample was quickly filtered with 0.22 μm membrane samples and placed in the PTFE tubule which contained 1 drop of 65% concentrated nitric acid, and the final pH of all samples were below 2.0. All kinetics experiments were run in duplicates. The solid sample after reaction were collected, washed with DI water and freeze dried for further analysis.
Biological toxicity experiments
The quantity and morphological changes of E. coli were observed after culture with BC-nZVI or nZVI. The supernatant of the treated culture solution was collected by centrifugation at different time intervals. Variation of protein and superoxide dismutase (SOD) in cells were monitored to study effects of BC-nZVI or nZVI on the cell activity of E. coli.
Chemical analysis
A laboratory Shanghai Leici pHS-3C pH meter (Shanghai, China) was used to monitor pH value. The morphological analysis of nZVI, biochar, and BC-nZVI samples were performed by a JEOL JEM-1011 transmission electron microscopy (TEM) (Tokyo, Japan), a FEI Quanta 600 scanning electron microscopy (SEM) (Eindhoven, the Netherlands), and a Carl Zeiss SIGMA 300 SEM (Oberkochen, Germany), respectively. The morphological analysis of E. Coli cells were performed by a JEOL JEM-1011 transmission electron microscopy (TEM) (Tokyo, Japan). The crystal morphology of nZVI samples were analyzed by Rigaku DXR-8000 X-ray diffraction (Tokyo, Japan). Concentrations of Fe(II) were measured at 510 nm by the Shimadzu UV-visible 2600 spectrophotometer (Kyoto, Japan). Standards containing known concentrations of ferrous ion were used as reference for each set of tests. Concentrations of Se(VI) in solution were measured by the PerkinElmer Optima 5300 DV ICP-OES (Waltham, United States). Contents of protein and SOD in cells by Coomassie brilliant blue G250 and SOD kit.
Results and discussion
Characterization of nZVI and BC-nZVI
Fig. 1 shows the morphology images of synthesized nZVI (TEM), biochar (SEM), and BC-nZVI (Fe/C = 2/1, SEM) samples. Synthesized nZVI exhibited thick chain structures for the aggregation of various spherical nZVI particles. Biochar had a complex net structure with pores, channels, and fibrous form which might easily embed the nZVI. SEM-EDS elemental mapping results of C (red), Fe (orange) and O (green) of BC-nZVI are illustrated in Fig. S2,† and it clearly showed the nZVI was uniformly embedded on the biochar. BET specific surface area (SSA) was an important parameter of characterizing nZVI reactivity. The SSA of the synthesized nZVI reached 160 m2 g−1, but the SSA of BC-nZVI decreased to 108 m2 g−1. Generally, nZVI with a lager SSA showed a higher reactivity towards contaminants. Thus the addition of biochar could reduce the reactivity of nZVI. The XRD patterns of unreacted nZVI and BC-nZVI are both represented in Fig. 2. The synthesized nZVI was mainly in its Fe0 state (PDF06-0696). Meanwhile the diffraction peak of Fe2O3 (PDF39-1346) also was detected in nZVI which indicated that part of nZVI might be oxidised during the synthesis of nZVI. The synthesized BC-nZVI could form lepidocrocite (PDF08-0098), but no iron oxide was detected in BC-nZVI samples which indicated that the addition of biochar changed the iron oxide type on nZVI surface.
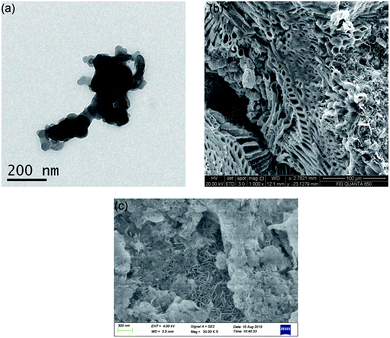 |
| Fig. 1 Morphology images of nZVI (a), biochar (b), and BC-nZVI (Fe/C = 2/1) (c). | |
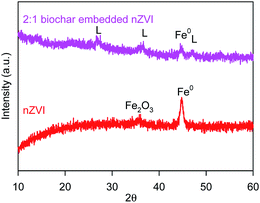 |
| Fig. 2 XRD patterns of nZVI and BC-nZVI (Fe/C of 2/1) before reaction. | |
Effects of different ratio of Fe/C on Se(VI) removal by BC-nZVI
In order to optimal the Fe/C of BC-nZVI materials for Se(VI) removal, effects of BC-nZVI with different Fe/C of 1/1, 2/1, 7/1 and nZVI on Se(VI) removal were compared experimentally. As shown in Fig. 3a, few Se(VI) was removed within 120 min by 1.0 g L−1 biochar alone. Generally biochar as carbon-based materials might absorb contaminants, but Se(VI) was hardly to be absorbed which indicated that Se(VI) showed a strong resistance to biochar. Nevertheless, Se(VI) was easily to be removed by nZVI, and the removal rate of Se(VI) was more than 95.4% in 30 min. Effects of three BC-nZVI samples on Se(VI) removal also were investigated. With the increase of Fe/C from 1/1 to 2/1, the removal rate of Se(VI) at 30 min increased from 48.8% to 95.9%. But the removal rate of Se(VI) at 30 min decreased to 59.5% when Fe/C reached to 7/1. On the one hand, the participation of nZVI in biochar materials could extensively enhance Se(VI) removal. On the other hand, the reactivity of BC-nZVI towards Se(VI) might be lower than pure nZVI. But the removal rate of Se(VI) by BC-nZVI with the optimal Fe/C of 2/1 at 30 min was similar to that by pure nZVI. So the replacement of part nZVI by biochar might enhance the reactivity of nZVI towards Se(VI). Generally, the release rate of Fe(II) represented the real reactivity of nZVI in water. Thus release kinetics of Fe(II) during Se(VI) removal are also monitored and shown in Fig. 3b. Fe(II) was quickly released with the degradation of Se(VI), and the release rate of Fe(II) during Se(VI) removal by various materials was consistent with the removal rate of Se(VI) which indicated that the removal of Se(VI) was ascribe to the corrosion of nZVI. Of three BC-nZVI, the BC-nZVI with the optimal Fe/C of 2/1 exhibited the highest release rate of Fe(II). Thus, BC-nZVI with Fe/C of 2/1 did not limit the reactivity towards Se(VI) but decreased the release of Fe(II) which reduced the discharge of residual sludge in engineering. The XRD patterns of nZVI and BC-nZVI (Fe/C of 2/1) samples after Se(VI) removal are both represented in Fig. 4. The results of XRD indicated the generation of lepidocrocite (γ-FeOOH) (PDF08-0098) after 120 min reaction with Se(VI). Other studies29,30 also identified that lepidocrocite was the first corrosion product in aerobic water. Besides, iron corrosion products of BC-nZVI after the reaction with Se(VI) were mainly lepidocrocite. Thus, the modification of nZVI by biochar did not change the corrosion process of BC-nZVI during Se(VI) removal.
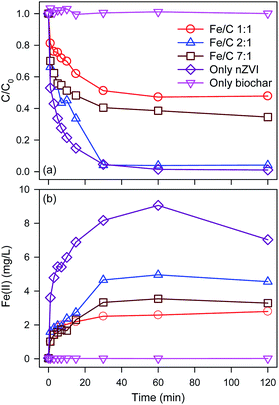 |
| Fig. 3 Kinetics of Se(VI) removal (a) and Fe(II) release during Se(VI) removal (b) by biochar, BC-nZVI, and nZVI with different Fe/C. Experiment conditions: [Se(IV)]0 = 3.0 mg L−1, [biochar] = [BC-nZVI] = [nZVI] = 1.0 g L−1, and pH = 5.0. | |
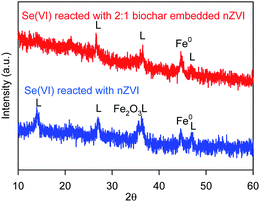 |
| Fig. 4 XRD patterns of nZVI and BC-nZVI (Fe/C of 2/1) after reaction. | |
Effects of key factors
BC-nZVI with the optimal Fe/C of 2/1 was selected to study effects of different factors on Se(VI) removal, and optimal experimental conditions were obtained. As shown in Fig. 5a, similar Se(VI) removal rate (at 30 min) are found at pH 4.0 (98.2%) and 5.0 (95.9%), but Se(VI) removal rate (at 30 min) at pH 6.0 was only 25.8%. Reactions between Se(VI) and nZVI needed the participation of H+, and acidic solution could facilitate Se(VI) removal.31 Meanwhile, low pH could also clean iron oxide layer on nZVI which generated during reactions.32 Solution pH increased for the consumption of H+ as the reaction progress, and the removal rate of Se(VI) might be inhibited. Effects of pH on the release of Fe(II) during Se(VI) removal are monitored in Fig. 5b. With pH increased from 4.0 to 6.0, the released rate of Fe(II) deceased. Curiously, the release rate of Fe(II) at pH 4.0 was much higher than that at pH 5.0, however there are no difference of Se(VI) removal rate at pH values between 4.0 and 5.0. The excessive release of Fe(II) at pH 4.0 did not promote the removal of Se(VI). The acid solution competed more reactivity sites of nZVI with Se(VI) during Se(VI) removal to generate more Fe(II). So pH 5.0 was selected as the solution pH for study effects of other factors on Se(VI) removal to obtain higher Se(VI) removal rate and decrease the generation of residual iron sludge.
 |
| Fig. 5 Effects of pH, BC-nZVI loading, initial Se(VI) concentration and Fe(II) release during Se(VI) removal by BC-nZVI. Experiment conditions: [Se(IV)]0 = 3.0 mg L−1 (a–d), [biochar] = [BC-nZVI] = [nZVI] = 1.0 g L−1 (a, b, e, and f), and pH = 5.0 (c–f). | |
Fig. 5c shows effects of BC-nZVI loading on the removal of Se(VI). With the increase of BC-nZVI loading from 0.5 g L−1 to 1 g L−1, the removal rate of Se(VI) at 30 min significantly increased from 25.5% to 95.9%. Generally, the increase of BC-nZVI could provide more surface active sites, which leaded to the promotion of Se(VI) removal rate. The increasing BC-nZVI loading from 1 g L−1 to 2 g L−1 slightly increased the removal rate of Se(VI) which indicated that surface active sites of 1 g L−1 BC-nZVI were enough to remove Se(VI), and the continuous increase of BC-nZVI could not improve the removal rate of Se(VI).
As shown in Fig. 5d, the increase of BC-nZVI loading from 0.5 g L−1 to 1 g L−1 can accelerate the release of Fe(II). But the release of Fe(II) during Se(VI) removal by 1 g L−1 BC-nZVI was similar to that by 2 g L−1 BC-nZVI, which indicated that the corrosion of nZVI was not promoted with the increase of BC-nZVI loading.
Effects of initial Se(VI) concentration on the removal of Se(VI) are illustrated in Fig. 5e. With the decrease of Se(VI) concentration, the removal rate of Se(VI) increased. When the initial Se(VI) concentration was lower than 3 mg L−1, Se(VI) was completely removed by BC-nZVI in 30 min. But the initial Se(VI) concentration reached to 6 mg L−1, the removal rate of Se(VI) at 30 min decreased to 25.9%. Reactive sites of BC-nZVI were limited, and the increasing Se(VI) decreased the removal rate of Se(VI). Meanwhile, the remarkable decline of Se(VI) removal rate indicated that high Se(VI) concentration might inhibited the reactivity of BC-nZVI. Fig. 5f exhibits release kinetics of Fe(II) during Se(VI) removal. The release rate of Fe(II) increased with the decrease of initial Se(VI) concentration which indicated that the corrosion of nZVI was in accordance with Se(VI) removal.
Biological toxicity of BC-nZVI to E. coli
Researchers reported that nZVI could exhibit the bactericidal effect on E. coli.23 Fig. 6 shows the TEM images of E. coli in the absence and presence of nZVI, and nZVI could rupture or deform E. coli cells. Meanwhile, BC-nZVI contain nZVI which could also destroy E. coli cells. To study the biological adverse effect of BC-nZVI, E. coli was selected as the model microorganism to investigate the potential toxicity of BC-nZVI. Comparative experiments in the growth of E. coli cells with nZVI and BC-nZVI at different loadings were conducted. As shown in Fig. 7a, the amount of E. coli cells gradually decreased with the increasing loading of nZVI and BC-nZVI in the range of 0–6 g L−1. But the amount of E. coli cells treated by nZVI was lower than that treated by BC-nZVI at the same loading. To further study the toxicity of BC-nZVI, the SOD enzyme activity of E. coli cells was measured. Generally, the SOD activity represented the degree of intracellular lipid peroxidation and the ability to scavenge reactive oxygen species. As shown in Fig. 7b, the SOD activity of E. coli cells decreases with the increasing nZVI or BC-nZVI loading which is similar to the variation of E. coli amount. However, the SOD activity of E. coli cells with nZVI was much lower than that with BC-nZVI. Thus some E. coli cells in the presence of nZVI were survive but the activity of E. coli cells was seriously damaged. Meanwhile, Fig. 7c illustrates the content of the protein in E. coli culture medium. The content of the protein was quickly increased after the treatment of nZVI or BC-nZVI, which indicated that cells of E. coli were destroyed and intracellular organic matter were dissolved. And E. coli culture medium with nZVI could release more protein which suggested that nZVI possessed a higher ability to break up E. coli cells than BC-nZVI. So results of various biological indicator demonstrated that the biochar modification reduced destructive effects of nZVI on E. coli cells.
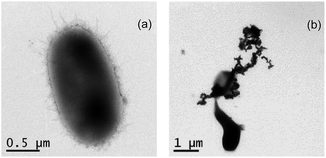 |
| Fig. 6 TEM images of original E. coli (a) and E. coli treated by nZVI (b). | |
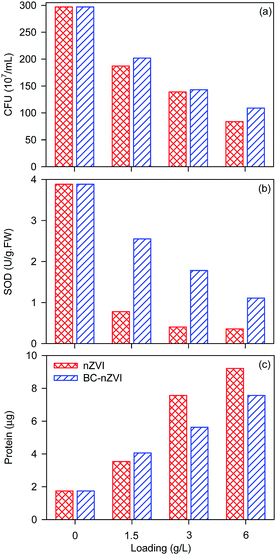 |
| Fig. 7 Effects of nZVI and BC-nZVI with different loading on the calculated CFU of E. coli (a), the SOD activity of E. coli (b), and the protein generation during the growth of E. coli (c). | |
Conclusions
BC-nZVI with different Fe/C and pure nZVI were synthesized to remove aquatic Se(VI) removal. The SEM-EDX elemental mapping analysis showed that nZVI could be uniformly embedded on the carbon-thermal synthetic biochar. The addition of biochar decreased the SSA and formed lepidocrocite in BC-nZVI. BC-nZVI with Fe/C of 2/1 showed optimal removal rate by comparing other samples with different Fe/C. And pure biochar could hardly absorb Se(VI), so the removal of Se(VI) by BC-nZVI was contributed to nZVI. Effects of solution pH, initial concentration of Se(VI), and BC-nZVI (Fe/C of 2/1) loading on Se(VI) removal were studied. And the optimal parameters were pH 4.0, 2 g L−1 BC-nZVI, and 1.5 mg L−1 Se(VI). E. coli were employed to study the biological toxicity of BC-nZVI and pure nZVI. Quantitative results including calculated amount, SOD activity, and protein content of E. coli cells indicated that nZVI and BC-nZVI with different loading both inhibited the growth of E. coli. And the inhibition efficiency of E. coli cells growth increased with the increase of the loading of nZVI and BC-nZVI. But the amount of E. coli cells treated by nZVI was lower than that treated by BC-nZVI at the same loading. Moreover, the SOD activity of E. coli cells with nZVI was much lower than that with BC-nZVI. Some E. coli cells in the presence of pure nZVI might be survived but the activity of E. coli cells was seriously damaged. Meanwhile, E. coli culture solution with nZVI released more protein than that with BC-nZVI, which indicated that more intracellular organic matter of E. coli cells were dissolved in the presence of nZVI. So modified nZVI by biochar was less harmful to E. coli cells than nZVI.
Conflicts of interest
There are no conflicts to declare.
Acknowledgements
The authors gratefully acknowledge the financial support of the National Natural Science Foundation of China (Grant No. 41807468), Zhejiang Provincial Natural Science Foundation of China (Grant No. LY18E080018), Shaoxing Public Welfare Project (Grant No. 2017B70042), and National innovation and entrepreneurship training program for college students (Grant No. 201810349008) and University students' science and technology innovation program of Zhejiang Province (Young Talents Program) (Grant No. 2018R432004).
Notes and references
- H. H. Zhou, T. Wang, Q. Li and D. D. Li, Biol. Trace Elem. Res., 2018, 186, 98–105 CrossRef CAS PubMed.
- L. H. Foster and S. Sumar, Crit. Rev. Food Sci. Nutr., 1997, 37, 211–228 CrossRef CAS PubMed.
- M. Sahebari, Z. Rezaieyazdi and M. Khodashahi, Curr. Rheumatol. Rev., 2019, 15, 123–134 CrossRef CAS PubMed.
- M. P. Rayman, The Lancet, 2000, 356, 233–241 CrossRef CAS.
- B. D. Gibson, D. W. Blowes, M. B. J. Lindsay and C. J. Ptacek, J. Hazard. Mater., 2012, 241–242, 92–100 CrossRef CAS PubMed.
- WHO, Agents Classified by the IARC Monographs, vol. 1–120, http://monographs.iarc.fr/ENG/Classification/latest_classif.php Search PubMed.
- D. Pontarolo, T. Sandy, N. Keller, M. Gearhart, V. Patel and J. Jimenez, Proceedings of the Water Environment Federation, 2017, 2017, 4118–4132 CrossRef.
- J. T. Olegario, N. Yee, M. Miller, J. Sczepaniak and B. Manning, J. Nanopart. Res., 2009, 12, 2057–2068 CrossRef.
- N. Bleiman and Y. G. Mishael, J. Hazard. Mater., 2010, 183, 590–595 CrossRef CAS PubMed.
- R. J. Shamberger, Sci. Total Environ., 1981, 17, 59–74 CrossRef CAS PubMed.
- P. Devi, R. Jain, A. Thakur, M. Kumar, N. K. Labhsetwar, M. Nayak and P. Kumar, Trends Anal. Chem., 2017, 95, 69–85 CrossRef CAS.
- S. Santos, G. Ungureanu, R. Boaventura and C. Botelho, Sci. Total Environ., 2015, 521–522, 246–260 CrossRef CAS PubMed.
- European Economic Community, Council Directive 98/83/EC Relating to the Water Quality of Water Intended for Human Consumption, Off. J. Eur. Commun., 1998, L330, 32–54 Search PubMed.
- WHO, Guidelines for Drinking-water Quality, World Health Organization, Geneva, 4th edn, 2011 Search PubMed.
- USEPA, National Primary Drinking Water Regulations, List of Contaminants and their (MCLs), EPA 816-F-09-0004, United states Environmental Protection Agency, Washington, DC, 2009 Search PubMed.
- Y. Zhang, C. Amrhein, A. Chang and W. T. Frankenberger, Sci. Total Environ., 2008, 407, 89–96 CrossRef CAS PubMed.
- R. López de Arroyabe Loyo, S. I. Nikitenko, A. C. Scheinost and M. Simonoff, Environ. Sci. Technol., 2008, 42, 2451–2456 CrossRef PubMed.
- L. P. Liang, W. J. Yang, X. H. Guan, J. L. Li, Z. J. Xu, J. Wu, Y. Y. Huang and X. Z. Zhang, Water Res., 2013, 47, 5846–5855 CrossRef CAS PubMed.
- L. P. Liang, W. Sun, X. H. Guan, Y. Y. Huang, W. Y. Choi, H. L. Bao, L. N. Li and Z. Jiang, Water Res., 2014, 49, 371–380 CrossRef CAS PubMed.
- C. Wang and W. Zhang, Environ. Sci. Technol., 1997, 31, 2154–2156 CrossRef CAS.
- S. J. Yu, L. Yin, H. W. Pang, Y. H. Wu, X. X. Wang, P. Zhang, B. W. Hu, Z. S. Chen and X. K. Wang, Chem. Eng. J., 2018, 352, 360–370 CrossRef CAS.
- S. J. Yu, X. X. Wang, Y. F. Liu, Z. S. Chen, Y. H. Wu, Y. Liu, H. W. Pang, G. Song, J. R. Chen and X. K. Wang, Chem. Eng. J., 2019, 365, 51–59 CrossRef CAS.
- C. Lee, J. Y. Kim, W. I. Lee, K. L. Nelson, J. Yoon and D. L. Sedlak, Environ. Sci. Technol., 2008, 42, 4927–4933 CrossRef CAS PubMed.
- X. Wang, H. L. Dong, Q. Zeng, Q. Y. Xia, L. M. Zhang and Z. Q. Zhou, Environ. Sci. Technol., 2017, 51, 7639–7647 CrossRef CAS PubMed.
- Y. S. El-Temsah and E. J. Joner, Chemosphere, 2012, 89, 76–82 CrossRef CAS PubMed.
- L. Zhou, W. Zhuang, X. Wang, K. Yu, S. Yang and S. Xia, Water Res., 2017, 111, 140–146 CrossRef CAS PubMed.
- S. J. Yu, Y. Liu, Y. J. Ai, X. X. Wang, R. Zhang, Z. S. Chen, Z. Chen, G. X. Zhao and X. K. Wang, Environ. Pollut., 2018, 242, 1–11 CrossRef CAS PubMed.
- H. W. Pang, Z. F. Diao, X. X. Wang, Y. Ma, S. J. Yu, H. T. Zhu, Z. S. Chen, B. W. Hu, J. R. Chen and X. K. Wang, Chem. Eng. J., 2019, 366, 368–377 CrossRef CAS.
- Y. H. Huang and T. C. Zhang, Water Res., 2005, 39, 1751–1760 CrossRef CAS PubMed.
- L. J. Xu and J. K. Wang, J. Hazard. Mater., 2011, 186, 256–264 CrossRef CAS PubMed.
- Y. Xie, H. Dong, G. Zeng, L. Zhang, Y. Cheng, K. Hou, Z. Jiang, C. Zhang and J. Deng, J. Hazard. Mater., 2017, 338, 306–312 CrossRef CAS PubMed.
- X. H. Guan, Y. K. Sun, H. J. Qin, J. X. Li, I. M. C. Lo, D. He and H. R. Dong, Water Res., 2015, 75, 224–248 CrossRef CAS PubMed.
Footnote |
† Electronic supplementary information (ESI) available. See DOI: 10.1039/c9ra04535j |
|
This journal is © The Royal Society of Chemistry 2019 |
Click here to see how this site uses Cookies. View our privacy policy here.