DOI:
10.1039/C9RA04604F
(Paper)
RSC Adv., 2019,
9, 30768-30772
A multicomponent, facile and catalyst-free microwave-assisted protocol for the synthesis of pyrazolo-[3,4-b]-quinolines under green conditions†
Received
19th June 2019
, Accepted 22nd September 2019
First published on 30th September 2019
Abstract
A facile, swift and ecofriendly microwave-assisted multi-component/one-pot protocol is designed for the synthesis of novel pyrazolo-[3,4-b]-quinolines at ambient temperature in aqueous ethanol as a reaction medium. The 18 novel pyrazolo-[3,4-b]-quinoline derivatives were synthesized by fusion of chosen aryl aldehyde, dimedone and 5-amino-3-methyl-1-phenylpyrazole in excellent yields (91–98%). All the molecular structures were confirmed by 1H-NMR, 15N-NMR, 13C-NMR, and HRMS data analysis. Operational simplicity, easy handling, one-step simple workup procedure, mild reaction conditions, short reaction time (≤10 min), high selectivity and no by-product formation are the striking features of the protocol.
Introduction
The advent of green alternate multicomponent reactions (MCRs) has provided latitude for researchers to explore new reactions, which can create vital pharmaceutical and biological skeletons in an eco-friendly way.1,2 In such reactions, multiple-bonds are formed between three or more reactants in a single step, with no need to isolate any intermediate or change the solvent.3 MCRs are well-known tools for easy carbon–carbon bond creation in synthetic chemistry,4 and offer an easy workup procedure, higher selectivity and improved yields due to generation of C–C and C–heteroatom bonds in a single step.2–4 MCRs offer notable benefits including simple handling, construction of highly complex molecules in a single step, formation of products in higher yields and minimizing the need for purification.5,6 Furthermore, atom economy, less solvent consumption and reduced waste generation are other key advantages.7–15
Microwave (MW) irradiation as a tool in organic synthesis has become important in the development of heterocyclic molecules and drug discovery processes, due to its ability to deliver improved yields in short reaction times.16,17 MW irradiation enhances the reaction rates and facilitates the reaction in mild conditions.18 The reaction rates get faster via a development of oscillation excitation, and mass transference in a microwave-environment.19 Further, MW irradiation displays many advantages like minimal energy consumption, minimal waste production and atom economy.20,21 As MW irradiation directly couples the molecules in the reaction mixture, the choice of the solvent is crucial to achieve optimum reaction conditions.22 Both polar (protic and aprotic) solvent are known to be effective in MW organic synthesis. Unlike non-polar solvents, such solvents can couple with a MW irradiation, thus generating more heat. Thus, MW irradiation provides a facile and benign means for synthesis of novel organic moieties, using one-pot approach.
Pyrazolo-[3,4-b]-quinolines are one of the most significant biological skeletons with wider applications in pharmaceutical and medicinal fields and in heterocyclic synthesis as intermediates.23,24 These moieties are fused bioactive molecules with antimicrobial,25 translocator protein ligands,26 anticancer,27 pulmonary hypertension,28 cytotoxicity,29 antiplatelet,30 anti-chagasic,31 antimalarial,32 antiviral33 and anti-inflammatory34 activities. Additionally, these molecules have extensively explored as Alzheimer's disease35 and anti-influenza virus36 treatment agents. Due to their growing importance, several articles have been reported in the recent past, for the preparation of pyrazolo-[3,4-b]-quinoline scaffolds using different homogeneous and heterogeneous materials such as FeNi3-ILs,37 PEG-OSO3H,38L-proline,39 InCl3 (ref. 40) and PEG-400 (ref. 41) as catalysts, to name few. However, the aforementioned procedures have limitations and they suffer from drawbacks, like prolonged reaction times, harsh reaction conditions, catalyst separation challenges, tedious workup, waste generation, toxic solvents, high reaction temperatures and low product yields. Therefore, there is demand for newer methods that could surmount the above challenges. Here, we report an efficient catalyst-free one-pot protocol for the preparation of pyrazolo-[3,4-b]-quinolines under MW irradiation in aqueous EtOH at room temperature, reacting the chosen aryl aldehyde, dimedone and 5-amino-3-methyl-1-phenylpyrazole. There have been no earlier reports for the preparation of novel pyrazolo-[3,4-b]-quinoline derivatives under MWI.
Experimental procedure
General process for preparation of pyrazolo-[3,4-b]-quinoline derivatives
A mixture of aromatic aldehyde (1.0 mmol), dimedone (1.0 mmol) and 5-amino-3-methyl-1-phenylpyrazole (1.0 mmol) were placed in a microwave vessel containing 5.0 mL EtOH
:
H2O (1
:
1 v/v) solvent (Scheme 1). The reaction vessel was MW assisted by using microwave irradiation power (at 150 W) for 5 minutes at 50 °C. The reaction progress was checked by thin layer chromatography (TLC) analysis (eluent n-hexane: ethyl acetate 3
:
2 v/v). After completion of reaction, the reaction mixture, washed with cold water to afford the crude product. Then it was purified by recrystallization from ethanol to obtain target molecules. Structures of all products were confirmed based on the spectra analysis, 1H-NMR, 15N-NMR, 13C-NMR and HRMS data. The instrumental details and spectral characterization data is incorporated in the ESI (SI-1†).
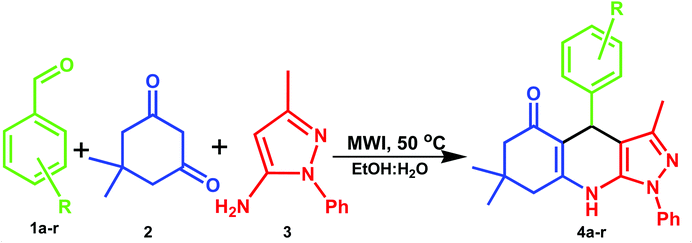 |
| Scheme 1 Three-component green synthetic route for pyrazolo-[3,4-b]-quinoline derivatives. | |
Results and discussion
The pilot reaction was investigated using aromatic aldehyde (1 mmol), dimedone (1 mmol) and 5-amino-3-methyl-1-phenylpyrazole (1 mmol) under microwave irradiation and conventional stirring conditions to optimize the reaction conditions for the preparation of pyrazolo-[3,4-b]-quinoline derivatives. First, the reaction accomplishment was assessed by engaging a series of solvents (polar and/or non-polar) as well as under solvent-free conditions to explore the target yields in shorter reaction times. Initially, the model reaction was conducted in the absence of any catalyst or solvent at room temperature (R.T). However, the reaction failed to give any product even after 6 h, under conventional or MWI conditions. Under both the conditions, no desired product detected, when the reaction was studied with non-polar solvents (hexane, toluene and DCM) for 6 h. In polar aprotic solvents (DMF, DMSO and THF), only a trace amount of yield was obtained with both the methods. Under polar protic solvents (H2O, MeOH and EtOH), only moderate yields occurred under silent method, even after 2 h, while good yields were noticed under MWI within 1 h. When, the reaction was carried in mixed solvent system (EtOH
:
H2O, 1
:
1 v/v), excellent yield (98%) was afforded in very short reaction time (5 min) under MWI (Table 1). As the mixture of polar solvents with higher dielectric constants could couple well with the microwave irradiation, it generates rapid heating.2,17 Accepting an equal molar aqueous ethanol as ideal solvent for the reaction, the influence of catalyst on the product yield and reduce reaction time was further examined. The reaction with various catalysts like NaHCO3, K2CO3, pyridine, and trimethylamine (inorganic or organic) in aqueous ethanol system afforded moderate to good yields, under both the conditions. When the title reaction was investigated with L-proline, an ionic liquid catalyst, both under silent and MWI conditions, no significant improvement was observed on the yield or reaction time. Hence, the reaction was optimized using benign and cost-effective aqueous ethanol as reaction medium as opposed to the other solvents and catalysts, under catalyst-free conditions.
Table 1 Optimization of the model reaction under varied MW irradiation and silent conditionsa
Entry |
Catalyst |
Condition |
Conventional |
MWI |
Time (h) |
Yield (%) |
Time (h) |
Yieldb (%) |
All products were characterized by 1H-NMR, 15N NMR, 13C-NMR and HRMS spectral analysis.
Isolated yields.
— no reaction.
|
1 |
Catalyst free |
R.T |
6.0 |
—c |
6.0 |
—c |
2 |
Solvent free |
R.T |
6.0 |
—c |
6.0 |
—c |
3 |
Hexane |
R.T |
6.0 |
—c |
6.0 |
—c |
4 |
Toluene |
R.T |
6.0 |
—c |
6.0 |
—c |
5 |
DCM |
R.T |
6.0 |
—c |
6.0 |
—c |
6 |
DMF |
R.T |
6.0 |
Trace |
6.0 |
Trace |
7 |
DMSO |
R.T |
6.0 |
Trace |
6.0 |
Trace |
8 |
THF |
R.T |
6.0 |
Trace |
6.0 |
Trace |
9 |
H2O |
R.T |
2.0 |
71 |
1.0 |
83 |
10 |
MeOH |
R.T |
2.0 |
64 |
1.0 |
72 |
11 |
EtOH |
R.T |
2.0 |
74 |
1.0 |
90 |
12
|
EtOH : H2O |
R.T
|
1.5
|
88
|
0.1
|
98
|
13 |
NaHCO3 |
R.T |
1.5 |
56 |
0.5 |
89 |
14 |
K2CO3 |
R.T |
1.0 |
61 |
0.5 |
90 |
15 |
Pyridine |
R.T |
1.5 |
51 |
0.75 |
84 |
16 |
TEA |
R.T |
1.0 |
49 |
0.75 |
81 |
17 |
L-proline |
R.T |
1.5 |
54 |
0.50 |
59 |
Further, the effect of reaction temperature on the product yield in aqueous EtOH was investigated under MW and catalyst-free conditions. When the temperature was decreased from 50 to 30 °C, the yield reduced from 98 to 50%, and interestingly the increase in temperature from 50 °C to 100 °C, also registered decrease in yield (from 98 to 93%), with marginal change in reaction time. Therefore, the reaction in aqueous EtOH via MW irradiation at 50 °C proved optimum condition in terms of the yield and reaction time (Table 2).
Table 2 Optimization of the temperature conversion of the model reactiona
Entry |
Temperature (°C) |
Time (min) |
Yield (%) |
Reaction conditions: aromatic aldehyde one equivalent, dimedone one equivalent and 5-amino-3-methyl-1-phenylpyrazole one equivalent in in EtOH : H2O aqueous solvent.
|
1 |
30 |
60 |
50 |
2 |
40 |
30 |
71 |
3
|
50
|
5
|
98
|
4 |
60 |
5 |
98 |
5 |
70 |
5 |
97 |
6 |
80 |
5 |
96 |
7 |
90 |
5 |
95 |
8 |
100 |
5 |
93 |
Using the ideal reaction conditions, the strength of this protocol was assessed for the preparation of pyrazolo-[3,4-b]-quinoline derivatives from varied aldehydes. The catalyst-free MW procedure proved good for one-pot synthesis of eleven pyrazolo-[3,4-b]-quinoline derivatives offering excellent yields in all the reactions. All the substrates, containing both electron-donating and electron-withdrawing groups (irrespective of ortho, meta and para positions) on the phenyl ring, participated in the reaction giving excellent yields (Table 3). Aliphatic aldehydes such as propionaldehyde, butyraldehyde, phenylacetaldehyde and cyclohexanecarboxaldehyde failed to provide product yields under the chosen reaction conditions. All the compounds were characterized and confirmed by 1H-NMR, 15N-NMR, 13C-NMR and HRMS.
Table 3 Synthesis of pyrazolo-[3,4-b]-quinoline derivatives in ethanol under MW irradiationa
Entry |
R |
Product |
Yield* (%) |
Mp (°C) |
Reaction conditions: arylaldehyde (1 mmol) dimedone (1 mmol) and 5-amino-3-methyl-1-phenylpyrazole one (1 mmol) in ethanol solvent (5 mL) were stirred at room temperature. R = substituted benzaldehydes, * = isolated yields.
|
1 |
2,3-OH |
4a
|
94 |
119–121 |
2 |
2-CF3 |
4b
|
93 |
136–137 |
3 |
2-F |
4c
|
94 |
183–184 |
4 |
2-Me |
4d
|
98 |
200–201 |
5 |
3,4,5-OMe |
4
|
94 |
197–198 |
6 |
3-OH, 4-OMe |
4f
|
92 |
239–241 |
7 |
3-OMe |
4g
|
96 |
176–178 |
8 |
4-Et |
4h
|
95 |
246–248 |
9 |
4-OMe |
4i
|
94 |
221–223 |
10 |
Benzaldehyde |
4j
|
91 |
190–192 |
11 |
3-Pyridine |
4k
|
94 |
239–241 |
12 |
3,4-OH |
4l
|
95 |
216–217 |
13 |
3-F |
4m
|
93 |
204–205 |
14 |
4-F |
4n
|
94 |
221–223 |
15 |
4-Br |
4o
|
92 |
190–192 |
16 |
4-Cl |
4p
|
96 |
175–176 |
17 |
3,4-OMe |
4q
|
96 |
235–237 |
18 |
2-Thio |
4r
|
94 |
218–219 |
A plausible mechanism for the synthetic reaction is illustrated in Scheme 2. It is proposed that initially, Knoevenagel condensation reaction occurs between an aryl aldehyde (2) and dimedone (1), resulting in the adduct A, which is dehydrated to give intermediate B. Michael type addition reaction follows between 5-amino-3-methyl-1-phenylpyrazole (3) and intermediate B. The resulting intermediate, C rearranges to a stable adduct D, which is further cyclized and dehydrated to yield the product (4).
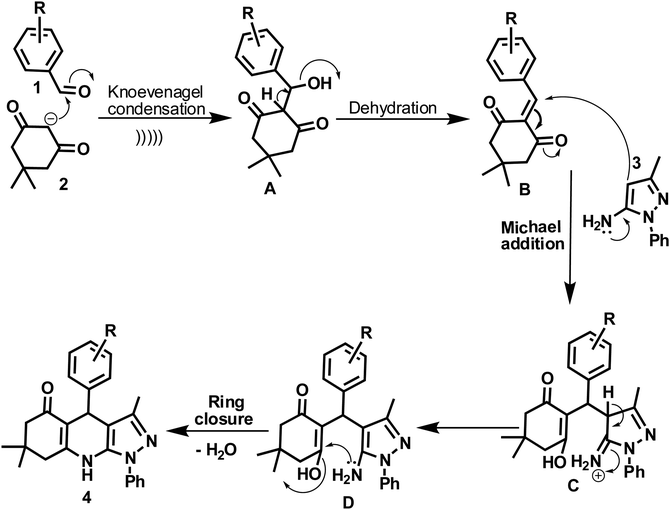 |
| Scheme 2 Plausible reaction mechanism for the formation of pyrazolo-[3,4-b]-quinoline derivatives. | |
Conclusion
In conclusion, we report a facile, convenient and green method for synthesis of eleven novel pyrazolo-[3,4-b]-quinolines under catalyst-free and aqueous solvent conditions via MW irradiation. The significant features of this present protocol being operational simplicity, simple workup procedure, mild conditions, short reaction times, high yields with high purity, and no column chromatography used for compound purification. To the best of our knowledge, this is the first report where MW irradiated has been used for the synthesis of pyrazolo-[3,4-b]-quinoline derivatives under catalyst-free and aqueous EtOH solvent condition. The present method will offer an attractive synthetic protocol for the preparation of structurally varied drug-like compounds for the pharmaceutical and drug design discovery fields.
Conflicts of interest
There are no conflicts to declare.
Acknowledgements
Authors sincerely thank the School of Chemistry and Physics for the material support and facilities to conduct this work.
References
- S. Maddila, K. K. Gangu, S. N. Maddila and S. B. Jonnalagadda, Curr. Org. Synth., 2017, 14, 634 CrossRef CAS.
- S. N. Maddila, S. Maddila, M. Khumalo, S. V. H. S. Bhaskaruni and B. J. Sreekantha, J. Mol. Struct., 2019, 1185, 357–360 CrossRef CAS.
- S. V. H. S. Bhaskaruni, K. K. Gangu, S. Maddila and S. B. Jonnalagadda, Chem. Rec., 2018, 18, 1–21 CrossRef.
- S. Maddila, K. K. Gangu, S. N. Maddila and S. B. Jonnalagadda, Mol. Diversity, 2017, 21, 247–255 CrossRef CAS.
- S. Shabalala, S. Maddila, W. Van Zyl and S. B. Jonnalagadda, Ind. Eng. Chem. Res., 2017, 56, 11372–11379 CrossRef CAS.
- K. K. Gangu, S. Maddila and S. B. Jonnalagadda, Ind. Eng. Chem. Res., 2017, 56, 2917–2924 CrossRef CAS.
- C. Ma, J.-Y. Zhou, Y.-Z. Zhang, Y. Jiao, G.-J. Mei and F. Shi, Chem. - Asian J., 2018, 13, 2549–2558 CrossRef CAS.
- X.-X. Sun, C. Li, Y.-Y. He, Z.-Q. Zhu, G.-J. Mei and F. Shi, Adv. Synth. Catal., 2017, 359, 2660–2670 CrossRef CAS.
- Y.-M. Wang, H.-H. Zhang, C. Li, T. Fan and F. Shi, Chem. Commun., 2016, 52, 1804–1807 RSC.
- X.-X. Sun, H.-H. Zhang, G.-H. Y.-Y. He and F. Shi, Chem. - Eur. J., 2016, 22, 17526–17532 CrossRef CAS.
- Q.-N. Zhu, Y.-C. Zhang, M.-M. Xu, X.-X. Sun, X. Yang and F. Shi, J. Org. Chem., 2016, 81, 7898–7907 CrossRef CAS.
- W. Dai, X.-L. Jiang, J.-Y. Tao and F. Shi, J. Org. Chem., 2016, 81, 185–192 CrossRef CAS.
- L. Wang, H.-H. Zhang, C. Li, T. Fan and F. Shi, J. Org. Chem., 2017, 82, 3605–3611 CrossRef CAS.
- W.-J. Hao, Q. Gao, B. Jiang, F. Liu, S.-L. Wang, S.-J. Tu and G. Li, J. Org. Chem., 2016, 81, 11276–11281 CrossRef CAS.
- T.-S. Zhang, Y.-J. Xiong, W.-J. Hao, X.-T. Zhu, S.-L. Wang, G. Li, S.-J. Tu and B. Jiang, J. Org. Chem., 2016, 81, 9350–9355 CrossRef CAS.
- H. Cho, F. Torok and B. Torok, Green Chem., 2014, 16, 3623–3634 RSC.
- P. Cristian, V. Chirosca, C. S. Heidenga and J. D. Hoeschele, Dalton Trans., 2015, 44, 3384–3392 RSC.
- A. Kokel, C. Schafer and B. Torok, Green Chem., 2017, 19, 3729–3751 RSC.
- A. Kulkarni and B. Torok, Green Chem., 2010, 12, 875–878 RSC.
- P. Prasanna, K. Balamurugan, S. Perumal and J. C. Menendez, Green Chem., 2011, 13, 2123–2129 RSC.
- A. Sharma, P. Appukkuttan and E. Van Eycken, Chem. Commun., 2012, 48, 1623–1637 RSC.
- A. Dastan, A. Kulkarni and B. Torok, Green Chem., 2012, 14, 17–37 RSC.
- V. Sharma, P. Bhatia, O. Alam, M. J. Naim, F. Nawaz, A. A. Sheikh and M. Jha, Bioorg. Chem., 2019, 89, 103007 CrossRef CAS.
- D. Raffa, B. Maggio, M. V. Raimondi, S. Cascioferro, F. Plescia, G. Cancemi and G. Daidone, Eur. J. Med. Chem., 2015, 97, 732–746 CrossRef CAS.
- S. T. Selvi, V. Nadaraj, S. Mohan, R. Sasi and M. Hema, Bioorg. Med. Chem., 2006, 14, 3896–3903 CrossRef CAS.
- A. Cappelli, G. Bini, S. Valenti, G. Giuliani, M. Paolino, M. Anzini, S. Vomero, G. Giorgi, A. Giordani, L. P. Stasi, F. Makovec, C. Ghelardini, L. Mannelli, A. Concas, P. Porcu and G. Biggio, J. Med. Chem., 2011, 54(20), 7165–7175 CrossRef CAS.
- C. Karthikeyan, R. Malla, C. R. Ashby, H. Amawi, K. L. Abbott, J. Moore, J. Chen, C. Balch, C. Lee, P. C. Flannery, P. Trivedi, J. S. Faridi, S. R. Pondugula and A. K. Tiwari, Cancer Lett., 2016, 376, 118–126 CrossRef CAS.
- L. Li, W. Zhang, F. Lin, X. Lu, W. Chen, X. Li, X. Zhou, R. Su, L. Wang, Z. Zheng and S. Li, Eur. J. Med. Chem., 2019, 173, 107–116 CrossRef CAS.
- C. Kurumurthy, B. Veeraswamy, P. S. Rao, G. S. Kumar, P. S. Rao, V. L. Reddy, J. Venkateswara Rao and B. Narsaiah, Bioorg. Med. Chem. Lett., 2014, 24, 746–749 CrossRef CAS.
- A. L. Lourenco, R. R. S. Salvador, L. A. Silva, M. S. Saito, J. F. R. Mello, L. M. Cabral, C. R. Rodrigues, M. A. F. Vera, A. M. T. de Souza, C. S. Craik, L. R. S. Dias, H. C. Castro and P. C. Sathler, Eur. J. Med. Chem., 2017, 135, 213–229 CrossRef CAS.
- L. R. S. Dias, M. B. Santos, S. de Albuquerque, H. C. Castro, A. M. T. de Souza, A. C. C. Freitas, M. A. V. DiVaio, L. M. Cabral and C. R. Rodrigues, Bioorg. Med. Chem. Lett., 2007, 211–219 CrossRef CAS.
- A. M. Hussin, F. A. Abu-Shanab and E. A. Ishak, Elements, 2000, 159, 55–68 Search PubMed.
- A. R. Azevedo, V. F. Ferreira, H. de Mello, L. R. Leao-Ferreira, A. V. Jabor, I. C. P. P. Frugulhetti, H. S. Pereira, N. Moussatche and A. M. R. Bernardino, Heterocycl. Commun., 2000, 8, 427–432 Search PubMed.
- L. W. Mohamed, M. A. Shaaban, A. F. Zaher and S. M. A. andA. M. Elsahar, Bioorg. Chem., 2019, 83, 47–54 CrossRef CAS.
- T. Umar, S. Shalini, Md K. Raza, S. Gusain, J. Kumar, P. Seth, M. Tiwari and N. Hoda, Eur. J. Med. Chem., 2019, 175, 2–19 CrossRef CAS PubMed.
- Z. LY, T. Liu, J. Yang, Y. Yang, C. Cai and S. Liu, ACS Comb. Sci., 2017, 19(7), 437–446 CrossRef.
- J. Safaei-Ghomi, R. Sadeghzadeh and H. Shahbazi-Alavi, RSC Adv., 2016, 6, 33676–33685 RSC.
- S. Paul and A. R. Das, Tetrahedron Lett., 2013, 54, 1149–1154 CrossRef CAS.
- D. Bhattacharjee, B. Kshiar and B. Myrboh, RSC Adv., 2016, 6, 95944–95950 RSC.
- J. M. Khurana, A. Chaudhary, B. Nand and A. Lumb, Tetrahedron Lett., 2012, 53, 3018–3022 CrossRef CAS.
- K. Karnakar, S. N. Murthy, K. Ramesh, G. Satish, J. B. Nanubolu and Y. Nageswar, Tetrahedron Lett., 2012, 53, 2897–2903 CrossRef CAS.
Footnote |
† Electronic supplementary information (ESI) available. See DOI: 10.1039/c9ra04604f |
|
This journal is © The Royal Society of Chemistry 2019 |
Click here to see how this site uses Cookies. View our privacy policy here.