DOI:
10.1039/C9RA06177K
(Paper)
RSC Adv., 2019,
9, 30292-30301
Characterization and comparison of Re-Du-Ning aerosol particles generated by different jet nebulizers
Received
8th August 2019
, Accepted 18th September 2019
First published on 25th September 2019
Abstract
Inhalation therapy is the first-line therapy for the treatment of respiratory diseases. Re-Du-Ning inhalation solution (RIS) is an aerosol derivative from the Re-Du-Ning injection and has been clinically used to treat respiratory diseases like pneumonia for more than twenty years in China. However, the aerosolization and inhalation performances of RIS using different nebulizers have not been characterized, which may affect the therapeutic effects of RIS on respiratory diseases. We investigated the inhalation performances of RIS using five different nebulizers utilizing Spraytec, breath simulator of BRS 2000 and NGI techniques. We tested 5 different types of jet nebulizer, using RIS and an adult breathing pattern, to determine the difference in aerosol delivery over time. The particle size distribution of RIS was monitored by a Spraytec laser particle sizer. Fine particle fraction (FPF) and mass median aerodynamic diameter (MMAD) for RIS were measured using NGI. Aerosol deposited on the filter was analysed using HPLC. Nebulization time was much longer for the Pari Boy SX (red) nebulizer than for the other nebulizers, with the minimum delivery rate (DR) and the maximum total delivered dose (TDD) and total exhalation dose (TED). Nebulization time for Pari Boy SX (blue) was the lowest, with the highest DR and the lowest TDD and TED. Furthermore, the aerodynamic particle size of RIS was much larger for the Pari blue and Pari LC Plus than other nebulizers. Pari red produced the smallest aerodynamic particle size of RIS in these five nebulizers. In addition, a good linear relationship was found between MMAD and D50 in these five nebulizers. The results demonstrated that Pari Boy SX (red) delivered most slowly and produced the smallest aerodynamic particle size of the RIS aerosols, which may be applied to manage lower respiratory diseases. Moreover, Pari LC Plus and Pari Boy SX (blue) emitted quickly and generated larger aerodynamic particle size of RIS aerosols, which could be used to treat upper respiratory diseases. A good linear relationship between MMAD and D50 showed Spraytec could be a reliable technique for the development, evaluation and quality control of aerosol particles of inhalation solution preparations.
Introduction
Inhalation therapy is commonly used to deliver drugs to the lung and is accepted as the first-line therapy for the treatment of respiratory diseases,1 such as asthma,2 pneumonia3 and chronic bronchitis.4 A nebulizer provides a convenient method for the pulmonary delivery of a wide range of drugs, including drug doses too large for delivery by other inhaler devices and combinations of different compatible drugs, especially for the elderly and children's medication.5,6 Notably, various nebulizers (e.g. conventional jet, ultrasonic and vibrating mesh nebulizers) with different properties are widely used in the clinic.7,8 These nebulizers can produce distinct size aerosol particles, which may deposit in different locations of the respiratory system after inhalation, and result in various therapeutic effects on respiratory diseases.9 Therefore, the choice of nebulizer device should be considered carefully and the inhalation performance of these nebulizers for each drug should be determined.
With the growing concerns regarding the adverse effects of chemical-based supplements, Traditional Chinese Medicine (TCM) has increased in popularity, especially for preventing and curing respiratory diseases.10,11 TCM is typically administered orally or topically,12,13 but the barrier lies in the large dosage required to achieve effects in respiratory diseases.14 The aerosols generated by nebulizer allow direct absorption to the bloodstream in lung alveoli, ensuring maximal penetration.15Re-Du-Ning inhalation solution (RIS) is a new preparation derivative from Re-Du-Ning injection, which has been clinically used to treat respiratory diseases like pneumonia for more than twenty years in China.16 This preparation is composed of the flowers of Lonicera japonica Thunb, the fruits of Gardenia jasminoides and Artemisia apiacea at the ratio of 25
:
15
:
12.17 It has been reported that these herbs are of low toxicity and have been used in China for hundreds of years to treat early febrile diseases, exogenous fever and boils.18–21 Previous studies showed that the main compounds in the flowers of Lonicera japonica (e.g. chlorogenic acid),22,23 the fruits of Gardenia jasminoides (e.g. geniposide)24,25 and Artemisia apiacea (e.g. artemisinin)26 have strong anti-inflammatory activities. And our previous work showed that RIS could suppress the secretion of inflammatory mediators through TLR4 signalling pathway in lipopolysaccharide stimulated RAW 264.7 macrophages,27 which suggested the potential anti-inflammatory effects of RIS. However, the nebulizers of RIS are varied and the processes of aerosolization are not systemically controlled, which may negatively impact the efficiency of drug delivery.28 In our present study, for the first time, we used five different nebulizers to generate aerosol particles of RIS. Subsequently, we determined the uniformity of delivered dose (UDD) of RIS by BRS 2000 breath simulator. Next, Spraytec was used to monitor the nebulization process and the size of aerosol droplet of RIS. Additionally, next generation impactor (NGI) was conducted to measure the aerodynamic particle size distribution. After each performance, we also compared these parameters of aerosol particles of RIS produced by five different nebulizers. This study provided guidelines to choose nebulizer for RIS in clinic.
Material
Re-Du-Ning inhalation solution (RIS, batch number: 160337) was supplied by Jiangsu Kanion Pharmaceutical Corporation (Jiangsu, P. R. China). Chlorogenic acid (batch number: 110753-200413) and geniposide (batch number: 110749-200309) were purchased from National Institute for the Control of Pharmaceutical and Biological Products (Beijing, P. R. China). Methanol and phosphoric acid were bought from Thermo Fisher Scientific (Waltham, Massachusetts, USA). Distilled water (Watsons Co., Ltd, Guangzhou, P. R. China) were of HPLC grade and all other chemical reagents used were of analytical grade.
Five nebulizers were involved in the study: Pari Boy SX (blue nozzle insert), Pari Boy SX (red nozzle insert), Pari Junior Boy SX, and Pari LC Plus were obtained from Pari Pharma GmbH (Starnberg, Germany). Yu Yue 403E was purchased from Jiangsu Yu Yue Medical Instrument Co., Ltd (Jiangsu, P. R. China). For each experiment, the nebulizer was filled with 2 mL of RIS.
Methods
Uniformity of delivered dose
The uniformity of delivered dose (UDD) has three components: delivery rate (DR), total delivered dose (TDD) and total exhalation dose (TED), which were determined as previously described.29 Briefly, a breathing simulator (BRS 2000, Copley Scientific Limited, Nottingham, UK) mimicking an adult breathing pattern (15 breath per min, tidal volume of 500 mL, inhalation/expiration is 1
:
1) was used (see Table 1). Each nebulizer was connected to the sine pump through a filter holder (Pari Pharma GmbH, Starnberg, Germany) containing an inhalation filter (F1) and an exhalation filter (F3) using rubber adaptors specifically design to connect the nebulizer mouthpiece to the filter holder (see Fig. 1A).
Table 1 Four different breath profiles of the breath simulator BRS 2000
Profile |
Characteristic |
Adult |
Child |
Baby |
Neonate |
Tidal volume (mL) |
500 |
155 |
50 |
25 |
Breath frequency (cycles per min) |
15 |
25 |
30 |
40 |
Ratio of inhalation to exhalation |
1 : 1 |
1 : 2 |
1 : 3 |
1 : 3 |
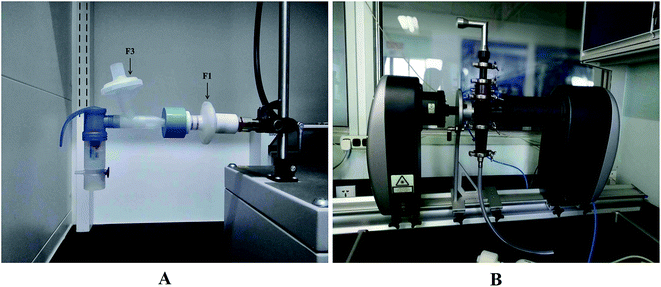 |
| Fig. 1 Nebulizer coupled with filters and Spraytec with inhalation cell. (A) The nebulizer of Pari Boy SX with red nozzle insert and two filter holders F1 contains an inhalation filter and F3 contains an exhalation filter. When the nebulization was run for 1 min, the filter and its filter holder (F1) were replaced by a new filter and holder (F2). The whole nebulization process was lasted for 10 min. DR was the mass emitted per minute, determined by quantifying drug deposited on F1. TDD was calculated by summing the amount of drug collected on F1, F2 and their holders. TED can also be acquired by calculating the drug amount on F3. Effective spray time is the ratio of DR to TDD. Each test was carried on in triplicate. (B) The components of Spraytec with the inhalation cell. | |
The pump was switched on and the nebulizer was activated after 10 s. The nebulizer was then switched off after 60 s. The pump was also stopped after 5 s and the filter (F1) and its filter holder were removed and substituted with a new filter and holder (F2). The breath simulator and nebulizer were then restarted and lasted for 10 minutes. The RIS amount deposited on the two filters and holders, the amount remaining inside the nebulization chamber and the amount left in the glass vial were quantitatively collected using 50% ethanol, respectively. The liquids collected from each filter were transferred into 50 mL volumetric flasks and then diluted with 50% ethanol to the same volume. The amount of chlorogenic acid and geniposide of each sample were determined by HPLC analysis. Delivery rate (DR) represents the mass emitted per minute, was determined by quantifying chlorogenic acid and geniposide collected on the F1, respectively. The total delivered dose (TDD) was calculated by summing the amount of chlorogenic acid and geniposide collected on F1, F2 and their filter holders, respectively. In addition, total exhalation dose could also be obtained by calculating the drug deposited on F3. Effective spray time is the ratio of DR to TDD. Each test was carried on in triplicate.
Real time particle size
Next, the laser diffraction-based technique consisted of a Malvern Spraytec (Malvern Instruments Ltd, Worcestershire, UK) equipped with an inhalation cell, was used to monitor the particle size diameter (PSD) of aerosols generated by the nebulizers. It consists of a Spraytec unit with a USP throat held in place by the inlet of the inhalation cell, and a high capacity vacuum pump (HCP 5, Copley Scientific, Limited, Nottingham, UK) calibrated at 15 L min−1 was attached to the outlet of the cell (see Fig. 1B). The nebulizer was filled with 2 mL of RIS and the total aerosol collection time was 5 min. Various parameters such as D10, D50, D90 and transmission were calculated by the Spraytec software (Malvern Instruments Ltd, Worcestershire, UK). D10, D50, and D90 represent maximal particle size diameter that includes 10%, 50%, and 90% of particles, respectively. Values presented are the average of at least 3 determinations.
Aerodynamic particle size analysis
The NGI (Copley Scientific Limited, Nottingham, UK) was operated at 15 L min−1 using a high capacity vacuum pump (HCP5, COPLEY Scientific, UK) and a critical flow controller (TPK2000, Copley Scientific Limited, Nottingham, UK) to measure the aerosol particle size distribution (PSD) at 5 °C.
The nebulizer was connected to the induction port mouthpiece adapter, filled with 2 mL of RIS, and the aerosol collection time was 5 min. When the aerosol finished, the nebulizer was switched off and the vacuum pump was stopped after 5 s. Drug deposition in the device, throat, all the stages and the filter was determined by HPLC analysis. For accuracy, each test was repeated three times. Results of each collector were imported to the CITDAS system (COPLEY Scientific, UK), and then the mass median aerodynamic diameter (MMAD), the fine particle fraction (FPF) and the geometric standard deviation (GSD) were calculated. Values presented are the average of at least 3 determinations.
HPLC analysis
The contents of chlorogenic acid and geniposide were quantitated by reverse phase HPLC according to the national drug standards of Re-Du-Ning injection.17 Briefly, an LC-20a HPLC system (Shimadzu Corporation, Tokyo, Japan) consisted of a quaternary pump, a standard auto-sampler, a column oven and a diode array detector. Chromatographic separation was performed on Agilent Zorbax SB C18 column (250 mm × 4.6 mm, 5 μm) at 30 °C. A linear gradient system consisted of A (methanol) and B (0.1% phosphoric acid water). The gradient elution profile was as follows: 0–10 min, 15–25% A; 10–30 min 25–35% A; 30–60 min, 35–50% A. The signal was monitored at 237 nm for geniposide and 324 nm for chlorogenic acid, respectively. The flow rate was maintained at 0.8 mL min−1. Re-equilibration duration was 20 min between individual runs. All collected samples were filtered through a 0.45 μm Millipore membrane filter, and an injection volume of samples was 10 μL in each experiment.
HPLC fingerprint analysis of RIS
As reported in our previous work,27 the HPLC fingerprint analysis of RIS was performed to gain chemical profile of RIS.
Results
HPLC fingerprint analysis of RIS
Geniposide was used as the reference substance in the HPLC fingerprint analysis of RIS. Geniposide (peak S) and extra 13 common peaks were assessed with relative retention times and peak area ratios (see Fig. 2 and Table 2).
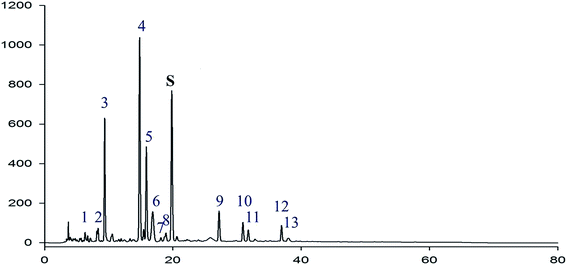 |
| Fig. 2 HPLC fingerprint chromatogram of RIS. Peak S represented geniposide. | |
Table 2 Relative retention times and peak area ratios of geniposide and 13 common peaksa
Peak no. |
Relative retention time |
Peak area ratio |
Peak S represented geniposide, which was selected as the reference substance.
|
1 |
0.286 |
0.03 |
2 |
0.400 |
0.05 |
3 |
0.462 |
0.62 |
4 |
0.759 |
1.28 |
5 |
0.810 |
0.48 |
6 |
0.835 |
0.11 |
7 |
0.912 |
0.01 |
8 |
0.956 |
0.03 |
S |
1.000 |
1.00 |
9 |
1.374 |
0.10 |
10 |
1.613 |
0.08 |
11 |
1.639 |
0.05 |
12 |
1.934 |
0.08 |
13 |
1.957 |
0.01 |
Determination of chlorogenic acid and geniposide
For quantitative consideration, the calibration curves of were established on different concentrations of nine consecutive injections. The linear concentration ranges of the calibration curves for chlorogenic acid and geniposide are 0.11–2.104 μg mL−1 and 0.14–2.792 μg mL−1, respectively. Moreover, regression equations calibrated for chlorogenic acid and geniposide were y = 4 × 107x − 1301.6 (R2 = 0.9998, n = 9) and y = 2 × 107x + 83.544 (R2 = 0.9997, n = 9), respectively. Based on the calibration curve of chlorogenic acid and geniposide, we calculated the delivery rate, total delivered dose, total exhalation dose and aerodynamic particle size distribution using the contents of chlorogenic acid and geniposide in each sample, which was shown in the following results (see Fig. 3B and 5B).
Determination of DR, TDD and TED
Fig. 3 showed that the parameters for five different nebulizers including DR, TDD and TED were calculated by the contents of chlorogenic acid and geniposide in all samples. As shown in Fig. 3, nebulization with the Pari Boy SX with blue nozzle insert resulted in the highest DR (0.96 ± 0.02 mg min−1) and the lowest TDD (3.17 ± 0.20 mg). However, nebulization with Pari Boy SX with red nozzle insert resulted in the minimal DR (0.66 ± 0.01 mg min−1) and the maximal TDD (5.19 ± 0.18 mg). The DR values of other three nebulizers were similar, and the average values range from 0.76 to 0.79 mg min−1. We also observed that nebulization with Pari LC Plus and Yuyue 403E had almost the same TDD values, and nebulization with the Pari Junior Boy SX had larger TDD value that these two nebulizers. Interestingly, the values of TED had similar trends with TDD values. Nebulization with Pari Boy SX with blue nozzle insert had the smallest TED value (0.43 ± 0.04 mg) and Pari Boy SX with red nozzle insert had the largest TED value (2.15 ± 0.10 mg). Values of effective nebulization time (EST) of five nebulizers were 3.30 ± 0.24, 8.05 ± 0.03, 6.16 ± 0.22, 4.40 ± 0.09, 4.71 ± 0.38 min, respectively. Additionally, nebulization of 2 mL RIS with these five nebulizers led to complete nebulization after 10 min. This is certainly an advantage leading to saving of medicament and therapy costs. In line with these results obtained from chlorogenic acid, the results of DR, TDD, TED and EST calculated from geniposide showed the same trend (see Fig. 3).
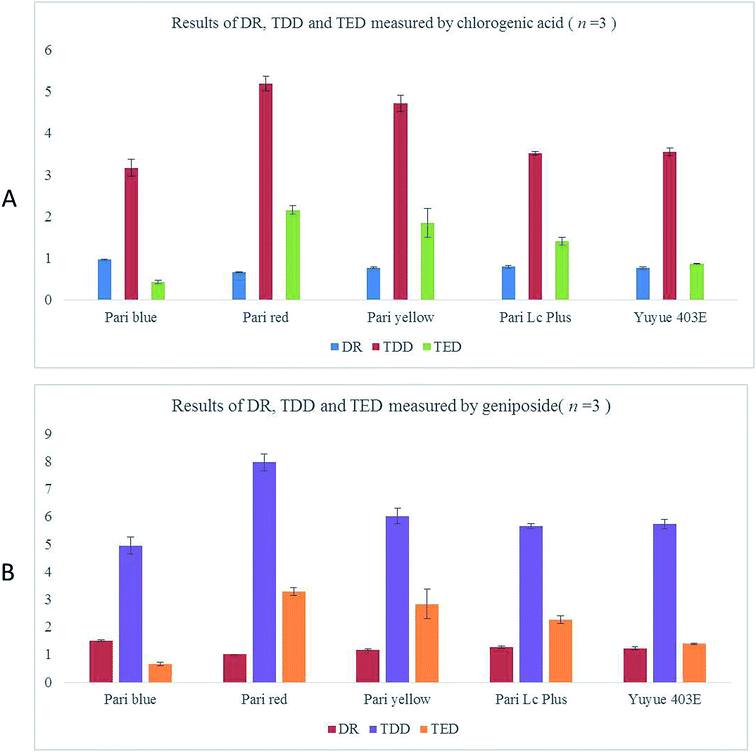 |
| Fig. 3 Uniformity delivery dose results of five different nebulizers determined by HPLC. (A) Results measured by chlorogenic acid (n = 3). (B) Results measured by geniposide (n = 3). DR, TDD and TED represented delivery rate, total delivered dose and total exhalation dose, respectively. | |
Determination of real-time particle size distribution
Next, we also used Spraytec to monitor the real-time particle size distribution and transmission of RIS aerosol generated by different nebulizers in vitro. As shown in Fig. 4, we observed that the value of transmission was high before the nebulization process, indicating there were few aerosol particles generated by nebulizers. The value of transmission was remarkably decreased as the nebulization process started, showing that these nebulizers began to produce the aerosol particles. When RIS was enough to be nebulized, the values of transmission remained steady. The transmission values were then obviously increased as insufficient aerosol particles produced by these nebulizers. The transmission versus time curve was like a U-shaped curve across the whole nebulization process.
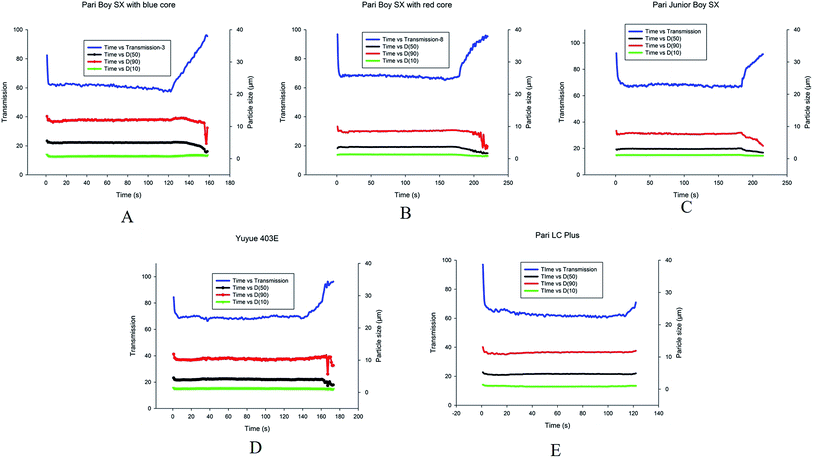 |
| Fig. 4 The Spraytec monitors the result of 5 different jet nebulizers. The inlet gas velocity was calibrated at 15 L min−1. D10, D50, D90 and transmission were calculated automatically in the Spraytec software. D10, D50, and D90 represent maximal particle size diameter that includes 10%, 50%, and 90% of particles, respectively. (A) Pari Boy SX with blue nozzle insert; (B) Pari Boy SX with red nozzle insert; (C) Pari Junior Boy SX; (D) Pari LC Plus; (E) Yuyue 403E. Values given are the mean ± SD at least 3 determinations. | |
As is shown in Table 3, the average values of D10 was from 0.81 to 1.35 μm, D50 was from 3.08 to 4.88 μm, and D90 was from 7.37 to 11.71 μm among these nebulizers. D50 and D90 involve the most aerosol particles generated by nebulizers and are the most commonly used parameters to access aerosol particle size distribution. In our study, we observed that the values of D50 and D90 of Pari Boy SX (blue) were the highest and the values of D50 and D90 of Pari Boy SX (red) were the lowest among these five nebulizers, suggesting that Pari Boy SX (blue) and Pari Boy SX (red) produce the largest and the smallest aerosol particles of RIS, respectively.
Table 3 Results of D10, D50, D90 of five different nebulizers (n = 3)a
Nebulizer |
D
10 (μm) |
D
50 (μm) |
D
90 (μm) |
D
10, D50, and D90 were calculated automatically by the Spraytec software. The inlet gas velocity was calibrated at 15 L min−1. D10, D50, and D90 represent maximal particle size diameter that includes 10%, 50%, and 90% of particles, respectively. Values given are the mean ± SD at least 3 determinations.
|
Pari blue |
0.81 ± 0.06 |
4.88 ± 0.07 |
11.71 ± 0.20 |
Pari red |
1.30 ± 0.17 |
3.08 ± 0.29 |
7.37 ± 0.86 |
Pari yellow |
1.35 ± 0.09 |
3.49 ± 0.06 |
8.13 ± 0.26 |
Pari LC Plus |
1.27 ± 0.32 |
4.67 ± 0.02 |
11.00 ± 0.28 |
Yuyue 403E |
1.30 ± 0.02 |
4.18 ± 0.12 |
10.69 ± 0.33 |
Determination of aerodynamic particle size distribution
In addition, MMAD and FPF, the values involved in the respirable dose calculation, were determined from the aerodynamic particle size distribution obtained from the NGI. As shown in Fig. 5 and Table 4, the highest values of MMAD among these nebulizers were obtained with Pari LC Plus and Pari blue. However, Pari LC Plus and Pari blue presented the lowest values of FPF with 44.7% and 45.7%, respectively. The Pari yellow and Yuyue 403E produced aerodynamic droplet size at 4.21 and 4.58 μm, respectively. The values of FPF in these two nebulizers were 58.8% and 52.6%, respectively. Meanwhile, it was found that the lowest aerodynamic particle size (3.12 μm) of RIS was generated by Pari red with the highest FPF value (76.0), showing that the highest percentages of particles produced by Pari red are below 5 μm. Additionally, similar trend was observed in the results of NGI measured by geniposide (see Fig. 5 and Table 5).
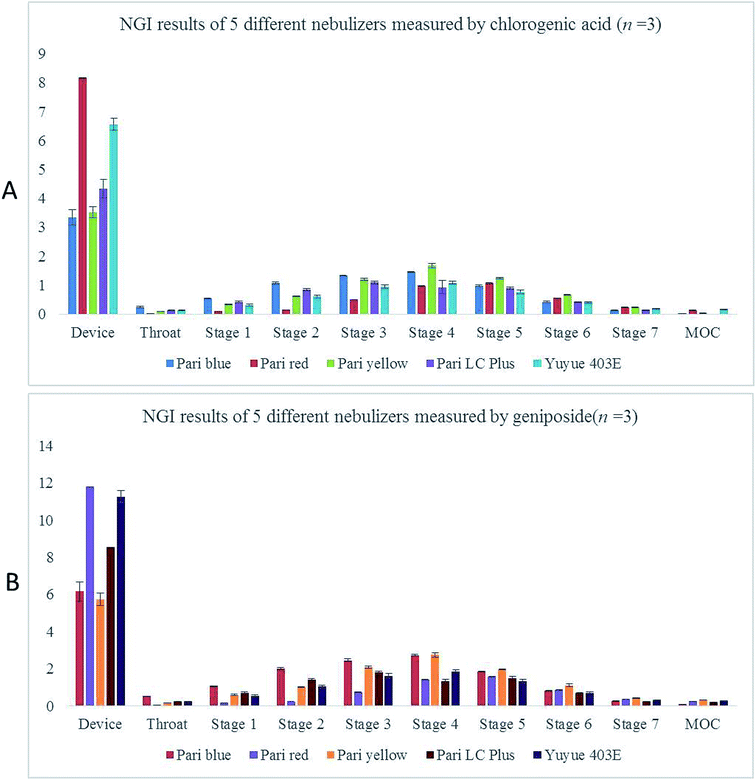 |
| Fig. 5 NGI results of five different nebulizers determined by HPLC. (A) NGI results measured by chlorogenic acid (n = 3). (B) NGI results measured by geniposide (n = 3). | |
Table 4 Aerodynamic particle size distribution (APSD) results of 5 different nebulizers measured by chlorogenic acid (n = 3)
Index |
Pari blue |
Pari red |
Pari yellow |
Pari LC Plus |
Yuyue 403E |
MMAD (μm) |
5.33 ± 0.04 |
3.12 ± 0.00 |
4.21 ± 0.06 |
5.38 ± 0.13 |
4.58 ± 0.13 |
FPF (%) |
44.7 ± 0.46 |
76.0 ± 0.14 |
58.8 ± 0.69 |
45.7 ± 2.52 |
52.6 ± 1.46 |
GSD |
2.10 ± 0.01 |
1.94 ± 0.01 |
2.84 ± 0.54 |
2.08 ± 0.08 |
2.15 ± 0.09 |
Table 5 APSD results of 5 different jet nebulizers calculated by geniposide (n = 3)a
APSD index |
Pari blue |
Pari red |
Pari yellow |
Pari LC Plus |
Yuyue 403E |
MMAD represents mass median aerodynamic diameter, FPF means fine particle fraction and GSD is short for geometric standard deviation. Values given are the mean ± SD at least 3 determinations.
|
MMAD (μm) |
5.25 ± 0.13 |
3.09 ± 0.01 |
4.10 ± 0.04 |
5.23 ± 0.13 |
4.61 ± 0.15 |
FPF (%) |
45.5 ± 0.13 |
75.8 ± 0.09 |
60.0 ± 0.51 |
47.1 ± 2.45 |
52.8 ± 1.73 |
GSD |
2.16 ± 0.07 |
1.97 ± 0.00 |
2.08 ± 0.08 |
2.11 ± 0.05 |
2.15 ± 0.07 |
Correlation analysis of MMAD and D50
In order to evaluate the Spraytec as a reliable measuring instrument for characterizing particle size for RIS, the MMAD measured by NGI were compared with and plotted against the D50 measured by Spraytec. As shown from Fig. 6, a linear relationship existed between the MMAD and the D50 when calculated with the regression. The regression equations for chlorogenic acid and geniposide were y = 0.7645x + 0.6418 (r = 0.9606, n = 15) and y = 0.7918x + 0.5731 (r = 0.9645, n = 15), respectively, indicating a good correlation (R2 of 0.9606 and 0.9645, respectively) between the D50 and MMAD (see Fig. 6).
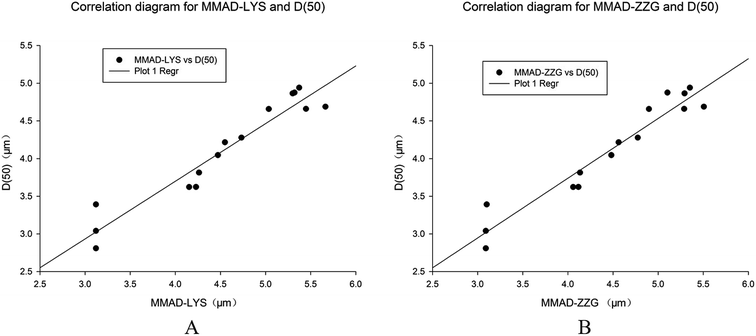 |
| Fig. 6 Correlation analysis of D50 and MMAD. (A) shows the correlation of D50 and MMAD measured by chlorogenic acid; (B) shows the correlation of D50 and MMAD measured by geniposide. | |
Discussion
In recent years, the focus of development in nebulizer technology has been geared towards making devices to be more patient-friendly.30 In terms of providing more efficient delivery, breath-enhanced nebulizer (such as Pari Boy SX, an attached compressor produces compressed gas) can deliver a more stable and consistent aerosol to the lungs when directed to the medication holding chamber through a narrow hole, wasting less medication.31,32 However, different types of breath-enhanced nebulizers are designed to have different drug delivery efficiencies and produce a variety of particle sizes, which may interact with a range of user-specific factors, including drug formulation and breathing pattern, to affect the delivered dose and deposited locations.33 In our present study, we used five classic breath-enhanced nebulizers to produce aerosol of RIS and compared parameters of aerosol particles measured by chlorogenic acid and geniposide, respectively. We found that these nebulizers delivered different doses of RIS and generated aerosol particle with various sizes, which may partially impact therapeutic effect of RIS on respiratory diseases.
BRS 2000 breath simulator is increasingly used in testing in vitro dose uniformity and aerodynamic particle size distribution data and covers a range of breathing patterns from neonate through to neonate, infant, child and adult.34 In our study, we chose an adult breathing pattern and determined the DR, TDD, and TED of aerosol of RIS produced by five nebulizers. The results indicated that the Pari Boy SX with red nozzle insert emitted aerosol of RIS most slowly, and the nebulization dosage and the loss of RIS were the largest among the five nebulizers. The lower flowrate of Pari red means lower air velocity, thus with the smaller hole in the holding chamber. Therefore, the emission of drug particles is more efficient and smaller particles are delivered from the device. In contrast with Pari red, Pari blue emitted aerosol of RIS most quickly, but the amount of aerosol emitted was the fewest among these five nebulizers, suggesting that larger particles were produced by Pari blue.
Particle size is a key parameter in defining the deposition pattern and bio-availability of drug material delivered to the respiratory system using nebulizers.35–37 The optimum particle size for deposition into the lung periphery and the lower airways should be between 1 and 5 μm, whereas, particles with size between 5 and 10 μm deposit mostly in the conducting airways.38–40 Currently, many types of apparatuses have been widely employed for aerosol particle size characterization, such as NGI and Spraytec.41,42 The NGI is a high-performance cascade impactor for classifying aerosol particle into size fractions for testing inhaled drug delivery devices such as nebulizers.43 Moreover, Spraytec is one of the most popular laser diffraction devices specifically designed to address the unique requirements of aerosol and spray droplet characterization and provides real-time and accurate droplet measurement.44–46 Consistent with the results of DR, TDD, and TED, we observed that Pari Boy SX (red) produced the lowest aerosol particle size of RIS among these five nebulizers, suggested by the values of D50 and MMAD. We also found that the largest FPF in Pari Boy SX (red) among these five nebulizers. It has been reported that the higher FPF translates into lower and upper airway doses and higher deposited doses in the lungs.47,48 These results indicated that the small particles generated by Pari Boy SX (red) deposition within the respiratory system is dominated by sedimentation and diffusion within the deep lung. Furthermore, the values of D50 and MMAD of Pari LC Plus and Pari blue were larger than other three nebulizers with lower FPF values, indicating that the aerosol particles of RIS emitted by these two nebulizers may mainly deposit by impaction in the oropharynx and bronchi, prior to reaching the lungs.
In addition, we also found that a good linear relationship between MMAD and D50 in these five nebulizers. The existence of this linear relationship demonstrated that the results of the Spraytec are useful for size evaluation of aerosol particles of RIS, suggesting that the Spraytec could quickly provide a very good evaluation of the aerodynamic behaviour of the particles of RIS produced by these five nebulizers.
Conclusion
Collectively, our study characterized and compared the parameters of five different nebulizers for RIS, including DR, TDD, TED, MMAD, and D50, by BRS 2000 breath simulator, Spraytec, and NGI in vitro. The results showed that Pari Boy SX with red nozzle insert emitted most slowly and produce the smallest aerodynamic particle size of RIS, which may be applied to manage lower respiratory diseases, such as chronic obstructive pulmonary disease (COPD) and asthma. Moreover, Pari LC Plus and Pari Boy SX with blue nozzle insert delivered quickly and larger aerodynamic particle size of RIS, which may deposit in upper or mid airways better, and could be used to treat upper respiratory diseases. Moreover, there is a good linear correlation between MMAD and D50, suggesting that Spraytec can be a useful tool for rapid screening of the most suitable device in the early stage of nebulizer study. This study provides guidelines for the nebulizer choice of RIS in managing respiratory diseases.
Abbreviations
RIS |
Re-Du-Ning inhalation solution |
NGI | Next generation impactor |
PSD | Particle size distribution |
FPF | Fine particle fraction |
MMAD | Mass median aerodynamic diameter |
HPLC | High performance liquid chromatography |
DR | Delivery rate |
TDD | Total delivered dose |
TED | Total exhalation dose |
D
10, D50 and D90 | The maximal particle size diameter that includes 10%, 50% and 90% of particles, respectively |
TCM | Traditional Chinese Medicine |
UDD | The uniformity of delivered dose |
GSD | Geometric standard deviation |
COPD | Chronic obstructive pulmonary disease |
Funding
This study was supported by National Natural Science Foundation of China (81803793), Fundamental Research Funds for the Central Universities (2018-JYBZZ-XJSJJ008).
Authors' contributions
Conceptualization, Gan Luo; Investigation, Cui Li, Yi Zhang, Yonghua Tao and Gan Luo; Methodology and software, Xiaoyan Gao; Formal analysis, Chanjuan Yu and Xinyue Liu; Funding, Yi Zhang and Gan Luo; Writing-origin draft, Gan Luo and Yi Zhang; Writing-review & editing, Brian Chi-Yan Cheng.
Conflicts of interest
The authors declare that they have no competing interests.
Acknowledgements
The study data were obtained from Institute of Chinese Materia Medica, China Academy of Chinese Medical Science. Furthermore, the authors would like to thank the chairman of IDDA: Dr Anny Shen and professor Yonghong Liao from Institute of Medicinal Plant Development, Chinese Academy of Medical Sciences for their technical help.
References
- S. W. Stein and C. G. Thiel, J. Aerosol Med. Pulm. Drug Delivery, 2017, 30, 20–41 CrossRef.
- L. Jahedi, S. R. Downie, B. Saini, H. K. Chan and S. Bosnic-Anticevich, J. Aerosol Med. Pulm. Drug Delivery, 2017, 30, 42–52 CrossRef.
- M. Li, L. Zhu, B. Liu, L. Du, X. Jia, L. Han and Y. Jin, Colloids Surf., B, 2016, 141, 408–416 CrossRef CAS.
- S. Hamada, Y. Ito, S. Imai, T. Oguma, A. Niimi and M. Mishima, Chest, 2011, 139, 930–932 CrossRef.
- A. C. Bos, H. A. Tiddens, K. Tong Minh, I. Heeres, J. L. Overweel-Uijterlinde, A. E. Kok, E. R. Andrinopoulou and H. M. Janssens, J. Cystic Fibrosis, 2016, 15, 645–651 CrossRef PubMed.
- R. Jogi, S. Lahelma, M. Vahteristo, A. Happonen and J. Haikarainen, J. Aerosol Med. Pulm. Drug Delivery, 2019, 32, 88–98 CrossRef CAS.
- O. N. McCallion, K. M. Taylor, M. Thomas and A. J. Taylor, Pharm. Res., 1995, 12, 1682–1688 CrossRef CAS.
- T. C. Carvalho and J. T. McConville, J. Pharm. Pharmacol., 2016, 68, 556–578 CrossRef CAS PubMed.
- M. Beck-Broichsitter, O. Samsonova, J. Nguyen, T. Schmehl, W. Seeger and T. Kissel, Eur. J. Pharm. Sci., 2016, 86, 34–40 CrossRef CAS PubMed.
- Y. C. Park, M. Jin, S. H. Kim, M. H. Kim, U. Namgung and Y. Yeo, J. Ethnopharmacol., 2014, 151, 123–130 CrossRef.
- H. Yoo, M. Kang, S. Pyo, H. S. Chae, K. H. Ryu, J. Kim and Y. W. Chin, J. Ethnopharmacol., 2017, 206, 298–305 CrossRef CAS PubMed.
- P. Li, B. Feng, H. Jiang, X. Han, Z. Wu, Y. Wang, J. Lin, Y. Zhang, M. Yang, L. Han and D. Zhang, Sci. Rep., 2018, 8, 10319 CrossRef.
- E. Thanik, J. A. Wisniewski, A. Nowak-Wegrzyn, H. Sampson and X. M. Li, Ann. Allergy, Asthma, Immunol., 2018, 121, 135–136 CrossRef PubMed.
- C. S. Arulparithi, T. A. Babu, C. Ravichandran, I. Santhanam, B. Sathyamurthi, S. Parivathini and J. Hemachitra, Indian J. Pediatr., 2015, 82, 328–332 CrossRef.
- X. Tang, H. Nian, X. Li, Y. Yang, X. Wang, L. Xu, H. Shi, X. Yang and R. Liu, BMC Complementary Altern. Med., 2017, 17, 380 CrossRef.
- Y. He, Chin. Med. Mod. Dis. Educ. China, 2015, 13, 123–125 Search PubMed.
-
CFDA, National drug standards for Re-Du-Ning injection, 2015, YBZ08202005-2015Z, pp. 1–7 Search PubMed.
- T. F. Tzeng, Y. C. Tzeng, Y. J. Cheng, S. S. Liou and I. M. Liu, Nutrients, 2015, 7, 8670–8684 CrossRef CAS.
- Y. J. Lin, C. C. Lai, C. H. Lai, S. C. Sue, C. W. Lin, C. H. Hung, T. H. Lin, W. Y. Hsu, S. M. Huang, Y. L. Hung, N. Tien, X. Liu, C. L. Chen and F. J. Tsai, Eur. J. Med. Chem., 2013, 62, 206–213 CrossRef CAS.
- E. Hsu, Trans. R. Soc. Trop. Med. Hyg., 2006, 100, 505–508 CrossRef CAS.
- F. van der Kooy and S. E. Sullivan, J. Ethnopharmacol., 2013, 150, 1–13 CrossRef CAS.
- S. H. Park, E. Roh, H. S. Kim, S. I. Baek, N. S. Choi, N. Kim, B. Y. Hwang, S. B. Han and Y. Kim, Biochem. Biophys. Res. Commun., 2013, 442, 183–188 CrossRef CAS.
- S. J. Kim, S. J. Yoon, Y. M. Kim, S. W. Hong, S. H. Yeon, K. I. Choe and S. M. Lee, J. Ethnopharmacol., 2014, 155, 256–266 CrossRef.
- X. Zheng, D. Yang, X. Liu, N. Wang, B. Li, H. Cao, Y. Lu, G. Wei, H. Zhou and J. Zheng, Int. Immunopharmacol., 2010, 10, 1209–1219 CrossRef CAS.
- Q. Shi, J. Cao, L. Fang, H. Zhao, Z. Liu, J. Ran, X. Zheng, X. Li, Y. Zhou, D. Ge, H. Zhang, L. Wang, Y. Ran and J. Fu, Int. Immunopharmacol., 2014, 20, 298–306 CrossRef CAS.
- K. H. Park, Y. D. Yoon, S. B. Han, S. J. Oh, J. Yun, C. W. Lee, K. Lee, S. K. Park, H. M. Kim and J. S. Kang, Int. Immunopharmacol., 2012, 14, 580–584 CrossRef CAS PubMed.
- Y. Zhang, B. Chi-Yan Cheng, R. Xie, B. Xu, X. Y. Gao and G. Luo, RSC Adv., 2019, 9, 8912–8925 RSC.
- A. R. Martin and W. H. Finlay, Expert Opin. Drug Delivery, 2015, 12, 889–900 CrossRef CAS.
- F. Buttini, I. Rossi, M. Di Cuia, A. Rossi, G. Colombo, L. Elviri, F. Sonvico and A. G. Balducci, Int. J. Pharm., 2016, 502, 242–248 CrossRef CAS.
- S. Unida, Y. Ito, R. Onodera, K. Tahara and H. Takeuchi, Asian J. Pharm. Sci., 2016, 11, 205–206 CrossRef.
- M. Elphick, D. von Hollen, J. N. Pritchard, K. Nikander, L. E. Hardaker and R. H. Hatley, Expert Opin. Drug Delivery, 2015, 12, 1375–1387 CrossRef CAS.
- H. L. Lin, T. P. Fang, H. S. Cho, G. H. Wan, M. J. Hsieh and J. B. Fink, Pulm. Pharmacol. Ther., 2018, 48, 225–231 CrossRef CAS.
- W. Kamin, F. Erdnuss and I. Kramer, J. Cystic Fibrosis, 2014, 13, 243–250 CrossRef CAS.
-
M. Copley, Assessing the role of breath simulators in OIP testing, 2014, https://www.copleyscientific.com/files/ww/news/COP-JOB-262_Copley-Breath-Simulators-Article.pdf Search PubMed.
- M. Ochowiak, M. Doligalski, L. Broniarz-Press, M. Matuszak and A. Gosciniak, Eur. J. Pharm. Sci., 2016, 85, 53–58 CrossRef CAS.
- E. V. Hillyer, D. B. Price, H. Chrystyn, R. J. Martin, E. Israel, W. M. C. van Aalderen, A. Papi, O. S. Usmani and N. Roche, J. Aerosol Med. Pulm. Drug Delivery, 2018, 31, 111–113 CrossRef.
- R. M. Sarhan, A. A. Elberry, N. S. Abdelwahab, H. Rabea, M. N. Salem and M. E. Abdelrahim, Respir. Care, 2018, 63, 1125–1131 CrossRef.
- R. Biswas, N. A. Hanania and A. Sabharwal, J. Aerosol Med. Pulm. Drug Delivery, 2017, 30, 256–266 CrossRef CAS.
- C. Singh, L. V. Koduri, V. Dhawale, T. D. Bhatt, R. Kumar, V. Grover, K. Tikoo and S. Suresh, Int. J. Pharm., 2015, 495, 627–632 CrossRef CAS.
- C. Darquenne, J. S. Fleming, I. Katz, A. R. Martin, J. Schroeter, O. S. Usmani, J. Venegas and O. Schmid, J. Aerosol Med. Pulm. Drug Delivery, 2016, 29, 107–126 CrossRef.
- X. Song, J. Hu, S. Zhan, R. Zhang and W. Tan, AAPS PharmSciTech, 2016, 17, 380–388 CrossRef.
- S. Wanning, R. Suverkrup and A. Lamprecht, Eur. J. Pharm. Sci., 2017, 96, 1–7 CrossRef CAS.
- X. Zhang, Q. Liu, J. Hu, L. Xu and W. Tan, Acta Pharm. Sin. B, 2014, 4, 79–85 CrossRef.
- G. Pilcer, F. Vanderbist and K. Amighi, Int. J. Pharm., 2008, 358, 75–81 CrossRef CAS.
- P. E. Santangelo, Exp. Therm. Fluid Sci., 2010, 34, 1353–1366 CrossRef.
- A. Haynes, M. S. Shaik, H. Krarup and M. Singh, J. Pharm. Sci., 2004, 93, 349–363 CrossRef CAS.
- R. Ambrus, E. Benke, A. Farkas, I. Balashazy and P. Szabo-Revesz, Eur. J. Pharm. Sci., 2018, 123, 20–27 CrossRef CAS.
- J. Yu, H. K. Chan, T. Gengenbach and J. A. Denman, Eur. J. Pharm. Biopharm., 2017, 119, 224–234 CrossRef CAS.
|
This journal is © The Royal Society of Chemistry 2019 |
Click here to see how this site uses Cookies. View our privacy policy here.