DOI:
10.1039/C9RA07348E
(Paper)
RSC Adv., 2019,
9, 36664-36669
Air-assisted ionic liquid dispersive liquid–liquid microextraction based on solidification of the aqueous phase for the determination of triazole fungicides in water samples by high-performance liquid chromatography†
Received
12th September 2019
, Accepted 3rd November 2019
First published on 11th November 2019
Abstract
A simple, rapid, and environmentally friendly approach was introduced to determine triazole fungicides in water samples by air-assisted ionic liquid dispersive liquid–liquid microextraction based on solidification of the aqueous phase using high-performance liquid chromatography-diode array detection. Ionic liquid was applied as the extraction solvent rather than a high-toxicity extraction solvent. The air-assisted dispersion method induced a trace amount of the ionic liquid to disperse as small droplets in the water sample, which significantly increased the contact area between the organic phase and the aqueous phase for the rapid transfer of target fungicides without using a dispersion solvent or auxiliary extraction devices. The solidification of the aqueous phase facilitated the collection of extraction solvent. The type of extraction solvent, the volume ratio of the extraction solvent to the water sample, the number of extraction cycles, the addition of NaCl, and pH values were evaluated. The recoveries were 72.65–100.13% with a relative standard deviation of 0.92% to 5.99%. The limits of quantification varied from 0.65 ng mL−1 to 1.83 ng mL−1. This approach can be used to determine fungicides in ground, river, and lake water samples.
1. Introduction
Pesticides primarily serve to boost agricultural productivity and secure crop production.1,2 However, pesticides are frequently detected in aquatic ecosystems. Pesticide residues even at trace levels severely break down the environment, posing a serious danger to the biological integrity of global water resources.3 Triazole fungicides are produced globally in a large quantity that accounts for about 21% of the global fungicide market value.4 The biosynthesis of fungal ergosterol was inhibited by triazole fungicides to effectively protect various crops against fungal infections.5,6 On the basis of environmental assessments, the exposure risk of triazole fungicides tends to be greater for freshwater fish, invertebrates, birds, and mammals, depending on the application pattern and rate.7 They exert estrogenic effects by interfering with estrogen receptors in mammals.8 As a probable human carcinogen, it also induces apoptosis of human trophoblast cells.5
Fast and accurate analysis of low-concentration of pesticides is challenging. Selecting an appropriate sample pretreatment method is just as important as the analytical instrumentation.9 Thus far, the pretreatment method liquid–liquid extraction (LLE) is frequently involved. The disadvantages of the pretreatment method include time-consuming methods, high cost, and the necessity for environmentally unfriendly solvents.10 Thus, significant endeavors have been made to investigate facile, quick, accurate, and user-friendly sample preparation techniques for pesticides.11
In 2006, Rezaee et al. introduced dispersive liquid–liquid microextraction (DLLME) to extract organic analytes from aqueous matrices.12 The method attracted a lot of interest from scientists who had been working in analytical science since the introduction. The method is built on ternary solvent components (aqueous phase, extraction solvent phase, and dispersive solvent phase). The formation of cloudy solution of fine organic droplets promotes the distribution of analytes from the water to extraction solvent, and thus an efficient extraction is achieved.13 This method has been applied in not only chemistry but also genetics and molecular biology, environmental science, chemical engineering, pharmaceutics, and many other fields.14
DLLME requires defined conditions, among which the most crucial is tantamount to select an appropriate extraction and dispersive solvent. One challenge of modern analytical chemistry is tantamount to develop methods that reduce or even eliminate the use of hazardous materials.14 Therefore, one problem of DLLME lies in selecting an extraction solvent that complies the requirements of green chemistry. Ionic liquid (IL), the extraction solvent selected in this study, is liquid salt over a wide temperature range, including room temperature. Its advantages include having lower vapor pressure, and being more environmentally friendly than organic solvents.15 Due to negligible vapor pressure, it is assumed that IL is not involved in air pollution. Traditional DLLME procedures related to the use of toxic organic solvents—for example, tetrachloromethane, chloroform, and toluene—generally are hazardous to organisms and the environment. IL has been widely used in areas of chemistry, environmental, agricultural, and biological sciences, and many others. IL has been applied to extract metals, drugs, pesticides, veterinary drugs, UV filters, etc14,16. IL exhibits low toxicity compared with traditional solvents,17,18 because it results from the interaction of cations and anions and does not disseminate harmful waste products.19 IL is superior in the sample pretreatment and becomes a new type of solvents used for microextraction.14 Water-immiscible ILs were selected as extraction solvent in the work in order to reduce the risk of migration to the aquatic environment. Imidazolium ILs, including 1-butyl-3-methylimidazolium hexafluorophosphate ([BMIM]PF6), 1-hexyl-3-methylimidazolium hexafluorophosphate ([HMIM]PF6), and 1-octyl-3-methylimidazolium hexafluorophosphate ([OMIM]PF6) and the like, have spurred widespread interest in the field of microextraction.
Another problem associated with DLLME is the requirements of dispersive solvent, which is used to generate the cloudy solution to enhance the interaction between analytes and extraction solvent and improve extraction efficiency.14 Utilizing disperser solvent, however, increases the addition of organic solvents throughout the extraction process and affects the distribution of analytes into the organic solvent.20 Recently, improved DLLME techniques have been applied to overcome this limitation and exclude the use of dispersive solvents, including ultrasound-assisted DLLME,21 vortex-assisted DLLME,22 and microwave-assisted DLLME.23 However, these auxiliary methods commonly need auxiliary equipment and long extraction time. At present, using an air-assisted (AA) method in the DLLME appears to be a better solution. In AA-DLLME, the extraction solvent disperses in aqueous solution with the help of air bubbles,24,25 in which the sample solution and extraction solvent are simultaneously aspirated and pumped into a centrifugation tube several times. Formed extraction solvent droplets may increase the transfer of the analytes into the extraction solvent to achieve satisfactory extraction recovery in a short time. Therefore, the use of AA-DLLME should raise concerns to achieve efficient extraction of different types of analytes.
The collection of extraction solvent is another great difficulty in the extraction process. In order to solve the problem, DLLME-SFO (Dispersive liquid–liquid microextraction based on solidification of floating organic droplet) was proposed.26 The extraction solvent with low density and the high freezing point was solidified through the ice bath. The floating extraction solvent was collected easily, minimizing the loss of the extraction solvent. Hence, DLLME-SFO has become one of the most interesting sample preparation techniques developed in recent years. However, few extraction solvents such as 1-undecanol, 1-dodecanol, n-hexadecane, cyclohexanol can be applied in DLLME-SFO.27 To the best of our knowledge, the application of the technique combined with ionic liquid has few reports so far. If there is a huge difference of freezing point between the ionic liquid and water, either organic or aqueous phase could be solidified by low temperature freezer, facilitating the phase separation and the collection of the extraction solvent.
In the study, a novel AA-IL-DLLME-SA (air-assisted ionic liquid dispersive liquid–liquid microextraction based on solidification of aqueous phase) technique was developed that takes advantage of the green ionic liquid, air-assisted dispersion methods, and solidification of aqueous phase to readily extract triazole fungicides in water samples. The type of extraction solvent, the volume ratio of extraction solvent to water sample, the number of extraction cycles, the addition of NaCl, and pH values were investigated and evaluated. Furthermore, the method was assessed in ground, river, and lake water samples.
2. Experimental
2.1 Chemicals and reagents
Strobilurin fungicides myclobutanil (98%), tebuconazole (98%), and epoxiconazole (98%) came from the Institute for the Control of Agrochemicals (Beijing, China). ILs [BMIM]PF6, [HMIM]PF6, and [OMIM]PF6 were brought from Shanghai Aladdin Biochemical Technology Co., Ltd. (Shanghai, China). Methanol was purchased from Honeywell (Morris Plains, NJ, USA). The reagents NaCl, NaOH, and HCl were brought from Sinopharm Chemical Reagent (Shanghai, China).
2.2 Instrumentation
Agilent 1260 high-performance liquid chromatography (HPLC) with an auto-injector and a diode array detector came from Agilent Technologies Inc. (Santa Clara, CA, USA). Chromatographic separations were achieved on the Agilent Eclipse Plus C18 column (250 × 4.6 mm, 5 μm). The injection volume was 20 μL. The mobile phase was methanol + water (4 + 1 by volume) at a flow rate of 0.5 mL min−1. The column temperature was held at 20 °C. The detection wavelength was 220 nm. The HPLC chromatogram was presented in Fig. S1.†
2.3 AA-IL-DLLME-SA procedure
The 5 mL of the water sample was transferred to a 15 mL centrifuge tube with a conical bottom. After adding 50 mg of NaCl and 125 μL of IL, the mixture was sucked into a 10 mL syringe. Air bubbles were generated because of the existence of interstice between the needle and syringe. Then the mixture was injected into the centrifuge tube with a large number of bubbles regenerated. Air bubbles promoted the transfer of target compounds into the IL. After five suction–injection cycles, the mixture was centrifuged at 4000 rpm for 3 min for phase separation, and the IL settled onto the conical bottom of the tube. Most of the water was removed and the centrifuge tube was put into low temperature freezer (−20 °C). Then the upper aqueous phase was solidified and the IL phase was recovered completely, dissolved in 50 μL of methanol before HPLC analysis. The schematic diagram of the AA-IL-DLLME-SA procedure was illustrated in Fig. 1.
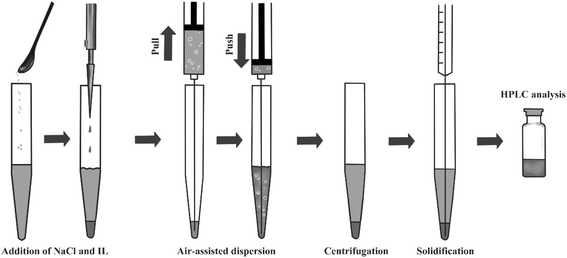 |
| Fig. 1 Schematic diagram of the AA-IL-DLLME-SA procedure. | |
3. Results and discussion
3.1 Optimization of extraction conditions
To identify suitable extraction parameters, experiments were carried out after adding the standards of triazole fungicides (myclobutanil, tebuconazole, and epoxiconazole) with the concentration of 100 ng mL−1. The type of extraction solvent, the volume ratio of extraction solvent to water sample, the number of extraction cycles, the addition of NaCl, and pH values were investigated and evaluated.
3.1.1 Effect of the type of extraction solvent. In the DLLME, the choice of an appropriate extraction solvent should be examined first. The extraction solvent is favorable with good chromatographic properties, low water solubility, high extraction capacity and distribution coefficient.28 In this experiment, the qualified imidazolium ILs [BMIM]PF6 (melting point, 6.5 °C), [HMIM]PF6 (melting point, −73.5 °C), and [OMIM]PF6 (melting point, −82.5 °C), were selected as the extraction solvent. Other invariable factors were as follows: the volume ratio of extraction solvent to water sample 125/5000, extraction cycles 5, the addition of NaCl 50 mg. The results in Fig. 2 showed that [OMIM]PF6 had better extraction efficiency than [BMIM]PF6 and [HMIM]PF6. The results may be due to the effect of the carbon chain length of the substituent in ionic liquids. Besides, the collection of [BMIM]PF6 could not be achieved by the solidification of aqueous phase. Thus, [OMIM]PF6 was selected as the extraction solvent.
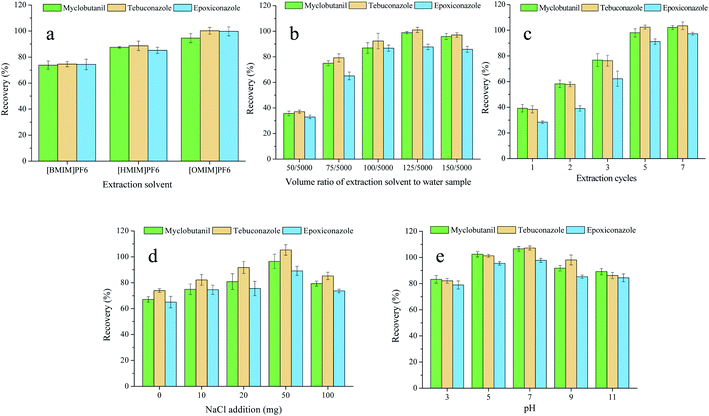 |
| Fig. 2 Investigation of appropriate conditions: effect of the type of extraction solvent (a); effect of the volume ratio of extraction solvent to water sample (b); effect of the number of extraction cycles (c); effect of the addition of NaCl (d); effect of pH values (e). | |
3.1.2 Effect of the volume ratio of extraction solvent to water sample. The volume of extraction solvent may affect the extraction efficiency and enrichment factors.28 To find the optimal volume of the extraction solvent, different volume ratios of [OMIM]PF6 to water sample were tested in the range of 50/5000 to 150/5000. Other invariable factors were as follows: the extraction solvent [OMIM]PF6, extraction cycles 5, the addition of NaCl 50 mg. As shown in Fig. 2, the recovery increased first and then tended to be stable with an increase in the ratio. The probable reason was that the target analytes could not be fully extracted by the small volume of extraction solvent, resulting in a low extraction recovery.29 A low volume of extraction solvent was desirable according to the point of view of green chemistry, therefore, the volume ratio of extraction solvent to water sample of 125/5000 was selected for further study.
3.1.3 Effect of the number of extraction cycles. In the method, the number of extractions cycles was defined as the number of repeatedly sucking extraction solvent and sample solution mixture into a 10 mL syringe and then the sample solution was injected into the centrifuge tube.13 This simple action led to dispersion of extraction solvent into the sample solution. Due to the repeated pumping treatment using a syringe, the mixed sample could be emulsified, increasing the interaction between the IL and the sample solution. The recovery is expected to be improved with the repetition of the extraction. In this paper, the effect of AA extraction cycles from one to seven was studied. Other invariable factors were as follows: the volume ratio of [OMIM]PF6 to water sample 125/5000, the addition of NaCl 50 mg. As shown in Fig. 2, when the number of cycles increased to five extraction cycles, good recovery and the relative standard deviation were achieved. With the increase of number of extraction cycles, the extraction efficiency increased because of better dispersion of extraction solvent in the sample solution. Fine droplets of extraction solvent were generated through five extraction cycles. Therefore, five extraction cycles were selected as the optimal value. Note that the step was completed in less than 30 s, indicating the effectiveness of the proposed AA-IL-DLLME-SA method.
3.1.4 Effect of the addition of NaCl. Ionic strength also may have affected extraction efficiency in DLLME. The extraction recoveries (Fig. 2) for the target analytes increased with increasing addition of NaCl and then decreased, which indicated that the amount of NaCl played an active role. This phenomenon could be explained by salting-out effects and salting-in effects.30 The addition of NaCl reduces the solubility of analytes in water samples and promotes the transfer of them to extraction solvent.13 However, the addition of NaCl could make the sample matrix denser and more viscous, leading to slower kinetics during the extraction process.31 The ion exchange process also reduces the extraction efficiency through increasing the solubility of IL in water samples. Therefore, changing ionic strength influenced the extraction performance of organic compounds because of the two opposite effects. The results in Fig. 2 shown that the recovery of triazole fungicides gradually increased and then decreased as the NaCl addition increased from 0 to 100 mg. Other invariable factors were as follows: the volume ratio of [OMIM]PF6 to water sample 125/5000, extraction cycles 5. Then, 50 mg of NaCl was considered for further study.
3.1.5 Effect of pH values. The pH of the water sample may affect the presence of existing analytes and therefore the extraction efficiency of DLLME.32 The influence of pH values (3 to 11) was evaluated with HCl or NaOH adjustment. Other invariable factors were as follows: the volume ratio of [OMIM]PF6 to water sample 125/5000, extraction cycles 5, the addition of NaCl 50 mg. When the pH values of the water sample were in the range of 3 to 11, the triazole fungicides were accessible to the IL extractant to achieve the recovery greater than 75% (Fig. 2). Thus, pH adjustment was unnecessary for the water samples.
3.2 Analytical performance of AA-IL-DLLME-SA
The analytical performance of the AA-IL-DLLME-SA method was evaluated with optimal extraction parameters. Three replicate extractions were implemented for each concentration. The linearity was evaluated using different water samples at different concentrations of triazole fungicides. Good calibration curves were obtained in the range of 10 to 1000 ng mL−1 for myclobutanil, tebuconazole, and epoxiconazole with coefficients of determination (R2) in the range of 0.995 to 0.999 (Table 1). The limits of detection and quantification (LODs and LOQs) calculated as 3 and 10 times the signal-to-noise ratio were 0.19–0.55 ng mL−1 and 0.65–1.83 ng mL−1, respectively.
Table 1 Analytical figures of merit of the proposed method
Analyte |
Sample |
Regression equation (ng mL−1) |
Coefficients of determination (R2) |
LOD (ng mL−1) |
LOQ (ng mL−1) |
Myclobutanil |
Ground water |
y = 4.124x + 85.18 |
0.999 |
0.26 |
0.85 |
River water |
y = 3.503x + 72.92 |
0.998 |
0.46 |
1.53 |
Lake water |
y = 3.001x + 146.41 |
0.998 |
0.25 |
0.85 |
Tebuconazole |
Ground water |
y = 3.984x + 90.82 |
0.998 |
0.20 |
0.66 |
River water |
y = 3.852x + 68.64 |
0.998 |
0.38 |
1.26 |
Lake water |
y = 3.252x + 188.12 |
0.996 |
0.19 |
0.65 |
Epoxiconazole |
Ground water |
y = 2.912x + 71.60 |
0.999 |
0.36 |
1.19 |
River water |
y = 2.653x + 79.41 |
0.998 |
0.55 |
1.83 |
Lake water |
y = 2.135x + 144.01 |
0.995 |
0.32 |
1.08 |
3.3 Analysis of real samples
To evaluate the applicability and accuracy of the AA-IL-DLLME-SA method, three actual water samples (ground, river, and lake water) were applied. The samples were extracted by AA-IL-DLLME-SA method and subsequently detected by HPLC to analyze the content of myclobutanil, tebuconazole, and epoxiconazole,33 indicating no residues in real samples. Thus, water samples spiked at concentrations of 10, 100, and 1000 ng mL−1 were extracted under optimal conditions to investigate the accuracy of the method. The results in Table 2 shown that the recovery of the three fungicides was 72.65–100.13% in the water samples with a relative standard deviation (RSD) of 0.92–5.99%. These results indicated that AA-IL-DLLME-SA had good applicability and accuracy.
Table 2 Analytical performance of the proposed method for real samples
Analyte |
Spiked level (ng mL−1) |
Ground water |
River water |
Lake water |
Recovery (%) |
RSD (%) |
Recovery (%) |
RSD (%) |
Recovery (%) |
RSD (%) |
Myclobutanil |
1000 |
82.01 |
2.42 |
79.46 |
2.49 |
90.63 |
1.68 |
100 |
93.59 |
1.07 |
77.34 |
1.65 |
75.52 |
1.03 |
10 |
95.26 |
2.41 |
90.46 |
3.29 |
93.93 |
2.45 |
Tebuconazole |
1000 |
91.67 |
2.52 |
88.50 |
5.99 |
98.34 |
1.85 |
100 |
100.13 |
0.92 |
79.80 |
2.57 |
76.50 |
2.57 |
10 |
93.71 |
4.26 |
90.09 |
3.34 |
95.13 |
3.39 |
Epoxiconazole |
1000 |
87.33 |
3.71 |
86.50 |
2.57 |
95.78 |
4.57 |
100 |
77.00 |
3.43 |
72.65 |
2.36 |
78.50 |
2.57 |
10 |
74.84 |
2.25 |
89.87 |
4.61 |
82.64 |
3.04 |
3.4 Comparison with other techniques
The analytical performance for determining triazole fungicides in the water samples was compared in Table 3, including solvent consumption, extraction time, recovery, and LOD. The reported methods were likely to consume more extraction solvent than the proposed AA-IL-DLLME-SA method. In addition, the toxicity of IL used in this study was lower than that of toluene, tetrachloroethane, and acetonitrile solvent, which met the requirements of green chemistry.14 The reduction in extraction time was another notable feature of this experiment. The extraction time was less than 30 s, which saved the pretreatment time and enhanced timeliness. Furthermore, the proposed method was easy to operate, which achieved comparable recovery and LOD without additional instruments (water bath heater,34 ice bath,35 tablet press machine,36 stirrer,37 and vortex agitator38). Compared with the other method based on ILs, this method was a fast disperser solvent-free approach and the ILs were simply collected after solidification of aqueous phase. In summary, the comparison results showed that the study had the characteristics of simple operation, rapidity, reduced organic solvent consumption, and environmental friendliness.
Table 3 Comparison of the AA-IL-DLLME-SA method with others
Method |
Solvent consumption |
Extraction time (min) |
Recovery (%) |
LOD (ng mL−1) |
Ref. |
Cloud point extraction. DLLME based on solidification of floating organic droplet. Tablet-effervescence-assisted dissolved carbon flotation. Stir bar sorptive extraction with DLLME. Vortex-assisted DLLME. |
CPEa-HPLC-UV |
Polyethylene glycol (400 mg) |
10 |
82.0–96.0 |
0.01–0.04 |
34 |
DLLME-SFOb-HPLC-DAD |
Methanol (200 μL), dodecanol (12 μL) |
1 |
84.8–110.2 |
0.06–0.10 |
35 |
TEA-DCFc-GC-MS |
Toluene (70 μL) |
15 |
82.5–112.9 |
0.15–0.26 |
36 |
SBSE-DLLMEd-GC-FID |
Methanol (1000 μL), tetrachloroethane (25 μL) |
30 |
71.0–116.0 |
0.53–24.00 |
37 |
VA-DLLMEe-HPLC-PDA |
Acetonitrile (250 μL), ionic liquid (70 μL) |
2 |
71.0–104.5 |
0.40–6.70 |
38 |
AA-IL-DLLME-SA-HPLC-DAD |
Ionic liquid (125 μL) |
<0.5 |
72.7–100.1 |
0.19–0.55 |
This study |
4. Conclusions
In this paper, the AA-IL-DLLME-SA method was introduced that took advantage of AA methods, IL, solidification of aqueous phase to extract, collect, and detect triazole fungicides in water samples by HPLC. Extraction solvent IL was evenly dispersed into water samples in the form of droplets within 30 s for extraction by repeatedly pumping the mixture into a centrifuge tube. The advantages of the method used in this study were the ability to avoid the use of high-toxicity extraction solvent and any dispersive solvent, facilitate the collection of extraction solvent. Additional extraction instruments (stirrer, vortex agitator, and ultrasonic mixer) were not applied. The developed method showed good extraction efficiency, linearity, accuracy, and applicability. Accordingly, AA-IL-DLLME-SA is a simple, rapid, environmentally friendly, and effective alternative for the analysis of triazole fungicides in water samples.
Conflict of interest
There are no conflicts of interest to declare.
Acknowledgements
This work was supported by the Shanxi Agricultural University Science and Technology Innovation [grant number 2017YJ35]; Project of Shanxi Key R & D Program of China [grant number 201803D221009-5]; Transformation of Scientific and Technological Achievements Programs of Higher Education Institutions in Shanxi.
References
- E. A. Songa and J. O. Okonkwo, Talanta, 2016, 155, 289–304 CrossRef CAS.
- P. Kumar, K.-H. Kim and A. Deep, Biosens. Bioelectron., 2015, 70, 469–481 CrossRef CAS.
- X. Zhang, Y. Luo and K. S. Goh, Environ. Pollut., 2018, 234, 48–58 CrossRef CAS.
- H. Hamdi, I. Ben Salem, Y. Ben Othmène, E. Annabi and S. Abid-Essefi, Pestic. Biochem. Physiol., 2018, 148, 62–67 CrossRef CAS.
- J. Zhou, J. Zhang, F. Li and J. Liu, J. Hazard. Mater., 2016, 308, 294–302 CrossRef CAS.
- N. Li, L. Deng, J. Li, Z. Wang, Y. Han and C. Liu, Pestic. Biochem. Physiol., 2018, 147, 102–109 CrossRef CAS.
- L. Chen, R. Li, J. Diao, Z. tian, S. Di, W. Zhang, C. Cheng and Z. Zhou, Ecotoxicol. Environ. Saf., 2017, 145, 623–629 CrossRef CAS.
- X. Lv, L. Pan, J. Wang, L. Lu, W. Yan, Y. Zhu, Y. Xu, M. Guo and S. Zhuang, Environ. Pollut., 2017, 222, 504–512 CrossRef CAS.
- M. Sajid, Trends Anal. Chem., 2018, 106, 169–182 CrossRef CAS.
- X. Wang, M. Gao, J. Gao, X. Wang, M. Ma and H. Wang, J. Chromatogr. B: Anal. Technol. Biomed. Life Sci., 2018, 1092, 19–28 CrossRef CAS.
- X. Yan, H. Li and X. Su, Trends Anal. Chem., 2018, 103, 1–20 CrossRef CAS.
- M. Rezaee, Y. Assadi, M.-R. Milani Hosseini, E. Aghaee, F. Ahmadi and S. Berijani, J. Chromatogr. A, 2006, 1116, 1–9 CrossRef CAS PubMed.
- V. Ferrone, R. Cotellese, M. Carlucci, L. Di Marco and G. Carlucci, J. Pharm. Biomed. Anal., 2018, 151, 266–273 CrossRef CAS.
- I. Rykowska, J. Ziemblińska and I. Nowak, J. Mol. Liq., 2018, 259, 319–339 CrossRef CAS.
- S. Sadeghi and Z. Nasehi, Spectrochim. Acta, Part A, 2018, 201, 134–142 CrossRef CAS.
- M. J. Trujillo-Rodríguez, P. Rocío-Bautista, V. Pino and A. M. Afonso, TrAC, Trends Anal. Chem., 2013, 51, 87–106 CrossRef.
- A. Mohammadi, R. Tavakoli, M. Kamankesh, H. Rashedi, A. Attaran and M. Delavar, Anal. Chim. Acta, 2013, 804, 104–110 CrossRef CAS.
- L. Ruiz-Aceituno, M. L. Sanz and L. Ramos, TrAC, Trends Anal. Chem., 2013, 43, 121–145 CrossRef CAS.
- M. Tuzen and O. Z. Pekiner, Food Chem., 2015, 188, 619–624 CrossRef CAS.
- E. Yiantzi, E. Psillakis, K. Tyrovola and N. Kalogerakis, Talanta, 2010, 80, 2057–2062 CrossRef CAS.
- C. Klinglmayr, K. Nöbauer, E. Razzazi-Fazeli and M. Cichna-Markl, J. Chromatogr. B: Anal. Technol. Biomed. Life Sci., 2010, 878, 187–193 CrossRef CAS.
- A. Gure, F. J. Lara, A. M. García-Campaña, N. Megersa and M. del Olmo-Iruela, Food Chem., 2015, 170, 348–353 CrossRef CAS.
- M. Kamankesh, A. Mohammadi, H. Hosseini and Z. Modarres Tehrani, Meat Sci., 2015, 103, 61–67 CrossRef CAS.
- N. Lamei, M. Ezoddin and K. Abdi, Talanta, 2017, 165, 176–181 CrossRef CAS.
- Z. Luo, J. Lu, H. Li, Y. Tu, Y. Wan and Z. Yang, Food Chem., 2018, 260, 174–182 CrossRef CAS.
- M.-I. Leong and S.-D. Huang, J. Chromatogr. A, 2008, 1211, 8–12 CrossRef CAS.
- F. R. Mansour and N. D. Danielson, Talanta, 2017, 170, 22–35 CrossRef CAS PubMed.
- X. You, X. Chen, F. Liu, F. Hou and Y. Li, Food Chem., 2018, 239, 354–359 CrossRef CAS.
- D. Yang, Y. Wang, J. Peng, C. Xun and Y. Yang, Ecotoxicol. Environ. Saf., 2019, 178, 130–136 CrossRef CAS.
- P. Zhou, K. Chen, M. Gao, J. Qu, Z. Zhang, R. A. Dahlgren, Y. Li, W. Liu, H. Huang and X. Wang, Food Chem., 2018, 268, 468–475 CrossRef CAS PubMed.
- Z. Huang, P. E. Chua and H. K. Lee, J. Chromatogr. A, 2015, 1399, 8–17 CrossRef CAS.
- X. Jing, L. Yang, W. Zhao, F. Wang, Z. Chen, L. Ma, L. Jia and X. Wang, J. Chromatogr. A, 2019, 1597, 46–53 CrossRef CAS.
- M. A. Farajzadeh, D. Djozan and R. F. Bakhtiyari, Talanta, 2010, 81, 1360–1367 CrossRef CAS.
- T. Tang, K. Qian, T. Shi, F. Wang, J. Li and Y. Cao, Anal. Chim. Acta, 2010, 680, 26–31 CrossRef CAS.
- C. Wang, Q. Wu, C. Wu and Z. Wang, J. Hazard. Mater., 2011, 185, 71–76 CrossRef CAS.
- Q. Wei, Z. Song, J. Nie, H. Xia, F. Chen, Z. Li and M. Lee, J. Sep. Sci., 2016, 39, 4603–4609 CrossRef CAS.
- M. A. Farajzadeh, D. Djozan, N. Nouri, M. Bamorowat and M. S. Shalamzari, J. Sep. Sci., 2015, 33, 1816–1828 CrossRef.
- Y. Zhang, Y. Zhang, Q. Zhao, W. Chen and B. Jiao, Food Anal. Methods, 2016, 9, 596–604 CrossRef.
Footnote |
† Electronic supplementary information (ESI) available. See DOI: 10.1039/c9ra07348e |
|
This journal is © The Royal Society of Chemistry 2019 |
Click here to see how this site uses Cookies. View our privacy policy here.