DOI:
10.1039/C9RA04961D
(Paper)
RSC Adv., 2019,
9, 27294-27304
Synthesis and discovery of 18β-glycyrrhetinic acid derivatives inhibiting cancer stem cell properties in ovarian cancer cells†
Received
1st July 2019
, Accepted 26th August 2019
First published on 30th August 2019
Abstract
Despite advances in ovarian cancer treatment, the five-year overall survival rate is less than 30% with the presence of cancer stem cells (CSCs). To develop CSC-targeting therapy, a series of 18β-glycyrrhetinic acid (GA) derivatives containing cinnamamide moiety have been designed, synthesized, and screened for their antiproliferative activity in SKOV3 and OVCAR3 cells. Most of the compounds exhibited stronger antiproliferative activity than GA, and compound 7c was the most active one. Further biological studies showed that compound 7c could induce apoptosis and suppress migration. In addition, compound 7c could not only observably decrease the colony formation and sphere formation ability, but also significantly reduce the CD44+, CD133+, and ALDH+ subpopulation in SKOV3 and OVCAR3 cells. In conclusion, these results indicate that compound 7c is a promising anti-CSC agent for further anti-ovarian cancer studies.
1. Introduction
Ovarian cancer is a highly heterogeneous neoplasm in histology, biological properties, and one of the most deadly malignant tumours of the female reproductive organs.1 Over 70% of ovarian cancer patients would experience recurrence, and the five-year overall survival rate is less than 30%.2 The current standard treatment of advanced ovarian cancer is cytoreductive surgery followed the chemotherapy treatment of a combination of cisplatin or carboplatin with paclitaxel. Standard treatment can eliminate the size of primary tumours and the bulk of cancer cells, but complete tumour eradication is not always achieved due to the presence of cancer stem cells (CSCs).3
CSCs account for about 0.1% of the cancer cells and have been separated from multiple solid tumours, including ovarian cancer, breast, brain, colon, prostate, and so on.4 Over the years, identification of CSCs depends on the specific markers on the surfaces of cell membranes and intracellular markers, including CD44, CD133, and aldehyde dehydrogenase (ALDH).5 CSCs display differentiation and self-renewal, and can form secondary or tertiary tumours. Accumulating evidence has proved that CSCs are linked to cancer initiation, progression, metastasis, relapse, and resistance to conventional therapies.6 In ovarian cancer, high expression of the CSC transcription factor was independently associated with poor overall survival.3,7 Thus, targeted therapy of CSCs is considered a potential therapeutic strategy against ovarian cancer.8
Natural compounds have aroused increasing attention in the field of cancer and CSCs due to their high activity and low side effects.9–11 Currently, about 60% of approved anticancer drugs come from unmodified natural products, their semisynthetic derivatives, or molecules synthesized based on natural products.12,13 18β-glycyrrhetinic acid (GA, 1, Fig. 1), a pentacyclic triterpenoid extracted from the root of Licorice, exerts a wide range of pharmacological activities including anti-inflammatory, antiviral, anti-oxidative and anticancer properties.14 GA has been investigated moderate anti-proliferative activity in ovarian cancer, breast, hepatoma, and stomach cancer cells.15 Although GA was not as active as other triterpenes, it can be easily obtained in large amounts. Thus, using GA as a scaffold to develop new low-toxicity and high-effectivity antitumor agents has aroused the great interest of scientists.16–20 The other natural compound cinnamic acid isolated from cinnamon or blossom, was found to have extensive physiological, including certain inhibitory activity against cancer cells.21,22 The amide bond is an important functional group in medicines as hydrogen bond donors and/or receptors. Therefore, we designed a series of GA derivatives containing cinnamamide moiety to investigate their anti-ovarian cancer activities.
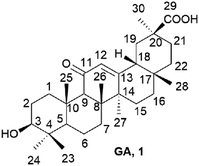 |
| Fig. 1 Structures of 18β-glycyrrhetinic acid (GA). | |
In this study, we designed and synthesized a series of GA derivatives containing CA moiety with piperazine or ethylenediamine (EDA) as linkers. These semisynthetic derivatives were tested for anti-proliferation activities in vitro against ovarian cancer cells. Compound 7c exhibited the strongest growth inhibition activities and the best selectivity among all of the target compounds. Thus 7c was selected for further biological assays, including apoptosis induction, migration inhibition, and the colony and spheres formation ability, the percentage of ovarian CSCs with CD44+ or CD133+ biomarker, and activity of ALDH.
2. Results and discussion
2.1 Chemistry
The preparation of the target compounds is outlined in Scheme 1. Compound 2a–2i, as the starting material, was reacted with SOCl2 in dichloromethane to obtain the corresponding acyl chloride, and then dropwise added into the solution of anhydrous piperazine in glacial acetic acid to afford 3a–3i. Compound 4a–4i was prepared in 63–87% yield by condensation between GA and 3a–3i in the presence of HATU/DIPEA at 100 °C for five hours. Methyl cinnamate 5a–5i can be easily obtained with cinnamoyl chloride and methanol in almost quantitative yield. 6a–6i was achieved by the ammonolysis of the ester group of 5a–5i with EDA under refluxing. Compound 7a–7i was synthesized in a similar way to 4a–4i in 50–70% yield, except that the reaction temperature changed to room temperature. The structures of GA derivatives were characterized using spectral methods.
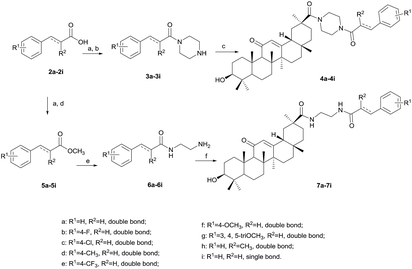 |
| Scheme 1 Reagents and conditions: (a) SOCl2, r.t., 1 h; (b) piperazine, CH3COOH, CH2Cl2, r.t., 2 h; (c) GA, HATU, DIPEA, DMF, 100 °C, 5 h; (d) CH3OH, CH2Cl2, r.t., 0.5 h; (e) EDA, reflux, 2 h; (f) GA, HATU, DIPEA, DMF, r.t., 5 h. | |
2.2 Biology
2.2.1 Evaluation of antiproliferative activity and selectivity. Eighteen GA derivatives were screened for their antiproliferative activities in vitro against human ovarian cancer cell line SKOV3 and OVCAR3 by MTT assay. The activities of GA and paclitaxel were used as a reference. As shown in Table 1, the majority of these derivatives exhibited stronger antiproliferative activities against two ovarian cancer cells when compared with GA, but weaker than paclitaxel. Compound 7a–7i with EDA as the linker showed higher antiproliferative activity than 4a–4i with piperazine as the linker. So 7a–7i were selected to test a selectivity index (SI, IC50 IOSE80 cell line/IC50 ovarian cancer cell line), and the results were summarized in Table 2. Compound 7a, 7c, 7e–7h exhibited selectivity with SI value superior to 1.0. Among them, compound 7c demonstrated the most potent antiproliferative activity against the SKOV3 and OVCAR3 cell lines (IC50 value of 27.9 μM and 33.9 μM against SKOV3 and OVCAR3 cell lines, respectively). Furthermore, compound 7c was 2–3 times more active in ovarian cell lines than in the normal ovarian epithelial cells, which indicated that the selectivity of 7c was closed to paclitaxel. These results suggested that the antiproliferative ability was significantly enhanced after the introduction of 4-chloro-substituted aminoethyl cinnamamide moiety at the C-30 carbonyl position of GA. Therefore, we choose the compound 7c to further investigate the anti-ovarian cancer mechanism of action.
Table 1 Cell viability (IC50 values) of GA derivatives in ovarian cancer cells
Compounds |
IC50a (μM) |
Compounds |
IC50a (μM) |
SKOV3 |
OVCAR3 |
SKOV3 |
OVCAR3 |
IC50: concentration that inhibits 50% of cell growth. The values are presented as the mean ± SD (standard error of the mean) from three separated experiments. |
4a |
78.0 ± 0.8 |
71.6 ± 2.4 |
7a |
58.7 ± 1.8 |
53.0 ± 2.0 |
4b |
42.1 ± 1.2 |
69.9 ± 1.8 |
7b |
59.0 ± 1.0 |
53.2 ± 2.2 |
4c |
59.9 ± 1.7 |
68.4 ± 1.2 |
7c |
27.9 ± 1.4 |
33.9 ± 1.1 |
4d |
>100 |
84.6 ± 2.0 |
7d |
>100 |
56.5 ± 2.8 |
4e |
71.3 ± 1.1 |
>100 |
7e |
45.4 ± 2.1 |
54.4 ± 1.0 |
4f |
84.9 ± 1.7 |
78.0 ± 2.2 |
7f |
58.9 ± 1.1 |
48.2 ± 2.0 |
4g |
74.3 ± 1.9 |
63.1 ± 2.6 |
7g |
49.4 ± 3.0 |
44.0 ± 1.8 |
4h |
>100 |
>100 |
7h |
56.7 ± 1.9 |
45.0 ± 1.9 |
4i |
95.0 ± 2.4 |
60.7 ± 2.0 |
7i |
>100 |
48.2 ± 2.4 |
Paclitaxel |
0.43 ± 0.03 |
0.38 ± 0.04 |
GA, 1 |
98.5 ± 1.6 |
72.4 ± 2.2 |
Table 2 Cell viability (IC50 values) of GA derivatives 7a–7i against normal ovarian epithelial cells (IOSE80) and selectivity index (SI)
Compounds |
IC50a (μM) |
SIb |
IOSE80 |
SKOV3 |
OVCAR3 |
IC50: concentration that inhibits 50% of cell growth. The values are presented as the mean ± SD (standard error of the mean) from three separated experiments. SI (selectivity index): IC50 IOSE80 cell line/IC50 ovarian cancer cell line. |
7a |
66.8 ± 0.9 |
1.1 |
1.3 |
7b |
48.4 ± 0.6 |
0.8 |
0.9 |
7c |
86.7 ± 0.9 |
3.1 |
2.6 |
7d |
65.7 ± 3.7 |
— |
1.2 |
7e |
71.8 ± 1.9 |
1.6 |
1.3 |
7f |
92.0 ± 1.1 |
1.6 |
1.9 |
7g |
53.0 ± 0.8 |
1.1 |
1.2 |
7h |
97.6 ± 5.7 |
1.7 |
2.2 |
7i |
>100 |
— |
— |
GA |
>100 |
— |
— |
Paclitaxel |
1.7 ± 0.1 |
4.0 |
4.5 |
2.2.2 Compound 7c induced apoptosis in SKOV3 and OVCAR3 cells. To investigate whether compound 7c inhibited cells proliferation by regulating apoptosis, cell apoptosis analysis was performed in SKOV3 and OVCAR3 cells. We performed flow cytometry using Annexin V and 7-AAD double staining to quantitatively evaluate apoptosis. The percentages of early and late phase apoptosis were significantly increased by compound 7c in a dose dependent manner (Fig. 2). To investigate the molecular mechanism of apoptosis induced by compound 7c, western blot analysis was conducted with the antibodies for apoptosis-associated proteins. As shown in Fig. 3, the expression of Caspase-3 and Bax was greatly increased, and the level of Bcl-2 was decreased significantly by treatment with 7c in SKOV3 and OVCAR3 cells. These results proved that the apoptosis of cells induced by compound 7c contributed to their antiproliferative effects.
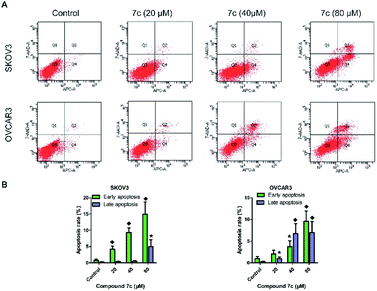 |
| Fig. 2 Compound 7c induced apoptosis in SKOV3 and OVCAR3 cells. Cells were treated with different concentrations of compound 7c for 24 h. (A) Cells were analysed by flow cytometryafter staining with Annexin V and 7AAD. (B) Quantitative analysis of apoptosis was shown. ★P < 0.05, ◆P < 0.01. | |
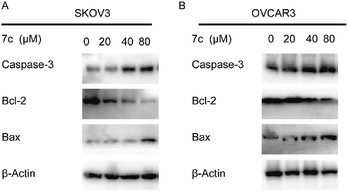 |
| Fig. 3 Effects of compound 7c on the levels of apoptosis-related protein in cells by western blot. (A) The western blot results of 7c in SKOV3 cells. (B) The western blot results of 7c in OVCAR3 cells. | |
2.2.3 Compound 7c inhibited the migration of SKOV3 and OVCAR3 cells. The wound healing assay was performed to evaluate the effect of compound 7c on the migration of SKOV3 and OVCAR3 cells. The cells were treated with compound 7c (22, 44 and 66 μM) for 24 h. As shown in Fig. 4A and B, the migration rate was significantly suppressed by compound 7c in SKOV3 and OVCAR3 cells in a dose-dependent manner. The transwell assay demonstrated that the number of transmembrane cells significantly reduced in the concentration of 7c manner (Fig. 4C and D). Taken together, the results indicated that compound 7c had an inhibitory effect on ovarian cancer cell migration.
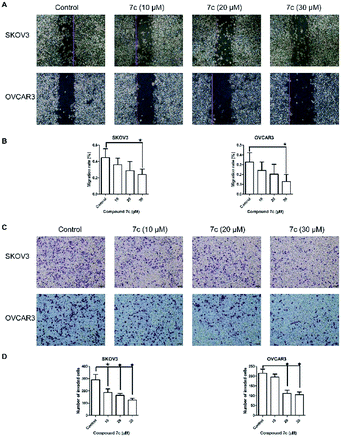 |
| Fig. 4 Compound 7c inhibited the migration of SKOV3 and OVCAR3 cells. (A) Monolayers were wounded by a plastic tip and then incubated for another 24 h with different concentrations of compound 7c. (B) Quantitative analysis of migration ratewas shown. (C) The transwell assay was performed to study the effect of compound 7c on the migration capacity. (D) Quantitative analysis of migrated cells was shown. Images of the wound morphology were acquired by light microscopy. Scale bar, 100 μm. ★P < 0.05, ◆P < 0.01. | |
2.2.4 Compound 7c inhibited cell colony formation. Colony formation is a basic characteristic of ovarian CSCs and represents the malignant potential. The colony formation assay was performed to study further whether compound 7c could inhibit forming colonies. As shown in Fig. 5, compound 7c dose-dependently decreased the number of colonies in SKOV3 and OVCAR3 cells. These results demonstrated that compound 7c significantly suppressed the colony formation and inhibited the unrestricted growth of human ovarian cancer cells.
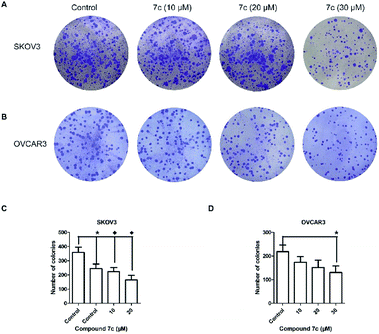 |
| Fig. 5 Compound 7c inhibited the colony formation ability in SKOV3 and OVCAR3 cells. Cells were seeded into 6-well plates and treated by indicated concentrations of compound 7c for 10 days. The colonies were stained with crystal violet dye and photographed. (A) Representative images of the colony formation assay for compound 7c in SKOV3 cells. (B) Representative images of the colony formation assay for compound 7c in OVCAR3 cells. (C) Quantitative data analysis of colony numbers for compound 7c in SKOV3 cells. (D) Quantitative data analysis of colony numbers for compound 7c in OVCAR3 cells. ★P < 0.05, ◆P < 0.01. | |
2.2.5 Compound 7c suppressed the sphere formation. We further determine whether compound 7c can suppress sphere formation that is one of the characteristics of CSCs. We added compound 7c to the secondary spheres derived from SKOV3 and OVCAR3 cells. The sphere formation ability decreased dramatically by showing much fewer number and smaller of spheres treated by compound 7c (Fig. 6). These results suggested that compound 7c attenuated the cancer stem cell characteristics in SKOV3 and OVCAR3 cells.
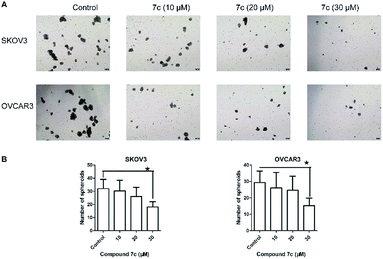 |
| Fig. 6 (A) Compound 7c inhibited the sphere formation ability of SKOV3 and OVCAR3 cells. Single cells were seeded in ultra-low attachment plates. Then cells were seeded to form secondary generation incubated with compound 7c, and the spheroids were photographed. (B) Quantitative analysis of the spheroid numbers was shown. ★P < 0.05. | |
2.2.6 Compound 7c reduced the expression of CSC markers in ovarian cancer cells. Convincing evidence from numerous studies reported that CD44 and CD133 was a vital biomarker of the ovarian CSCs.3 To further study the effects of compound 7c on the proportion of the CD44+ and CD133+ in SKOV3 and OVCAR3 cells, the cells were treated with the indicated concentration of compound 7c for 24 h, followed by staining with the respective antibody (Fig. 7). The results indicated that compound 7c could markedly reduce the proportion of CD44+ and CD133+ cells, which further confirmed the potent inhibitory effect of compound 7c in ovarian CSCs.
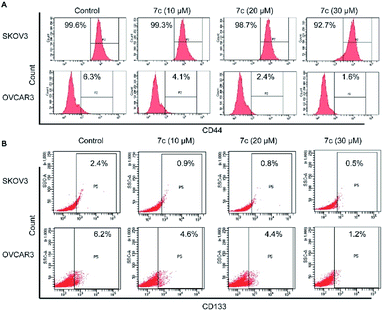 |
| Fig. 7 Compound 7c reduced the CD44+ and CD133+ cell subpopulation of SKOV3 and OVCAR3 cells. (A) The cell population was analysed by flow cytometry with compound 7c for 24 h in SKOV3. (B) The cell population was analysed by flow cytometry with compound 7c for 24 h in OVCAR3 cells. | |
2.2.7 Compound 7c reduced the percentage of ALDH+ cells in ovarian cancer cells. ALDH enzymatic activity has been reported to be one of the most promising markers of ovarian cancer stem cell, and its activity was studied using the ALDHEFLUOR™ assay kit.23 To further study the effects of compound 7c on regulating the CSC characteristics, we assessed the percentage of the ALDH+ cells in SKOV3 and OVCAR3 cells after treated by compound 7c. The SKOV3 and OVCAR3 cells were exposed to 7c for 24 h, followed by staining with ALDH reagent and then detection by flow cytometry (Fig. 8). The results indicated that compound 7c treatment dose-dependently decreased the percentage of ALDH+ cells compared with the control, and provided an additional evidence for the important role of compound 7c in the elimination of ovarian cancer CSCs.
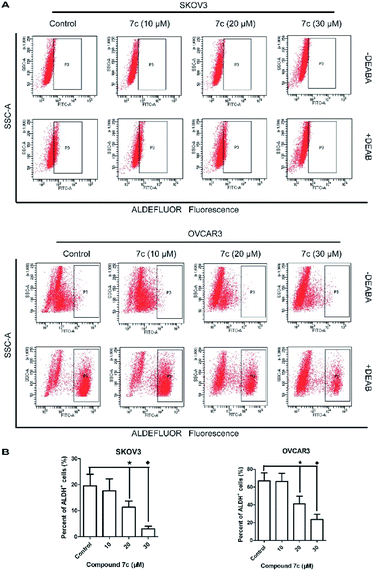 |
| Fig. 8 Compound 7c reduced the population of ALDH+ of the subpopulation of SKOV3 and OVCAR3 cells. (A) Cells were treated with compound 7c for 24 h and FACS analysis of the percentage of ALDH+ cells. (B) Quantification of FACS analysis of the percentage of ALDH+ cells were shown. ★P < 0.05, ◆P < 0.01. | |
3. Conclusions
In the present study, a series of GA derivatives containing cinnamamide moiety were designed and synthesized. Through evaluation of anticancer activity in ovarian cancer cell lines, the results showed that the antiproliferative activities of GA derivatives were significantly stronger compared with GA. The most potent compound 7c was selected for further biological studies in SKOV3 and OVCAR3 cells. The compound 7c could induce apoptosis and suppress migration. In addition, the compound 7c could not only observably decreased the colony and sphere formation ability, but also significantly reduced the CD44+, CD133+, and ALDH+ subpopulation in SKOV3 and OVCAR3. In conclusion, these results showed that compound 7c exhibited the potent elimination of ovarian CSCs and need to be further studied.
4. Experimental section
4.1 Chemistry
Melting points (mp) were determined on an electrically heated X4 digital visual melting point apparatus. 1H NMR and 13C NMR spectra were performed on a Bruker ARX-400 or AVANCE NEO-500 NMR spectrometer in the indicated solvents (TMS as internal standard) with CDCl3 or d6-DMSO as the solvent. Mass spectra (MS) were measured on a Finnigan MAT/USA spectrometer (LC-MS). High-resolution mass spectra (HR-MS) were obtained using a Q-TOF instrument equipped with an ESI source. Most chemicals were commercial suppliers in analytic grade without further purification unless otherwise noted. All of the target compounds were ≥95% purity determined by HPLC analysis.
4.1.1 General procedure for the synthesis of 3a–3i. Compound 2a–2i (5.0 mmol) were added to a round bottom flask containing SOCl2 (10 mL), and the mixture was stirred at room temperature for 1 h. The solution was removed under reduced pressure to obtain the corresponding acyl chloride. A solution of cinnamic acid chloride in dry dichloromethane (5 mL) was dropwise added into the solution of piperazine (10.0 mmol) dissolved in glacial acetic acid (20 mL) and reacted at room temperature for 2 h. After the reaction was finished, the solution was adjusted to pH 7–9 by 40% NaOH, and then extracted with dichloromethane (3×). The organic layer was dried over anhydrous magnesium sulphate, filtered and evaporated. Purification was performed using column chromatography (dichloromethane/methanol = 50/1) to give the desired product 3a–3i.
(E)-3-Phenyl-1-(piperazin-1-yl)prop-2-en-1-one (3a). Yellow solid; mp 86.3–87.5 °C; yield 82%; 1H NMR (500 MHz, d6-DMSO) δ 7.73–7.68 (2H, m), 7.47 (1H, d, J = 15.4 Hz), 7.43–7.34 (3H, m), 7.23 (1H, d, J = 15.4 Hz), 3.61 (2H, m), 3.49 (2H, m), 2.69 (4H, s), 1.23 (1H, s); LC-MS: [M + H]+ 217.1.
(E)-3-(4-Fluorophenyl)-1-(piperazin-1-yl)prop-2-en-1-one (3b). Yellow solid; mp 76.7–77.7 °C; yield 71%; 1H NMR (500 MHz, d6-DMSO) δ 7.77 (2H, dd, J = 8.6, 5.7 Hz), 7.45 (1H, d, J = 15.4 Hz), 7.20 (3H, dd, J = 20.1, 12.0 Hz), 3.59 (2H, m), 3.48 (2H, m), 2.67 (4H, m), 1.22 (1H, s); LC-MS: [M + H]+ 234.1.
(E)-3-(4-Chlorophenyl)-1-(piperazin-1-yl)prop-2-en-1-one (3c). Yellow solid; mp 85.4–86.1 °C; yield 73%; 1H NMR (500 MHz, d6-DMSO) δ 7.82–7.74 (2H, m), 7.47 (1H, d, J = 15.4 Hz), 7.25–7.19 (3H, m), 3.63 (2H, m), 3.51 (2H, m), 2.72 (4H, m), 1.23 (1H, s); LC-MS: [M + H]+ 251.2.
(E)-1-(Piperazin-1-yl)-3-(p-tolyl)prop-2-en-1-one (3d). Yellow solid; mp 77.8–78.4 °C; yield 75%; 1H NMR (500 MHz, CDCl3) δ 7.65 (1H, d, J = 15.4 Hz), 7.41 (2H, d, J = 8.0 Hz), 7.17 (2H, d, J = 7.9 Hz), 6.82 (1H, d, J = 15.4 Hz), 3.72–3.66 (4H, m), 3.05–2.85 (4H, m), 2.36 (3H, s), 1.25 (1H, s); LC-MS: [M + H]+ 231.1.
(E)-1-(Piperazin-1-yl)-3-(4-(trifluoromethyl)phenyl)prop-2-en-1-one (3e). Yellow solid; mp 83.1–83.3 °C; yield 76%; 1H NMR (500 MHz, d6-DMSO) δ 7.74 (2H, d, J = 8.5 Hz), 7.44 (3H, dd, J = 11.9, 3.3 Hz), 7.26 (1H, d, J = 15.5 Hz), 3.60 (2H, m), 3.49 (2H, m), 2.68 (4H, m), 1.22 (1H, s); LC-MS: [M + H]+ 285.2.
(E)-3-(4-Methoxyphenyl)-1-(piperazin-1-yl)prop-2-en-1-one (3f). Yellow solid; mp 79.2–80.4 °C; yield 83%; 1H NMR (500 MHz, d6-DMSO) δ 7.64 (2H, d, J = 8.7 Hz), 7.42 (1H, d, J = 15.4 Hz), 7.07 (1H, d, J = 15.3 Hz), 6.94 (2H, d, J = 8.7 Hz), 3.78 (3H, s), 3.59 (2H, m), 3.47 (2H, m), 2.68 (4H, m); LC-MS: [M + H]+ 247.1.
(E)-1-(Piperazin-1-yl)-3-(3,4,5-trimethoxyphenyl)prop-2-en-1-one (3g). Yellow solid; mp 81.2–82.5 °C; yield 62%; 1H NMR (500 MHz, d6-DMSO) δ 7.42 (1H, d, J = 15.3 Hz), 7.16 (1H, d, J = 15.3 Hz), 7.02 (2H, s), 3.81 (6H, s), 3.67 (3H, s), 3.58 (4H, m), 2.74 (4H, m), 1.22 (1H, s); LC-MS: [M + H]+ 307.3.
(E)-2-Methyl-3-phenyl-1-(piperazin-1-yl)prop-2-en-1-one (3h). Yellow solid; mp 85.2–86.1 °C; yield 60%; 1H NMR (500 MHz, d6-DMSO) δ 7.38–7.27 (5H, m), 6.44 (1H, s), 3.43–3.32 (4H, m), 2.69–2.67 (4H, m), 1.98 (3H, d, J = 1.4 Hz), 1.22 (1H, s); LC-MS: [M + H]+ 231.2.
3-Phenyl-1-(piperazin-1-yl)propan-1-one (3i). Yellow oil; yield 72%; 1H NMR (500 MHz, d6-DMSO) δ 7.30–7.13 (5H, m), 3.36–3.24 (4H, m), 2.79–2.77 (2H, m), 2.59–2.49 (6H, m), 1.22 (1H, s); LC-MS: [M + H]+ 219.1.
4.1.2 General procedure for the synthesis of 4a–4i. GA (100 mg, 0.21 mmol) was dissolved in DMF (10 mL), HATU (100 mg, 0.26 mmol) and DIPEA (0.16 mL, 0.88 mmol) were added and the mixture was stirred at room temperature for 0.5 h. Then compound 3a–3i were added into the solution and reacted at 100 °C for 5 h. Upon completion, the mixture was poured into water and filtered under reduced pressure to obtain the crude product. Purification was performed using preparative TLC (dichloromethane/methanol = 30/1) to give the desired product 4a–4i
(E)-1-(4-(3β-Hydroxy-11-oxo-18β-olean-12-en-30-carbonyl)piperazin-1-yl)-3-phenyl-2-propen-1-one (4a). White solid; mp 169.7–172.2 °C; yield 71%; 1H NMR (400 MHz, CDCl3) δ 7.70 (1H, d, J = 15.3 Hz), 7.52 (2H, s), 7.37 (3H, s), 6.86 (1H, d, J = 15.1 Hz), 5.69 (1H, s), 3.69 (8H, m), 3.27–3.15 (1H, m), 2.77 (1H, d, J = 12.8 Hz), 2.33 (2H, m), 2.12–1.92 (3H, m), 1.88–1.19 (1H, m), 1.68–1.58 (5H, m), 1.50–1.39 (5H, m), 1.36 (3H, s), 1.24 (3H, s), 1.22–1.14 (2H, m), 1.12 (3H, s), 1.11 (3H, s), 1.04 (1H, d, J = 1.3 Hz), 1.00 (3H, s), 0.96–0.91 (1H, m), 0.81 (3H, s), 0.80 (3H, s), 0.69 (1H, d, J = 11.2 Hz); 13C NMR (101 MHz, CDCl3) δ 200.06, 174.25, 169.27, 143.53, 134.96, 129.82, 128.81 (3C), 128.60, 127.82 (2C), 116.45, 78.72, 61.77, 54.90, 48.03, 45.25, 43.94 (2C), 43.88 (2C), 43.25, 39.09 (2C), 37.70, 37.05, 33.01, 32.78, 31.76, 29.67, 28.39, 28.06, 27.26, 27.05, 26.67, 26.37, 23.16 (2C), 18.65, 17.45, 16.36, 15.56; HRMS (ESI): m/z calcd for C43H61N2O4 [M + H]+ 669.4631, found 669.4654.
(E)-1-(4-(3β-hydroxy-11-oxo-18β-olean-12-en-30-carbonyl)piperazin-1-yl)-3-(4-(trifluoromethyl)phenyl)-2-propen-1-one (4b). White solid; mp 179.9–182.1 °C; yield 67%; 1H NMR (400 MHz, CDCl3) δ 7.65 (1H, d, J = 15.3 Hz), 7.51 (2H, dd, J = 8.4, 5.5 Hz), 7.06 (2H, t, J = 8.5 Hz), 6.77 (1H, d, J = 15.4 Hz), 5.67 (1H, s), 3.68 (8H, m), 3.23–3.19 (1H, m), 2.77 (1H, d, J = 13.5 Hz), 2.33–2.28 (2H, m), 2.07–1.96 (3H, m), 1.87–1.80 (1H, m), 1.68–1.57 (5H, m), 1.49–1.39 (5H, m), 1.36 (3H, s), 1.24 (3H, s), 1.18 (2H, d, J = 15.2 Hz), 1.12 (3H, s), 1.11 (3H, s), 1.04 (1H, s), 0.99 (3H, s), 0.96–0.94 (1H, m), 0.81 (3H, s), 0.79 (3H, s), 0.69 (1H, d, J = 11.3 Hz); 13C NMR (101 MHz, CDCl3) δ 200.05, 174.26, 169.27, 165.50, 163.56 (d, J = 251.5 Hz), 142.28, 131.20 (d, J = 4.0 Hz), 129.63 (2C, J = 8.1 Hz), 128.59, 116.14 (d, J = 2.0 Hz), 115.92 (2C, d, J = 21.2 Hz), 78.71, 61.77, 54.91, 48.07, 45.25, 43.94 (2C), 43.84 (2C), 43.26, 39.10 (2C), 37.69, 37.06, 33.06, 32.78, 31.77 (2C), 28.39, 28.07, 27.27, 27.03, 26.68, 26.37, 23.14 (2C), 18.65, 17.46, 16.36, 15.56; HRMS (ESI): m/z calcd for C43H60FN2O4 [M + H]+ 687.4537, found 687.4558.
(E)-1-(4-(3β-Hydroxy-11-oxo-18β-olean-12-en-30-carbonyl)piperazin-1-yl)-3-(4-(chloro)phenyl)-2-propen-1-one (4c). White solid; mp 168.5–169.6 °C; yield 73%; 1H NMR (400 MHz, CDCl3) δ 7.64 (1H, d, J = 15.5 Hz), 7.46 (2H, d, J = 7.8 Hz), 7.35 (2H, d, J = 8.2 Hz), 6.83 (1H, d, J = 14.9 Hz), 5.68 (1H, s), 3.68 (8H, m), 3.24–3.20 (1H, m), 2.78 (1H, d, J = 12.9 Hz), 2.30 (2H, d, J = 21.1 Hz), 2.09–1.97 (3H, m), 1.87–1.80 (1H, m), 1.69–1.58 (5H, m), 1.49–1.40 (5H, m), 1.36 (3H, s), 1.24 (3H, s), 1.19 (2H, d, J = 12.3 Hz), 1.12 (3H, s), 1.11 (3H, s), 1.05 (1H, s), 1.00 (3H, s), 0.95 (1H, d, J = 9.5 Hz), 0.81 (3H, s), 0.80 (3H, s), 0.69 (1H, d, J = 12.1 Hz); 13C NMR (101 MHz, CDCl3) δ 200.07, 174.27, 169.29, 165.38, 142.14, 135.64, 133.45, 129.06 (2C), 128.99 (2C), 128.58, 116.96, 78.71, 61.77, 54.91, 48.07, 45.25, 43.94 (2C), 43.83 (2C), 43.26, 39.11, 39.10, 37.69, 37.06, 33.05, 32.78, 31.77 (2C), 28.38, 28.07, 27.26, 27.03, 26.68, 26.37, 23.14 (2C), 18.65, 17.45, 16.35, 15.56; HRMS (ESI): m/z calcd for C43H60ClN2O4 [M + H]+ 703.4242, found 703.4258.
(E)-1-(4-(3β-Hydroxy-11-oxo-18β-olean-12-en-30-carbonyl)piperazin-1-yl)-3-(p-tolyl)-2-propen-1-one (4d). White solid; mp 172.8–173.9 °C; yield 87%; 1H NMR (400 MHz, CDCl3) δ 7.67 (1H, d, J = 15.3 Hz), 7.42 (2H, d, J = 7.8 Hz), 7.18 (2H, d, J = 7.7 Hz), 6.80 (1H, d, J = 15.2 Hz), 5.68 (1H, s), 3.67 (8H, m), 3.23–3.19 (1H, m), 2.77 (1H, d, J = 13.1 Hz), 2.36 (3H, s), 2.31 (2H, d, J = 13.0 Hz), 2.11–1.94 (3H, m), 1.85–1.79 (1H, m), 1.66–1.57 (5H, m), 1.47–1.38 (5H, m), 1.35 (3H, s), 1.23 (3H, s), 1.18 (2H, d, J = 13.5 Hz), 1.12 (3H, s), 1.10 (3H, s), 1.04 (1H, s), 0.99 (3H, s), 0.96–0.94 (1H, m), 0.81 (3H, s), 0.79 (3H, s), 0.69 (1H, d, J = 11.6 Hz); 13C NMR (101 MHz, CDCl3) δ 200.06, 174.27, 169.29, 165.89, 143.55, 140.16, 132.23, 129.54 (2C), 128.61, 127.82 (2C), 115.28, 78.71, 61.78, 54.93, 48.04, 45.27, 43.95 (2C), 43.92 (2C), 43.27, 39.11 (2C), 37.72, 37.07, 32.99, 32.80, 31.87, 31.78, 28.40, 28.09, 27.28, 27.04, 26.70, 26.38, 23.16 (2C), 21.43, 18.67, 17.47, 16.37, 15.59; HRMS (ESI): m/z calcd for C44H63N2O4 [M + H]+ 683.4788, found 683.4810.
(E)-1-(4-(3β-Hydroxy-11-oxo-18β-olean-12-en-30-carbonyl)piperazin-1-yl)-3-(4-(trifluoromethyl)phenyl)-2-propen-1-one (4e). White solid; mp 172.4–174.3 °C; yield 80%; 1H NMR (400 MHz, CDCl3) δ 7.70 (1H, d, J = 15.5 Hz), 7.63 (4H, s), 6.93 (1H, d, J = 15.4 Hz), 5.67 (1H, s), 3.69 (8H, m), 3.26–3.15 (1H, m), 2.77 (1H, d, J = 13.6 Hz), 2.33 (1H, s), 2.29 (1H, d, J = 12.0 Hz), 2.06–1.94 (3H, m), 1.85 (1H, d, J = 13.1 Hz), 1.68–1.61 (5H, m), 1.47–1.41 (5H, m), 1.36 (3H, s), 1.32 (1H, s), 1.24 (3H, s), 1.19 (1H, d, J = 12.8 Hz), 1.12 (3H, s), 1.11 (3H, s), 1.05 (1H, s), 1.00 (3H, s), 0.97–0.92 (1H, m), 0.81 (3H, s), 0.80 (3H, s), 0.69 (1H, d, J = 11.4 Hz); 13C NMR (101 MHz, CDCl3) δ 200.01, 174.32, 169.22, 165.07, 141.68, 138.49, 128.61, 127.93 (2C), 127.85, 125.78 (2C, q, J = 4.0 Hz), 123.83 (d, J = 272.7 Hz), 119.09, 78.73, 61.81, 54.97, 48.13, 45.28, 43.98 (2C), 43.87 (2C), 43.30, 39.16, 39.12, 37.71, 37.11, 33.13, 32.83, 31.80 (2C), 28.39, 28.09, 27.30, 27.03, 26.74, 26.41, 23.14 (2C), 18.69, 17.49, 16.35, 15.56; HRMS (ESI): m/z calcd for C44H60F3N2O4 [M + H]+ 737.4505, found 737.4526.
(E)-1-(4-(3β-Hydroxy-11-oxo-18β-olean-12-en-30-carbonyl)piperazin-1-yl)-3-(4-(methoxy)phenyl)-2-propen-1-one (4f). White solid; mp 1172.3–174.2 °C; yield 80%; 1H NMR (400 MHz, CDCl3) δ 7.66 (1H, d, J = 15.3 Hz), 7.48 (2H, d, J = 8.7 Hz), 6.90 (2H, d, J = 8.7 Hz), 6.72 (1H, d, J = 15.4 Hz), 5.69 (1H, s), 3.83 (3H, s), 3.68 (8H, m), 3.26–3.16 (1H, m), 2.78 (1H, d, J = 13.4 Hz), 2.32 (2H, d, J = 13.2 Hz), 2.08–1.98 (3H, m), 1.86–1.81 (1H, m), 1.65–1.57 (5H, m), 1.47–1.42 (4H, m), 1.36 (3H, s), 1.31 (1H, s), 1.24 (3H, s), 1.18 (2H, d, J = 16.1 Hz),1.13 (3H, s), 1.12 (3H, s), 1.04 (1H, s), 1.00 (3H, s), 0.96 (1H, d, J = 14.9 Hz), 0.82 (3H, s), 0.80 (3H, s), 0.69 (1H, d, J = 11.4 Hz); 13C NMR (101 MHz, CDCl3) δ 200.06, 174.27, 169.14, 165.85, 160.92, 143.28, 129.45 (2C), 128.60, 127.72, 114.23 (2C), 113.85, 78.76, 61.79, 55.36, 54.93, 48.05, 45.27, 43.96 (2C), 43.91 (2C), 43.28, 39.12 (2C), 37.73, 37.08, 33.00, 32.81, 31.78 (2C), 28.40, 28.08, 27.29, 27.06, 26.71, 26.39, 23.16 (2C), 18.68, 17.48, 16.37, 15.57; HRMS (ESI): m/z calcd for C44H63N2O5 [M + H]+ 699.4737, found 699.4765.
(E)-1-(4-(3β-Hydroxy-11-oxo-18β-olean-12-en-30-carbonyl)piperazin-1-yl)-3-(3,4,5-(trimethoxy)phenyl)-2-propen-1-one (4g). White solid; mp 1163.2–164.8 °C; yield 63%; 1H NMR (400 MHz, CDCl3) δ 7.62 (1H, d, J = 15.3 Hz), 6.72 (3H, d, J = 17.7 Hz), 5.68 (1H, s), 3.90 (6H, s), 3.88 (3H, s), 3.70 (8H, m), 3.22 (1H, m), 2.78 (1H, d, J = 13.2 Hz), 2.34 (1H, s), 2.29 (1H, d, J = 16.0 Hz), 2.10–1.98 (3H, m), 1.87–1.82 (1H, m), 1.71–1.60 (5H, m), 1.48–1.41 (5H, m), 1.37 (3H, s), 1.25 (3H, s), 1.20 (2H, d, J = 15.3 Hz), 1.13 (3H, s), 1.12 (3H, s), 1.05 (1H, s), 1.00 (3H, s), 0.96 (1H, d, J = 10.7 Hz), 0.82 (3H, s), 0.81 (3H, s), 0.70 (1H, d, J = 11.1 Hz); 13C NMR (101 MHz, CDCl3) δ 200.06, 174.30, 169.28, 165.68, 153.42 (2C), 143.64, 139.84, 130.54, 128.60, 115.65, 105.15 (2C), 78.73, 61.81, 60.94, 56.23 (2C), 54.96, 48.14, 45.28, 43.98 (2C), 43.85 (2C), 43.30, 39.15, 39.11, 37.71, 37.10, 33.14, 32.82, 31.79 (2C), 28.39, 28.08, 27.29, 27.03, 26.74, 26.41, 23.14 (2C), 18.69, 17.48, 16.35, 15.55; HRMS (ESI): m/z calcd for C46H67N2O7 [M + H]+ 759.4948, found 759.4978.
(E)-1-(4-(3β-Hydroxy-11-oxo-18β-olean-12-en-30-carbonyl)piperazin-1-yl)-2-methyl-3-phenyl-2-propen-1-one (4h). White solid; mp 1145.3–146.6 °C; yield 80%; 1H NMR (400 MHz, CDCl3) δ; 7.40–7.29 (5H, m), 6.56 (1H, s), 5.68 (1H, s), 3.69–3.62 (8H, m), 3.21 (1H, dd, J = 10.2, 5.1 Hz), 2.78 (1H, d, J = 13.3 Hz), 2.33 (1H, s), 2.29 (1H, d, J = 11.5 Hz), 2.11 (3H, s), 2.05–1.96 (3H, m), 1.87–1.80 (1H, m), 1.69–1.59 (6H, m), 1.48–1.38 (5H, m), 1.36 (3H, s), 1.24 (3H, s), 1.19 (1H, d, J = 13.9 Hz), 1.13 (3H, s), 1.11 (3H, s), 1.05 (1H, s), 1.00 (3H, s), 0.95 (1H, d, J = 14.3 Hz), 0.81 (3H, s), 0.80 (3H, s), 0.69 (1H, d, J = 11.1 Hz); 13C NMR (101 MHz, CDCl3) δ 200.05, 174.28, 172.59, 169.27, 135.57, 132.23, 130.41, 129.02 (2C), 128.57, 128.36 (2C), 127.64, 78.72, 61.77, 54.93, 48.10, 45.26, 43.92 (2C), 43.82 (2C), 43.27, 39.10 (2C), 37.69, 37.06, 33.12, 32.79, 31.77 (2C), 28.37, 28.07, 27.26, 27.03, 26.69, 26.39, 23.12 (2C), 18.66, 17.46, 16.34, 16.20, 15.56; HRMS (ESI): m/z calcd for C44H63N2O4 [M + H]+ 683.4788, found 683.4813.
(E)-1-(4-(3β-Hydroxy-11-oxo-18β-olean-12-en-30-carbonyl)piperazin-1-yl)-3-phenylpropyl-1-one (4i). White solid; mp 134.8–136.1 °C; yield 63%; 1H NMR (400 MHz, CDCl3) δ 7.27 (2H, d, J = 7.0 Hz), 7.20 (3H, d, J = 7.1 Hz), 5.64 (1H, s), 3.60–3.34 (8H, m), 3.22–3.18 (1H, m), 3.10–3.02 (1H, m), 2.96 (2H, t, J = 7.6 Hz), 2.76 (1H, d, J = 13.5 Hz), 2.62 (2H, t, J = 7.6 Hz), 2.31 (1H, s), 2.25 (1H, d, J = 12.2 Hz), 2.07–1.91 (3H, m), 1.86–1.77 (1H, m), 1.64–1.56 (5H, m), 1.46–1.37 (5H, m), 1.34 (3H, s), 1.19 (3H, s), 1.15 (1H, s), 1.11 (3H, s), 1.09 (3H, s), 1.02 (1H, s), 0.98 (3H, s), 0.94 (1H, d, J = 12.5 Hz), 0.78 (6H, s), 0.68 (1H, d, J = 11.5 Hz); 13C NMR (101 MHz, CDCl3) δ 200.00, 174.14, 170.89, 169.25, 140.85, 128.49 (2C), 128.38 (2C), 128.36, 126.24, 78.62, 61.71, 54.86, 47.99, 45.41, 45.20, 43.82 (2C), 43.75 (2C), 43.20, 41.56, 39.05, 37.62, 37.00, 34.85, 32.96, 32.73, 31.69, 31.39, 28.32, 28.03, 27.20, 26.93, 26.62, 26.32, 23.08 (2C), 18.60, 17.40, 16.30, 15.54; HRMS (ESI): m/z calcd for C43H63N2O4 [M + H]+ 671.4788, found 671.4811.
4.1.3 General procedure for the synthesis of 6a–6i. Anhydrous methanol (1 mL, 25 mmol) was added slowly into the solution of cinnamic acid chloride (prepared as described above) in dry dichloromethane (10 mL) and reacted at room temperature for 0.5 h. Then the solvent was evaporated in vacuo to give the crude product, which was not purified for further reaction. The product was dissolved in ethylenediamine and reacted under reflux for 2 h. After the reaction was finished, the solution was extracted with ethyl acetate (3×) and washed by saturated brine (3×). The organic layer was dried over anhydrous magnesium sulphate, filtered and evaporated. Purification was performed using column chromatography (dichloromethane/methanol = 50/1) to give the desired product 6a–6i.
N-(2-Aminoethyl)cinnamamide (6a). Yellow oil; yield 52%; 1H NMR (500 MHz, d6-DMSO) δ 8.12 (1H, s), 7.55 (2H, d, J = 7.1 Hz), 7.42–7.34 (4H, m), 6.63 (1H, d, J = 15.8 Hz), 3.19 (2H, dd, J = 12.2, 6.2 Hz), 2.65 (2H, t, J = 6.4 Hz), 1.22 (1H, s); LC-MS: [M + H]+ 191.1.
(E)-N-(2-Aminoethyl)-3-(4-fluorophenyl)acrylamide (6b). Yellow oil; yield 61%; 1H NMR (500 MHz, d6-DMSO) δ 8.52 (1H, t, J = 5.5 Hz), 7.64 (2H, dd, J = 8.7, 5.6 Hz), 7.47 (1H, d, J = 15.9 Hz), 7.26 (2H, t, J = 8.8 Hz), 6.60 (1H, d, J = 15.9 Hz), 3.42 (2H, dd, J = 12.1, 6.1 Hz), 2.90 (2H, t, J = 6.2 Hz), 1.23 (2H, s); LC-MS: [M + H]+ 209.1.
(E)-N-(2-Aminoethyl)-3-(4-chlorophenyl)acrylamide (6c). Yellow oil; yield 51%; 1H NMR (500 MHz, d6-DMSO) δ 8.16 (1H, t, J = 5.4 Hz), 7.58 (2H, d, J = 8.5 Hz), 7.46 (2H, d, J = 8.5 Hz), 7.40 (1H, d, J = 15.8 Hz), 6.63 (1H, d, J = 15.8 Hz), 3.21 (2H, dd, J = 12.2, 6.2 Hz), 2.67 (2H, t, J = 6.4 Hz), 1.22 (2H, s); LC-MS: [M + H]+ 225.2.
(E)-N-(2-Aminoethyl)-3-(p-tolyl)acrylamide (6d). Yellow oil; yield 56%; 1H NMR (500 MHz, d6-DMSO) δ 8.08 (1H, t, J = 5.3 Hz), 7.43 (2H, d, J = 8.0 Hz), 7.36 (1H, d, J = 15.8 Hz), 7.21 (2H, d, J = 7.9 Hz), 6.57 (1H, d, J = 15.8 Hz), 3.18 (2H, dd, J = 12.2, 6.2 Hz), 2.64 (2H, t, J = 6.4 Hz), 2.31 (3H, s), 1.22 (2H, s); LC-MS: [M + H]+ 205.1.
(E)-N-(2-Aminoethyl)-3-(4-(trifluoromethyl)phenyl)acrylamide (6e). Yellow oil; yield 60%; 1H NMR (500 MHz, d6-DMSO) δ 8.59 (1H, t, J = 5.6 Hz), 7.84–7.76 (4H, m), 7.54 (1H, d, J = 15.9 Hz), 6.78 (1H, d, J = 15.9 Hz), 3.43 (2H, q, J = 6.1 Hz), 2.91 (2H, t, J = 6.3 Hz), 1.23 (2H, s); LC-MS: [M + H]+ 259.2.
(E)-N-(2-Aminoethyl)-3-(4-methoxyphenyl)acrylamide (6f). Yellow oil; yield 68%; 1H NMR (500 MHz, d6-DMSO) δ 8.03 (1H, m), 7.49 (2H, d, J = 8.7 Hz), 7.35 (1H, d, J = 15.8 Hz), 6.96 (2H, d, J = 8.7 Hz), 6.47 (1H, d, J = 15.8 Hz), 3.77 (3H, s), 3.20–3.17 (2H, m), 2.66–2.62 (2H, m), 1.22 (2H, s); LC-MS: [M + H]+ 221.1.
(E)-N-(2-Aminoethyl)-3-(3,4,5-trimethoxyphenyl)acrylamide (6g). Yellow oil; yield 52%; 1H NMR (500 MHz, d6-DMSO) δ 8.04 (1H, t, J = 5.5 Hz), 7.35 (1H, d, J = 15.7 Hz), 6.88 (2H, s), 6.58 (1H, d, J = 15.7 Hz), 3.80 (6H, s), 3.67 (3H, s), 3.21–3.17 (2H, m), 2.65 (2H, m), 1.22 (2H, s); LC-MS: [M + H]+ 281.1.
(E)-N-(2-Aminoethyl)-2-methyl-3-phenylacrylamide (6h). Yellow oil; yield 54%; 1H NMR (500 MHz, d6-DMSO) δ 8.06 (1H, t, J = 5.0 Hz), 7.50–7.27 (5H, m), 7.24 (1H, s), 3.53 (1H, s), 3.23 (1H, dd, J = 12.1, 6.2 Hz), 2.72 (1H, t, J = 6.4 Hz), 2.00 (3H, d, J = 1.2 Hz), 1.22 (2H, s); LC-MS: [M + H]+ 205.1.
N-(2-Aminoethyl)-3-phenylpropanamide (6i). Yellow oil; yield 59%; 1H NMR (500 MHz, d6-DMSO) δ 8.23 (1H, s), 7.30–7.11 (5H, m), 3.27–3.24 (2H, m), 2.82–2.76 (4H, m), 2.41–2.38 (2H, m), 1.22 (2H, s); LC-MS: [M + H]+ 193.1.
4.1.4 General procedure for the synthesis of 7a–7i. GA (100 mg, 0.21 mmol) was dissolved in DMF (10 mL), HATU (100 mg, 0.26 mmol) and DIPEA (0.16 mL, 0.88 mmol) were added and the mixture was stirred at room temperature for 0.5 h. Then compound 6a–6i were added into the resulting solution and reacted at room temperature for 5 h. Upon completion, the mixture was poured into water and filtered under reduced pressure to obtain the crude product. Purification was performed using preparative TLC (dichloromethane/methanol = 30/1) to give the desired product 7a–7i.
(E)-1-(4-(3β-hydroxy-11-oxo-18β-olean-12-en-30-carbonyl)ethanediamine-1-yl)-3-phenyl-2-propen-1-one (7a). White solid; mp 168.9–170.1 °C; yield 68%; 1H NMR (400 MHz, CDCl3) δ 7.61 (1H, d, J = 15.8 Hz), 7.50–7.49 (2H, m), 7.34–7.33 (3H, m), 6.68 (1H, s), 6.58 (1H, s), 6.42 (1H, d, J = 14.7 Hz), 5.72 (1H, s), 3.62–3.36 (4H, m), 3.23–3.20 (1H, m), 2.77 (1H, d, J = 13.0 Hz), 2.30 (1H, s), 2.12 (1H, d, J = 10.7 Hz), 2.07–1.95 (1H, m), 1.88 (2H, d, J = 9.1 Hz), 1.83–1.72 (2H, m), 1.67–1.56 (7H, m), 1.45–1.37 (4H, m), 1.35 (3H, s), 1.16 (1H, s), 1.11 (6H, s), 1.01 (3H, s), 0.99 (3H, s), 0.90 (1H, s), 0.80 (3H, s), 0.74 (3H, s), 0.68 (1H, d, J = 9.9 Hz); 13C NMR (101 MHz, CDCl3) δ 200.02, 177.53, 169.18, 167.25, 141.46, 134.65, 129.69, 128.79 (2C), 128.51, 127.89 (2C), 120.30, 78.75, 61.78, 54.94, 48.04, 45.32, 43.64, 43.18, 41.56, 40.67, 40.19, 39.17, 39.12, 37.56, 37.08, 32.74, 31.84, 31.42, 29.48, 28.43, 28.10, 27.28, 26.46, 26.43, 23.36, 18.57, 17.46, 16.35, 15.58; HRMS (ESI): m/z calcd for C41H59N2O4 [M + H]+ 643.4475, found 643.4500.
(E)-1-(4-(3β-hydroxy-11-oxo-18β-olean-12-en-30-carbonyl)ethanediamine-1-yl)-3-(4-(fluoro)phenyl)-2-propen-1-one (7b). White solid; mp 178.2–179.3 °C; yield 50%; 1H NMR (400 MHz, CDCl3) δ 7.57 (1H, d, J = 15.5 Hz), 7.53–7.43 (2H, m), 7.03 (2H, t, J = 8.4 Hz), 6.65 (2H, s), 6.34 (1H, d, J = 15.5 Hz), 5.71 (1H, s), 3.65–3.36 (4H, m), 3.21 (1H, dd, J = 10.2, 5.0 Hz), 2.76 (1H, d, J = 13.3 Hz), 2.30 (1H, s), 2.10 (1H, d, J = 12.3 Hz), 2.01 (1H, dd, J = 12.9, 9.1 Hz), 1.88 (2H, d, J = 14.1 Hz), 1.77 (1H, dd, J = 14.3, 10.7 Hz), 1.68–1.60 (6H, m), 1.42–1.38 (5H, m), 1.35 (3H, s), 1.18 (1H, s), 1.11 (6H, s), 0.99 (6H, s), 0.94 (2H, d, J = 8.2 Hz), 0.80 (3H, s), 0.73 (3H, s), 0.67 (1H, d, J = 11.2 Hz); 13C NMR (101 MHz, d6-DMSO) δ 199.11, 175.54, 169.60, 165.19, 162.68 (d, J = 247.4 Hz), 137.65, 131.54 (d, J = 3.0 Hz), 129.71 (2C, d, J = 8.1 Hz), 127.67, 122.05, 115.86 (2C, d, J = 22.2 Hz), 76.66, 61.20, 54.18, 47.54, 44.82, 43.00 (2C), 42.88, 40.80, 38.84, 38.62, 37.31, 36.70, 32.20, 31.45 (2C), 30.43, 28.84, 28.22, 28.20, 27.03, 26.10, 26.03, 23.10, 18.24, 17.21, 16.15, 16.06; HRMS (ESI): m/z calcd for C41H58FN2O4 [M + H]+ 661.4381, found 661.4402.
(E)-1-(4-(3β-hydroxy-11-oxo-18β-olean-12-en-30-carbonyl)ethanediamine-1-yl)-3-(4-(chloro)phenyl)-2-propen-1-one (7c). White solid; mp 164.7–166.2 °C; yield 70%; 1H NMR (400 MHz, CDCl3) δ 7.54 (1H, d, J = 15.3 Hz), 7.41 (2H, d, J = 8.0 Hz), 7.30 (2H, d, J = 8.0 Hz), 6.90 (1H, s), 6.81 (1H, s), 6.42 (1H, d, J = 15.5 Hz), 5.69 (1H, s), 3.63–3.36 (4H, m), 3.21 (1H, dd, J = 10.0, 4.6 Hz), 2.74 (1H, d, J = 13.4 Hz), 2.29 (1H, s), 2.08 (1H, d, J = 13.8 Hz), 1.98 (1H, d, J = 14.2 Hz), 1.89 (2H, d, J = 11.9 Hz), 1.81–1.71 (2H, m), 1.66–1.56 (6H, m), 1.43–1.35 (5H, m), 1.34 (3H, s), 1.16 (1H, s), 1.10 (6H, s), 0.99 (3H, s), 0.97 (3H, s), 0.89 (1H, d, J = 18.5 Hz), 0.79 (3H, s), 0.71 (3H, s), 0.66 (1H, d, J = 11.3 Hz); 13C NMR (101 MHz, d6-DMSO) δ 199.06, 175.53, 169.54, 165.02, 137.46, 133.83, 133.80, 129.22 (2C), 128.91 (2C), 127.65, 122.93, 76.64, 61.18, 54.16, 47.51, 44.79, 42.97, 42.86, 40.79, 38.88, 38.81, 38.59, 37.29, 36.68, 32.18, 31.43 (2C), 30.40, 28.82, 28.18 (2C), 27.01, 26.08, 26.02, 23.07, 18.21, 17.19, 16.12, 16.03; HRMS (ESI): m/z calcd for C41H58ClN2O4 [M + H]+ 677.4085, found 677.4112.
(E)-1-(4-(3β-hydroxy-11-oxo-18β-olean-12-en-30-carbonyl)ethanediamine-1-yl)-3-(p-tolyl)-2-propen-1-one (7d). White solid; mp 166.8–169.1 °C; yield 52%; 1H NMR (400 MHz, d6-DMSO) δ 8.02 (1H, s), 7.65 (1H, s), 7.41 (2H, d, J = 8.0 Hz), 7.34 (1H, d, J = 15.7 Hz), 7.17 (2H, d, J = 7.8 Hz), 6.51 (1H, d, J = 15.7 Hz), 5.53 (1H, s), 4.31 (1H, d, J = 5.0 Hz), 3.19–3.16 (4H, m), 2.98 (1H, m), 2.58 (1H, d, J = 13.2 Hz), 2.29 (3H, s), 2.27 (1H, s), 2.07–2.00 (2H, m), 1.88–1.86 (1H, m), 1.79–1.75 (1H, m), 1.71–1.63 (1H, m), 1.62–1.55 (2H, m), 1.52–1.46 (2H, m), 1.40–1.37 (1H, m), 1.31 (3H, s), 1.29–1.18 (6H, m), 1.09 (1H, d, J = 8.4 Hz), 1.02 (1H, s), 0.99 (6H, s), 0.89 (3H, s), 0.89 (3H, s), 0.84 (1H, s), 0.67 (6H, s); 13C NMR (101 MHz, d6-DMSO) δ 199.12, 175.57, 169.61, 165.45, 139.12, 138.82, 132.16, 129.53 (2C), 127.69, 127.56 (2C), 121.07, 76.67, 61.23, 54.19, 47.56, 44.84, 43.01, 42.90, 40.82, 38.86, 38.68, 38.64, 37.33, 36.72, 32.21, 31.46 (2C), 30.45, 28.86, 28.27, 28.22, 27.05, 26.12, 26.04, 23.12, 21.03, 18.28, 17.23, 16.18, 16.08; HRMS (ESI): m/z calcd for C42H61N2O4 [M + H]+ 657.4631, found 657.4657.
(E)-1-(4-(3β-hydroxy-11-oxo-18β-olean-12-en-30-carbonyl)ethanediamine-1-yl)-3-(4-(trifluoromethyl)phenyl)-2-propen-1-one (7e). White solid; mp 1174.5–176.9 °C; yield 72%; 1H NMR (400 MHz, d6-DMSO) δ 8.13 (1H, s), 7.75–7.69 (4H, m), 7.63 (1H, s), 7.44 (1H, d, J = 15.7 Hz), 6.72 (1H, d, J = 15.9 Hz), 5.52 (1H, s), 4.28 (1H, d, J = 4.8 Hz), 3.26–3.13 (4H, m), 3.04–2.98 (1H, m), 2.56 (1H, d, J = 13.1 Hz), 2.26 (1H, s), 2.05–1.98 (2H, m), 1.88 (1H, d, J = 6.8 Hz), 1.75 (1H, s), 1.68 (1H, d, J = 13.4 Hz), 1.47 (2H, d, J = 9.6 Hz), 1.39 (1H, d, J = 11.7 Hz), 1.30 (3H, s), 1.27–1.22 (5H, m), 1.08 (1H, d, J = 13.5 Hz), 1.02 (1H, s), 0.99 (3H, s), 0.94 (3H, s), 0.91 (1H, s), 0.88 (3H, s), 0.83 (3H, s), 0.72 (1H, s), 0.66 (6H, s); 13C NMR (101 MHz, d6-DMSO) δ 199.09, 175.57, 169.57, 164.75, 138.94, 137.17, 128.95 (d, J = 9.1 Hz), 128.19 (2C), 127.61 (q, J = 10.1 Hz), 125.75 (2C, q, J = 4.0 Hz), 124.92, 124.16 (d, J = 273.7 Hz), 76.65, 61.19, 54.15, 47.51, 44.80, 43.01, 42.86, 40.78, 38.83, 38.61, 38.51, 37.30, 36.71, 36.66, 32.17, 31.45, 30.40, 28.85, 28.19, 28.15, 27.02, 26.09, 26.03, 23.09, 18.17, 17.19, 16.09, 16.03; HRMS (ESI): m/z calcd for C42H58F3N2O4 [M + H]+ 711.4349, found 711.4374.
(E)-1-(4-(3β-hydroxy-11-oxo-18β-olean-12-en-30-carbonyl)ethanediamine-1-yl)-3-(4-(methoxy)phenyl)-2-propen-1-one (7f). White solid; mp 162.9–165.3 °C; yield 69%; 1H NMR (400 MHz, d6-DMSO) δ 7.97 (1H, s), 7.65 (1H, s), 7.47 (2H, d, J = 8.6 Hz), 7.33 (1H, d, J = 15.7 Hz), 6.92 (2H, d, J = 8.6 Hz), 6.42 (1H, d, J = 15.7 Hz), 5.53 (1H, s), 4.31 (1H, d, J = 5.0 Hz), 3.76 (3H, s), 3.30–3.04 (4H, m), 3.06–2.96 (1H, m), 2.58 (1H, d, J = 12.8 Hz), 2.28 (1H, s), 2.03–2.00 (2H, m), 1.88–1.86 (1H, m), 1.78–1.75 (1H, m), 1.71–1.68 (1H, m), 1.62–1.57 (2H, m), 1.52–1.49 (2H, m), 1.42–1.38 (1H, m), 1.31 (3H, s), 1.27–1.22 (5H, m), 1.09 (1H, d, J = 10.5 Hz), 1.01 (1H, m), 1.00 (6H, s), 0.91 (3H, s), 0.89 (3H, s), 0.83 (1H, d, J = 9.1 Hz), 0.67 (6H, s); 13C NMR (101 MHz, d6-DMSO) δ 199.52, 175.91, 170.00, 165.97, 160.66, 138.93, 129.51 (2C), 128.04, 127.84, 119.98, 114.73 (2C), 77.03, 61.58, 55.64, 54.53, 47.92, 45.21, 43.36, 43.26, 41.16, 39.21, 39.06, 39.00, 37.68, 37.07, 32.56, 31.82 (2C), 30.78, 29.20, 28.63, 28.57, 27.39, 26.46, 26.40, 23.46, 18.65, 17.57, 16.57, 16.44; HRMS (ESI): m/z calcd for C42H61N2O5 [M + H]+ 673.4580, found 673.4603.
(E)-1-(4-(3β-hydroxy-11-oxo-18β-olean-12-en-30-carbonyl)ethanediamine-1-yl)-3-(3,4,5-(trimethoxy)phenyl)-2-propen-1-one (7g). White solid; mp 166.3–167.5 °C; yield 58%; 1H NMR (400 MHz, d6-DMSO) δ 8.02 (1H, s), 7.64 (1H, s), 7.33 (1H, d, J = 15.7 Hz), 6.85 (2H, s), 6.52 (1H, d, J = 15.7 Hz), 5.54 (1H, s), 4.28 (1H, d, J = 5.0 Hz), 3.79 (6H, s), 3.65 (3H, s), 3.26–3.11 (4H, m), 3.00–2.99 (1H, m), 2.57 (1H, d, J = 12.9 Hz), 2.26 (1H, s), 2.03–1.99 (2H, m), 1.88 (1H, m), 1.77–1.74 (1H, m), 1.67–1.62 (1H, m), 1.59–1.56 (2H, m), 1.50–1.46 (2H, m), 1.39 (1H, m), 1.30 (3H, s), 1.29–1.21 (6H, m), 1.09 (1H, d, J = 10.0 Hz), 1.00 (1H, m), 0.99 (3H, s), 0.95 (3H, s), 0.90 (3H, s), 0.88 (3H, s), 0.83 (1H, m), 0.69 (3H, s), 0.65 (3H, s); 13C NMR (101 MHz, d6-DMSO) δ 199.20, 175.47, 169.62, 165.49, 153.09 (2C), 139.13, 138.64, 130.55, 127.81, 121.33, 104.97 (2C), 76.68, 61.25, 60.13, 55.88 (2C), 54.19, 47.53, 44.84, 43.02, 42.91, 40.79, 38.88, 38.81, 38.65, 37.36, 36.71, 32.20, 31.48 (2C), 30.53, 28.89, 28.34, 28.23, 27.05, 26.13, 26.07, 23.15, 18.24, 17.24, 16.23, 16.09; HRMS (ESI): m/z calcd for C44H65N2O7 [M + H]+ 733.4792, found 733.4821.
(E)-1-(4-(3β-hydroxy-11-oxo-18β-olean-12-en-30-carbonyl)ethanediamine-1-yl)-2-methyl-3-phenyl-2-propen-1-one(7h). White solid; mp 145.8–147.2 °C; yield 51%; 1H NMR (400 MHz, d6-DMSO) δ 7.96 (1H, s), 7.70 (1H, s), 7.41–7.27 (5H, m), 7.22 (1H, s), 5.51 (1H, s), 4.31 (1H, d, J = 5.0 Hz), 3.28–3.20 (4H, m), 3.05–2.95 (1H, m), 2.58 (1H, d, J = 12.6 Hz), 2.29 (1H, s), 2.10–2.01 (2H, m), 1.99 (3H, s), 1.89–1.88 (1H, m), 1.80–1.77 (1H, m), 1.73–1.67 (1H, m), 1.62–1.57 (2H, m), 1.52–1.49 (2H, m), 1.47–1.37 (1H, m), 1.30–1.19 (6H, m), 1.32 (3H, s), 1.11 (2H, d, J = 14.3 Hz), 1.01 (6H, s), 0.97 (3H, s), 0.92 (1H, m), 0.89 (3H, s), 0.68 (6H, s); 13C NMR (101 MHz, d6-DMSO) δ 199.09, 175.74, 169.66, 168.84, 136.12, 132.49, 132.30, 129.24 (2C), 128.39 (2C), 127.66, 127.61, 76.65, 61.22, 54.18, 47.60, 44.86, 42.97, 42.92, 40.81, 38.83, 38.59 (2C), 37.30, 36.72, 32.21, 31.43 (2C), 30.51, 28.81, 28.30, 28.20, 27.03, 26.10, 26.00, 23.10, 18.35, 17.21, 16.22, 16.05, 14.27; HRMS (ESI): m/z calcd for C42H61N2O4 [M + H]+ 657.4631, found 657.4654.
(E)-1-(4-(3β-hydroxy-11-oxo-18β-olean-12-en-30-carbonyl)ethanediamine-1-yl)-3-phenylpropyl-1-one (7i). White solid; mp 142.2–143.2 °C; yield 67%; 1H NMR (400 MHz, d6-DMSO) δ 7.80 (1H, s), 7.58 (1H, s), 7.24 (2H, t, J = 7.3 Hz), 7.19–7.11 (3H, m), 5.51 (1H, s), 4.25 (1H, s), 3.17–3.03 (4H, m), 3.03–2.96 (1H, m), 2.82–2.74 (2H, m), 2.58 (1H, d, J = 13.5 Hz), 2.38–2.32 (2H, m), 2.31 (1H, s), 2.10–2.01 (2H, m), 1.87–1.85 (1H, m), 1.78–1.73 (2H, m), 1.63–1.57 (2H, m), 1.54–1.46 (2H, m), 1.49–1.36 (2H, m), 1.33 (3H, s), 1.30–1.20 (5H, m), 1.13 (1H, d, J = 10.5 Hz), 1.02 (3H, s), 1.01 (3H, s), 0.99 (3H, s), 0.96–0.92 (1H, m), 0.90 (3H, s), 0.72 (3H, s), 0.70 (1H, s), 0.68 (3H, s); 13C NMR (101 MHz, d6-DMSO) δ 199.09, 175.36, 171.57, 169.66, 141.33, 128.27 (2C), 128.18 (2C), 127.57, 125.87, 76.64, 61.22, 54.17, 47.66, 44.87, 42.93 (2C), 40.81, 38.82, 38.69, 38.58, 37.30, 37.15, 36.71, 32.20, 31.43 (2C), 31.09, 30.48, 28.75, 28.40, 28.18, 27.02, 26.10, 25.99, 23.08, 18.37, 17.20, 16.20, 16.03; HRMS (ESI): m/z calcd for C41H61N2O4 [M + H]+ 645.4631, found 645.4659.
4.2 Pharmacology
4.2.1 Cell culture and cell viability analysis. In this study, human ovarian cancer cell lines (SKOV3 and OVCAR3) and normal ovarian epithelial cells (IOSE80) were purchased from the Cell Bank of Shanghai Institute of Biochemistry and Cell Biology (Shanghai, China). SKOV3 cells were grown in RPMI-1640 medium supplemented with 10% fetal bovine serum (FBS; Thermo Fisher Scientific, Waltham, MA, USA) and 100 U per mL penicillin-streptomycin (Sigma-Aldrich; Merck KGaA, Darmstadt, Germany) and incubated at 37 °C with 5% CO2. OVCAR3 and IOSE80 cells were grown in Dulbecco's Modified Eagle medium (DMEM, Thermo Fisher Scientific, Waltham, MA, USA). Cell viability was assessed using the MTT assay. The compounds were dissolved in DMSO and the maximum concentration of DMSO did not exceed 0.1% (v/v). Cells were incubated on 96 well plates at1 × 104 cells/well in a final volume of 100 μL medium and cultured for 24 h. Then, cells were treated with different concentrations of compounds for 48 h. Then, 50 μL of MTT (2 mg mL−1) was added to each well, and the cells were incubated for 48 h. The supernatant was removed, and 150 μL of DMSO was added to each well for 10 min to dissolve the formazan crystals. After agitated for 10 min, the absorbance was measured at 490 nm using microplate reader (Molecular Devices, LLC, Sunnyvale, CA, USA). The concentrations to inhibit growth by 50% were calculated with GraphPad Prism 7.
4.2.2 Apoptosis assay. Cells were plated in 6-well plates and treated with compound for 24 h. Then, cells were harvested, washed, and stained with FITC-conjugated annexin V and 7-AAD (BD Biosciences, San Diego, CA, USA) in the dark for 10 min at room temperature. The stained cells were quantitatively determined the apoptotic cells by flow cytometer (BD FACSCanto, San Jose, CA, USA).
4.2.3 Western blot analysis. Cells were treated with compound for 24 h. Then, cells were harvested and lysed by RIPA buffer. An equivalent amount of protein was separated by 10% SDS-PAGE and then transferred into PVDF membrane (Millipore, Bedford MA). The membrane was blocked with 5% defatted dry milk and sequentially incubated with primary antibodies overnight at 4 °C and secondary antibodies at room temperature for 1 h. Antibodies were obtained from Cell Signalling Technology (Beverly, MA, USA). The membrane was washed with TBST buffer, and the bands were detected using an in an AlPHAImager 2200 (BioTechne, Minneapolis, MN, USA).
4.2.4 Wound healing assay. A total of 1 × 105 cells were seeded in 6-well culture plates and were allowed to grow to 80% confluence. An artificial wound was created by a sterile 10 μLtip, and the detached cells were washed off gently. Then, cells were incubated in the presence of different concentrations compound. Finally, the gap created was measured after 24 h using an inverted microscope (CKX71, Olympus Corporation, Tokyo, Japan). The wound width was analysed by ImageJ software.
4.2.5 Cell migration assay. Cell migration was studied with transwell chambers in 24-well plates (Corning Inc., Tewksbury, MA, USA). 5 × 104 cells in suspension serum-free medium containing compound were seeded into the upper chambers. A total volume of 0.6 mL medium contained 10% FBS was added to the lower chamber. After 24 h, cells located in the upper membrane were removed lightly. The bottom of the membrane was stained with 0.5% crystal violet (Sigma-Aldrich; Merck KgaA, Darmstadt, Germany) for 15 min after fixed with 4% paraformaldehyde for 15 min. The crystal violet positive cells were counted.
4.2.6 Colony formation assay. 300 cells were diluted in 2 mL culture medium and plated in 6-well plates for 24 h. Then, cells were treated with compound at indicated concentrations. The cells were then cultured for 10 days. Finally, the cells were fixed with 4% paraformaldehyde for 30 min and stained with 0.5% crystal violet for 15 min. Plates were photographed, and colonies were counted by ImageJ software. Images are shown as representatives of three independent experiments.
4.2.7 Sphere formation assay. Cells were seeded at a density of 5 × 103 cells per well in 6-well ultralow attachment plates (Corning Inc., Corning, NY, USA) at 37 °C with 5% CO2. Cells were incubated with DMEF/F12 (1
:
1) with B-27 (Thermo Fisher Scientific, Grand Island, NY, USA), 10 ng mL−1 fibroblast growth factor-basic (bFGF, R&D, Minneapolis, MN, USA), and 10 ng mL−1 epidermal growth factor (EGF, Gibco, Carlsbad, CA, USA). After 10 days, tumourspheres were harvested, and then equal cells were plated to form secondary generation. The compound was added at indicated concentrations after plating for 24 h. Cells were cultured for a subsequent 10 days. The culture medium with the compound was replaced three times during incubation. The cells were visualized, and the number of spheres (>50 μm) was counted using an inverted microscope (CKX71, Olympus Corporation, Tokyo, Japan)
4.2.8 Flow cytometry analysis. Cells were treated with compound for 24 h and then harvested. Cells were washed with cold phosphate-buffered saline (PBS) and counted. The cell of each group was adjusted to 1 × 106 cells per mL. Approximately 100 μL of the cell suspension was incubated with specific fluorescein isothiocyanate (FITC)-conjugated anti-human CD44 or CD133 (BD Biosciences, San Diego, CA, USA) in the dark for 0.5 h. IgGof the same isotype and same species was used as an isotype control for each antibody. Cells were analysed using a flow cytometer (BD FACS Canto, San Jose, CA, USA).
4.2.9 ALDEFLUOR™kit ALDH analysis. The population of ALDH+ was measured by an ALDEFLUOR™ assay kit according to the manufacturer's instructions (Stemcell Technologies, Vancouver, BC, Canada). Cells were treated with compound at indicated concentrations for 24 h. Then, cells were harvested and suspended using ALDEFLUOR™ assay buffer to prepare samples with a concentration of 1 × 106 cells per mL. 1 mL sample and 5 μL activated ALDEFLUOR reagent was added into a tube and mixed rapidly. Next, 0.5 mL mixture was transferred to another tube with an added 5 μL ALDH inhibitor diethylaminbenzaldehyde (DEAB) as a negative control. All tubes were mixed quickly and incubated for 40 minutes at 37 °C. All samples were centrifuged and resuspended in 0.4 mL of ALDEFLUOR™ assay buffer. Finally, all samples were subjected to FACS analysis.
4.2.10 Statistical analysis. Data were presented as means ± standard deviations (SD), and statistical analysis was conducted using SPSS 19.0 software. The significance of the difference between compared groups was determined with the student's t-test. All data are representative of the experiment carried out in triplicate. The mean was considered significantly different if P < 0.05.
Conflicts of interest
The authors declare no conflict of interest.
Acknowledgements
This work was supported by the Natural Science Foundation of Shandong Province (ZR2016HB05, ZR2016HB45 and ZR2019PC030), the open project of Shandong Collaborative Innovation Centre for Antibody Drugs (CIC-AD1827), the Postdoctoral Science Foundation of China (2018M632607), and the National Natural Science Foundation of China (81600087).
Notes and references
- B. M. Reid, J. B. Permuth and T. A. Sellers, Cancer Biol. Med., 2017, 14, 9–32 CAS.
- L. A. Torre, B. Trabert, C. E. DeSantis, K. D. Miller, G. Samimi, C. D. Runowicz, M. M. Gaudet, A. Jemal and R. L. Siegel, CA A Cancer J. Clin., 2018, 68, 284–296 CrossRef PubMed.
- Z. Pieterse, M. A. Amaya-Padilla, T. Singomat, M. Binju, B. D. Madjid, Y. Yu and P. Kaur, Int. J. Biochem. Cell Biol., 2019, 106, 117–126 CrossRef CAS PubMed.
- J. E. Visvader and G. J. Lindeman, Nat. Rev. Cancer, 2008, 8, 755–768 CrossRef CAS PubMed.
- M. E. Toledo-Guzman, G. D. Bigoni-Ordonez, M. Ibanez Hernandez and E. Ortiz-Sanchez, World J. Stem Cells, 2018, 10, 183–195 CrossRef PubMed.
- B. C. Prager, Q. Xie, S. Bao and J. N. Rich, Cell Stem Cell, 2019, 24, 41–53 CrossRef CAS PubMed.
- M. K. Siu, E. S. Wong, D. S. Kong, H. Y. Chan, L. Jiang, O. G. Wong, E. W. Lam, K. K. Chan, H. Y. Ngan, X. F. Le and A. N. Cheung, Oncogene, 2013, 32, 3500–3509 CrossRef CAS PubMed.
- A. Mihanfar, J. Aghazadeh Attari, I. Mohebbi, M. Majidinia, M. Kaviani, M. Yousefi and B. Yousefi, J. Cell. Physiol., 2019, 234, 3238–3253 CrossRef CAS PubMed.
- W. F. Taylor and E. Jabbarzadeh, Am. J. Cancer, 2017, 7, 1588–1605 CAS.
- F. Pistollato, R. Calderon Iglesias, R. Ruiz, S. Aparicio, J. Crespo, L. Dzul Lopez, F. Giampieri and M. Battino, Cancer Lett., 2017, 411, 191–200 CrossRef CAS PubMed.
- X. Li, H. Wang, J. Ding, S. Nie, L. Wang, L. Zhang and S. Ren, Eur. J. Pharmacol., 2019, 842, 146–156 CrossRef CAS PubMed.
- D. J. Newman and G. M. Cragg, J. Nat. Prod., 2007, 70, 461–477 CrossRef CAS PubMed.
- G. M. Cragg and D. J. Newman, Biochim. Biophys. Acta, 2013, 1830, 3670–3695 CrossRef CAS PubMed.
- H. Hussain, I. R. Green, U. Shamraiz, M. Saleem, A. Badshah, G. Abbas, N. U. Rehman and M. Irshad, Expert Opin. Ther. Pat., 2018, 28, 383–398 CrossRef CAS PubMed.
- X. F. Wang, Q. M. Zhou, Y. Y. Lu, H. Zhang, S. Huang and S. B. Su, Expert Opin. Ther. Targets, 2015, 19, 577–587 CrossRef CAS PubMed.
- F. Zhou, G. R. Wu, D. S. Cai, B. Xu, M. M. Yan, T. Ma, W. B. Guo, W. X. Zhang, X. M. Huang, X. H. Jia, Y. Q. Yang, F. Gao, P. L. Wang and H. M. Lei, Eur. J. Med. Chem., 2019, 178, 623–635 CrossRef CAS PubMed.
- L. Jin, L. Dai, M. Ji and H. Wang, Bioorg. Chem., 2019, 85, 179–190 CrossRef CAS PubMed.
- R. Wang, W. Yang, Y. Fan, W. Dehaen, Y. Li, H. Li, W. Wang, Q. Zheng and Q. Huai, Bioorg. Chem., 2019, 88, 102951 CrossRef CAS PubMed.
- B. Xu, G. R. Wu, X. Y. Zhang, M. M. Yan, R. Zhao, N. N. Xue, K. Fang, H. Wang, M. Chen, W. B. Guo, P. L. Wang and H. M. Lei, Molecules, 2017, 22, 924 CrossRef PubMed.
- Y. Li, L. Feng, Z. F. Song, H. B. Li and Q. Y. Huai, Molecules, 2016, 21, 199 CrossRef PubMed.
- P. Su, Y. Shi, J. Wang, X. Shen and J. Zhang, Anti Cancer Agents Med. Chem., 2015, 15, 980–987 CrossRef CAS PubMed.
- Z. Zhao, H. Song, J. Xie, T. Liu, X. Zhao, X. Chen, X. He, S. Wu, Y. Zhang and X. Zheng, Eur. J. Med. Chem., 2019, 173, 213–227 CrossRef CAS PubMed.
- D. Kim, B. H. Choi, I. G. Ryoo and M. K. Kwak, Cell Death Dis., 2018, 9, 896 CrossRef PubMed.
Footnote |
† Electronic supplementary information (ESI) available. See DOI: 10.1039/c9ra04961d |
|
This journal is © The Royal Society of Chemistry 2019 |
Click here to see how this site uses Cookies. View our privacy policy here.