DOI:
10.1039/C9SC00640K
(Edge Article)
Chem. Sci., 2019,
10, 5787-5792
Cyano-borrowing reaction: nickel-catalyzed direct conversion of cyanohydrins and aldehydes/ketones to β-cyano ketone†
Received
5th February 2019
, Accepted 4th May 2019
First published on 6th May 2019
Abstract
A direct nickel-catalyzed, high atom- and step-economical reaction of cyanohydrins with aldehydes or ketones via an unprecedented “cyano-borrowing reaction” has been developed. Cleavage of the C–CN bond of cyanohydrins followed by aldol condensation and conjugate addition of cyanide to α,β-unsaturated ketones proceeded to deliver a range of racemic β-cyano ketones with good to high yields. The practical procedure with the use of a commercial and less-toxic CN source bodes well for wide application of this protocol.
Introduction
Nitriles are important synthesis intermediates in transformation1 and are key components in various natural products, medicinal pharmacophores and drugs,2 and in organic synthesis, the cyano group is equivalent to an amine or carbonyl group. The catalytic addition of the cyano group to the C
C bond has been established as one of the most direct pathways for the synthesis of nitriles.3,4 Among the important nitriles, β-cyano-ketones are commonly utilized in organic synthesis.5 One of the classical approaches to deliver these compounds is the catalyzed conjugate addition of cyanide to α,β-unsaturated carbonyl compounds (hydrocyanation procedure, Scheme 1a),6 which utilizes the highly toxic and explosive HCN gas as the cyano source. An alternative strategy is transfer hydrocyanation, which involves the commercially available, less-toxic and less-explosive cyanohydrin to deliver nitriles (Scheme 1b), but with low atom economy.7 Recently, Morandi developed a nickel-catalyzed transfer hydrocyanation reaction between alkyl nitriles and alkenes or aryl chlorides, which utilizes non-toxic alkyl nitriles as the cyanide source.8 Although the catalyzed hydrocyanation and transfer hydrocyanation reactions have been well developed,6,7 it remains an important challenge to bypass the usage of toxic HCN gas as the cyano source and overcome the issue of atom-economy. To mitigate these concerns and inspired by the atom- and step-economical procedure of β-alkylation of secondary alcohols with aldehydes via borrowing hydrogen reactions,9,10 we postulated that the cyano group could be tolerated in the reaction with a mechanism analogous to the borrowing hydrogen reactions. Guided by these considerations, we envisioned that, as shown in Scheme 1c, under the catalysis of a transition metal, there is cleavage of the C–CN bond11,12 of the cyanohydrin and delivery of the corresponding ketones and metal–cyano intermediate ([M]n–CN), followed by aldol condensation of ketones with aldehydes, and subsequent conjugate addition of cyanide to chalcones and utilization of the [M]n+1–CN as the cyano donor to deliver the desired products (Scheme 1c). This hypothesis is notable in that cyanohydrin plays a dual role both as the source of ketone and the cyanide donor with high atom- and step-economy. Herein, we report the first catalytic process of the direct transformation of cyanohydrins with aldehydes to deliver β-cyano ketones as the sole product via nickel catalyzed cyano-borrowing reactions.13
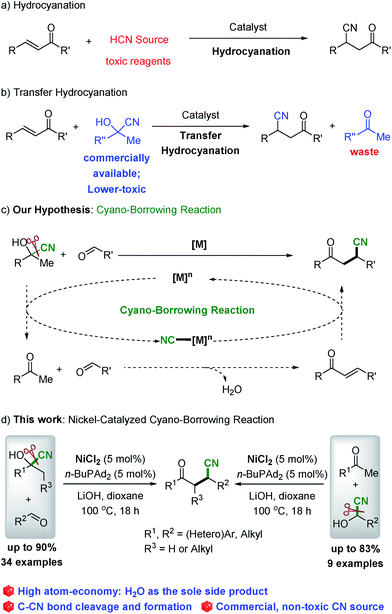 |
| Scheme 1 Development of cyano-borrowing. | |
Results and discussion
To test our hypothesis, we initiated the cyano-borrowing reaction using commercially available acetophenone cyanohydrin 1a which could be prepared from acetophenone and TMSCN and benzaldehyde 2a for the optimization of the reaction conditions. After screening an array of transition metal catalysts, we found that nickel complexes showed better performance. To our delight, a cocktail consisting of NiBr2, PPh3, and LiOH as the base in dioxane at 100 °C could deliver the desired racemic β-cyano ketone 3aa in 52% yield (Table 1, entry 1). Notably, determined by the crude 1H NMR of the reaction mixture, not even a trace of 1,2-addition products was observed.14 As shown in Table 1, we then examined an extensive array of parameters. By varying the anion of the nickel salt (i.e., NiCl2, Ni(OAc)2, Ni(OTf)2 and Ni(acac)2) as precatalysts, NiCl2 exhibited the best yield (Table 1, entries 3–5 vs. 2). The screening of different phosphines revealed that this transformation requires a bulky, electron rich ligand, and t-BuPAd2 showed the highest reactivity with 82% yield (Table 1, entry 11 vs. 2 and 6–10). Stronger bases such as NaOH and KOt-Bu show low reactivity. Meanwhile, Cs2CO3 and organic bases (i.e., DMAP and DBU) failed to provide the target products (Table 1, entries 14–16). Optimization of the solvent led to no improvement (Table 1, entries 17–19). Lowering the temperature to 80 °C gives a lower yield (Table 1, entry 20). Control experiments verified that the presence of a Ni-complex was necessary to achieve high yield in the cyano-borrowing reaction (Table 1, entry 21). No reaction occurred in the absence of LiOH (Table 1, entry 22). After the screening of the reaction parameters, we found that NiCl2 and n-BuPAd2 as the precatalyst and LiOH as the base in dioxane at 100 °C for 20 h (82% yield) were the optimal conditions.
Table 1 Screening studies of β-alkylation of cyanohydrin 1a with benzaldehyde 2aa

|
Entry |
[Ni] |
L
|
Base |
Solvent |
Yieldb (%) |
The reaction was carried out with 0.4 mmol of 1a, 0.4 mmol of 2a, 5 mol% [Ni], 10 mol% ligand (L) and 300 mol% base in 0.5 mL of solvent at 100 °C for 18 h.
Isolated yield.
The reaction was carried out at 80 °C. dppm = bis(diphenylphosphino)methane; dppe = bis(diphenylphosphino)ethane; dppp = bis(diphenylphosphino)-propane; dppb = bis(diphenylphosphino)butane; n-BuPAd2 = di(1-adamantyl)-n-butylphosphine; TBME = methyl tert-butyl ether.
|
1 |
NiBr2 |
PPh3 |
LiOH |
Dioxane |
52 |
2 |
NiCl2 |
PPh3 |
LiOH |
Dioxane |
76 |
3 |
Ni(OAc)2 |
PPh3 |
LiOH |
Dioxane |
66 |
4 |
Ni(OTf)2 |
PPh3 |
LiOH |
Dioxane |
62 |
5 |
Ni(acac)2 |
PPh3 |
LiOH |
Dioxane |
71 |
6 |
NiCl2 |
PCy3 |
LiOH |
Dioxane |
76 |
7 |
NiCl2 |
dppe |
LiOH |
Dioxane |
72 |
8 |
NiCl2 |
dppp |
LiOH |
Dioxane |
78 |
9 |
NiCl2 |
dppb |
LiOH |
Dioxane |
37 |
10 |
NiCl2 |
BINAP |
LiOH |
Dioxane |
44 |
11
|
NiCl
2
|
n-
BuPAd
2
|
LiOH
|
Dioxane
|
82
|
12 |
NiCl2 |
n-BuPAd2 |
NaOH |
Dioxane |
28 |
13 |
NiCl2 |
n-BuPAd2 |
KOt-Bu |
Dioxane |
Trace |
14 |
NiCl2 |
n-BuPAd2 |
Cs2CO3 |
Dioxane |
0 |
15 |
NiCl2 |
n-BuPAd2 |
DMAP |
Dioxane |
0 |
16 |
NiCl2 |
n-BuPAd2 |
DBU |
Dioxane |
0 |
17 |
NiCl2 |
n-BuPAd2 |
LiOH |
Toluene |
22 |
18 |
NiCl2 |
n-BuPAd2 |
LiOH |
TBME |
23 |
19 |
NiCl2 |
n-BuPAd2 |
LiOH |
THF |
72 |
20c |
NiCl2 |
n-BuPAd2 |
LiOH |
Dioxane |
59 |
21 |
— |
— |
LiOH |
Dioxane |
<10 |
22 |
NiCl2 |
n-BuPAd2 |
— |
Dioxane |
0 |
Having identified the optimized reaction conditions, we further explored the substrate scope of this reaction. Various commercially available ketone cyanohydrins were examined with benzaldehyde (2a) and the results are summarized in Scheme 2. The electron-deficient substrate with varying substituent patterns at the p-position did not dramatically influence yields (3ba–3da). Substituting the cyanohydrin with p-methyl also shows high reactivity and 72% of the desired product was obtained (Scheme 2, 3ea). However, substituting the para position with a more electron-rich functionality (i.e., p-MeO) led to diminished yields, but the desired product could be delivered with 86% yield while increasing the reaction temperature to 120 °C (Scheme 2, 3fa). Reactions of cyanohydrin with ortho- and meta-substitution on aryl groups gave excellent yields (Scheme 2, 3ga–3ja). The reaction proceeds smoothly in the case of the cyanohydrin bearing a thienyl group, affording the corresponding product 3la in 82% isolated yield. In terms of the alkyl substituents, cyanohydrin derived from butanone proceeded smoothly to afford the desired product with 40% yield (Scheme 2, 3ma). The substrate bearing a cyclopropyl group was well tolerated, leading to the cyclopropyl substituted product with 57% yield (Scheme 2, 3na). Importantly, the cyclopropyl group remains untouched, which indicates that this nickel-catalyzed protocol does not proceed via a radical pathway.
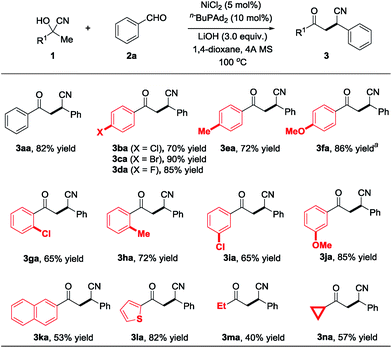 |
| Scheme 2 Reaction scope of ketone cyanohydrins. aThe reaction was carried out at 120 °C. | |
Next, various aldehydes were investigated with acetophenone cyanohydrin (1a) using the optimized reaction conditions, and representative results are summarized in Scheme 3. Benzaldehydes bearing various electron-deficient (3ab–3ad and 3ag–3ah), electron-neutral (3al and 3am) and electron-rich (3ae–3af and 3ai–3ak) substituents reacted with 1a to deliver the desired products in moderate to excellent yields (58–90%). Furthermore, the reaction is also compatible with heteroaryl rings, such as 2-furanyl (3an), 2-thiophenyl (3ao) and unprotected 3-indolyl (3ap), providing diverse β-cyano ketones in 51–77% yield. Remarkably, compared with the aryl aldehydes, the alkyl substituted aldehydes (e.g., 2-phenylacetaldehyde and cyclohexanecarbaldehyde) reacted with 1a to afford the corresponding products 3aq and 3ar in 64% and 80% yields, respectively. The aldehyde containing a sulfur atom is also tolerated under the nickel-catalyzed protocol and delivers the desired product 3as with a slightly lower yield. To demonstrate the practicality and scalability of our protocol, we proceeded to carry out a gram-scale reaction with 5.0 mmol 2i reacted with 10.0 mmol 1a catalyzed by 5 mmol% of NiCl2, affording 1.12 g 3ai in 85% yield, suggesting that this procedure is quite reliable and practically applicable.
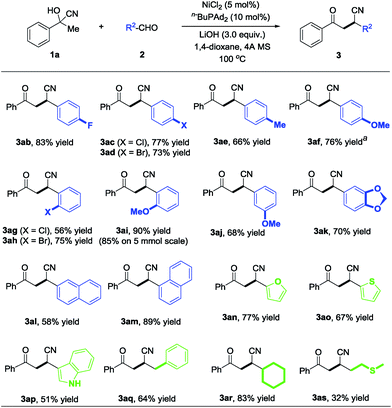 |
| Scheme 3 Reaction scope of aldehydes. aThe reaction was carried out at 120 °C. | |
Inspired by the success with the nickel-catalyzed cyano-borrowing reaction of cyanohydrins derived from ketones 1 with aldehydes, we further demonstrate the cyano-borrowing protocol with more challenging substrates such as aldehyde cyanohydrin 5. To our delight, benzaldehyde cyanohydrin 5a reacted with acetophenone 4a smoothly under standard conditions, yielding the corresponding product 3aa with full conversion and 83% isolated yield. To indicate the generality of this protocol, we then examined the scope of benzaldehyde cyanohydrins and ketones. In addition to the phenyl group, it was found that substrates bearing electron-rich or electron-deficient substituents on the benzene ring were well tolerated to give β-cyano ketones in moderate to good yields (3ba, 3ea, 3ad and 3af in Scheme 4). Moreover, heteroaryl and alkyl substituents also participated in this protocol very well (Scheme 4, 3la, 3ma, 3ao and 3ar).
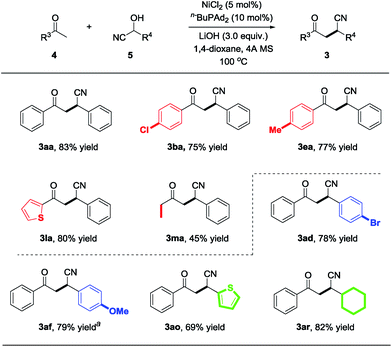 |
| Scheme 4 Examples of aldehyde cyanohydrin with ketone. aThe reaction was carried out at 120 °C. | |
To illustrate the scope and limitations of the new transformation, challenging substrates beyond methyl ketones were further examined, shown in Scheme 5. The propiophenone cyanohydrin 6 was selected to react with benzaldehyde 2a under standard reaction conditions, and the desired product 8 was obtained in 31% isolated yield and with high diastereoselectivity (dr > 10
:
1) (Scheme 5a, left). Furthermore, we also examined benzaldehyde cyanohydrin 5a and propiophenone 7, with a nickel catalyst, and the corresponding product 8 was obtained in 25% yield (Scheme 5a, right). Notably, the bioactive ketone, epiandrosterone was tested in this reaction with the partner of benzaldehyde cyanohydrin 5a, and the corresponding product 10 was delivered in 57% yield with 5
:
1 dr (Scheme 5b), which shows the potential of this nickel-catalyzed cyano borrowing process for the selective modification of bioactive ketones.
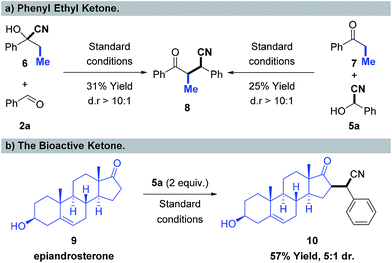 |
| Scheme 5 Cyano-borrowing beyond methyl ketones. | |
To shed light on the mechanism for the nickel-catalyzed cyano-borrowing protocol, a series of control experiments were conducted. As shown in Scheme 6, acetophenone cyanohydrin 1a reacted smoothly with chalcone 11 and give the corresponding product 3aa in excellent yield, which indicated that cyanohydrin is the source of the cyano group in transfer hydrocyanation under standard reaction conditions. Benzaldehyde cyanohydrin 5a could also react with chalcone 11 efficiently, delivering 3aa with 89% yield, and the hydrogen-borrowing product 12 was not observed, showing that cleavage of the C–CN bond is easier than that of the C–H bond in cyanohydrins. Meanwhile, in the crossover reaction of 1a, 2e and 11 under standard conditions, we got the corresponding products 3aa and 3ae with the ratio of 1.05
:
1, which shows that the cyano group from the cleavage of the C–CN bond of cyanohydrin was a free anion in this nickel-catalyzed protocol and has the same opportunity to conjugate to each chalcone. Together, these experimental results support our hypothesis on nickel-catalyzed step- and atom-economical cyano-borrowing reaction of cyanohydrin with aldehydes or ketones (Scheme 1c) (for more details of the mechanism studies, please see the ESI†).
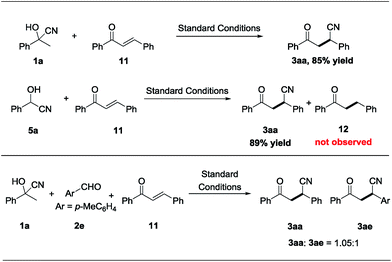 |
| Scheme 6 Mechanism studies. | |
Experimental
General procedure
Method A.
To a vial equipped with a dried stir bar was added aldehydes (0.2 mmol), ketone cyanohydrins (0.4 mmol), NiCl2 (5 mol%), n-BuPAd2 (5 mol%), LiOH (0.6 mmol), 100 mg 4Å MS and anhydrous dioxane (1 mL) in a glovebox. The reaction mixture was taken outside the glovebox and allowed to stir at room temperature for 30 min. After that, the reaction mixture was allowed to stir at 100 °C for 18 hours. The crude reaction mixture was concentrated under reduced pressure and directly purified by silica gel chromatography to give pure products.
Method B.
To a vial equipped with a dried stir bar was added ketones (0.2 mmol), aldehyde cyanohydrins (0.4 mmol), NiCl2 (5 mol%), n-BuPAd2 (5 mol%), LiOH (0.6 mmol), 100 mg 4Å MS and anhydrous dioxane (1 mL) in a glovebox. The procedure was the same as Method A.
Conclusions
In conclusion, we have developed an unprecedented nickel-catalyzed protocol for the direct conversion of cyanohydrins and aldehydes or ketones into racemic β-cyano ketones via a nickel-catalyzed cyano-borrowing reaction. A range of cyanohydrins derived from aldehydes or ketones could be tolerated and delivered products with high regioselectivity and good to excellent yields. To the best of our knowledge, catalytic conversion of cyanohydrins into β-cyano ketones by reaction with aldehydes/ketones has not been reported. Further studies of catalytic cyano-borrowing reaction of cyanohydrins are in progress in our research lab and will be reported in due course.
Conflicts of interest
There are no conflicts to declare.
Acknowledgements
We are grateful for the financial support provided by the National Natural Science Foundation of China (21801209 and 21801210), the Fundamental Research Funds for the Central Universities (SWU118117, XDJK2018C044 and SWU118028) and the Venture & Innovation Support Program for Chongqing Overseas Returnees (cx2018085).
Notes and references
-
(a) R. J. H. Gregory, Chem. Rev., 1999, 99, 3649–3682 CrossRef CAS PubMed;
(b) D. Enders and J. P. Shilvock, Chem. Soc. Rev., 2000, 29, 359–373 RSC;
(c) M.-X. Wang, Top. Catal., 2005, 35, 117–130 CrossRef CAS;
(d)
M. B. Smith, March's Advanced Organic Chemistry: Reactions, Mechanisms, and Structure, Wiley-Interscience, 6th edn, 2007 Search PubMed;
(e) R. Wang and J. R. Falck, Catal. Rev.: Sci. Eng., 2014, 56, 288–331 CrossRef CAS PubMed;
(f) M. Tobisu, R. Nakamura, Y. Kita and N. Chatani, J. Am. Chem. Soc., 2009, 131, 3174–3175 CrossRef CAS PubMed;
(g) G. A. Chotana, M. A. Rak and M. R. Smith, J. Am. Chem. Soc., 2005, 127, 10539–10544 CrossRef CAS PubMed;
(h) T. Patra, S. Agasti, A. Modak and D. Maiti, Chem. Commun., 2013, 49, 8362–8364 RSC;
(i) T. Patra, S. Agasti, Akanksha and D. Maiti, Chem. Commun., 2013, 49, 69–71 RSC;
(j) M. Tobisu, H. Kinuta, Y. Kita, E. Rémond and N. Chatani, J. Am. Chem. Soc., 2012, 134, 115–118 CrossRef CAS PubMed;
(k) M. Tobisu, Y. Kita and N. Chatani, J. Am. Chem. Soc., 2006, 128, 8152–8153 CrossRef CAS PubMed;
(l) E. C. Keske, T. H. West and G. C. Lloyd-Jones, ACS Catal., 2018, 8932–8940 CrossRef CAS.
-
(a)
P. Pollak, G. Romeder, F. Hagedorn and H. P. Gelbke, Nitriles, Ullmann's Encyclopedia of Industrial Chemistry, Wiley-VCH, June 15, 2000 Search PubMed;
(b) J. S. Miller and J. L. Manson, Acc. Chem. Res., 2001, 34, 563–570 CrossRef CAS PubMed;
(c)
A. Kleemann, J. Engel, B. Kutscher and D. Reichert, Pharmaceutical substances: Syntheses, Patents, Applications of the most relevant APIs, Georg Thieme Verlag, Stuttgart, New York, 2001 Search PubMed;
(d) L. J. Goujon, A. Khaldi, A. Maziz, C. Plesse, G. T. M. Nguyen, P.-H. Aubert, F. Vidal, C. Chevrot and D. Teyssié, Macromolecules, 2011, 44, 9683–9691 CrossRef CAS;
(e) F. F. Fleming, Nat. Prod. Rep., 1999, 16, 597–606 RSC;
(f) F. F. Fleming, L. Yao, P. C. Ravikumar, L. Funk and B. C. Shook, J. Med. Chem., 2010, 53, 7902–7917 CrossRef CAS PubMed.
- For reviews, see:
(a)
T. V. Rajanbabu, Hydrocyanation of Alkenes and Alkynes, in Organic Reactions, John Wiley & Sons, Inc., Hoboken, NJ, 2011, ch. 1, vol. 75, pp. 1–74 Search PubMed;
(b) M. Beller, J. Seayad, A. Tillack and H. Jiao, Angew. Chem., Int. Ed., 2004, 43, 3368–3398 CrossRef CAS PubMed and the references therein
(c)
T. V. RajanBabu and A. L. Casalnuovo, in Comprehensive Asymmetric Catalysis, ed. E. N. Jacobsen, A. Pfaltz and H. Yamamoto, Springer, Berlin, 1999, vol. 1, pp. 367–378 Search PubMed;
(d)
J. Wilting and D. Vogt, in Handbook of C-H Transformations, ed. G. Dyker, Wiley-VCH, Weinheim, 1st edn, 2005, vol. 1, pp 87–96 Search PubMed;
(e) L. Bini, C. Müller and D. Vogt, ChemCatChem, 2010, 2, 590–608 CrossRef CAS;
(f)
P. W. N. M. van Leeuwen, in Science of Synthesis: Stereoselective Synthesis 1, ed. J. G. de Vries, Thieme, Stuttgart, 2011, pp. 409–475 Search PubMed.
- For reviews on the conjugate reactions, see:
(a)
A. Córdova, Catalytic Asymmetric Conjugate Reactions, WileyVCH, Weinheim, Germany, 2014, pp. 1–439 Search PubMed;
(b)
M. Mauduit, O. Baslé, H. Clavier, C. Crévisy and A. DenicourtNowicki, Metal-Catalyzed Asymmetric Nucleophilic Addition to Electron–Deficient Alkenes, Wiley-VCH, Weinheim, Germany, 2014, p. 189 Search PubMed;
(c)
J. L. Vicario, E. Reyes, L. Carrillo and U. Uria, Organocatalytic Asymmetric Nucleophilic Addition to Electron–Deficient Alkenes, WileyVCH, Weinheim, Germany, 2014, p. 119 Search PubMed;
(d) K. Zheng, X. Liu and X. Feng, Chem. Rev., 2018, 118, 7586–7656 CrossRef CAS PubMed.
-
(a) G. M. Sammis and E. N. Jacobsen, J. Am. Chem. Soc., 2003, 125, 4442–4443 CrossRef CAS PubMed;
(b) T. Mita, K. Sasaki, M. Kanai and M. Shibasaki, J. Am. Chem. Soc., 2005, 127, 514–515 CrossRef CAS PubMed;
(c) I. Fujimori, T. Mita, K. Maki, M. Shiro, A. Sato, S. Furusho, M. Kanai and M. Shibasaki, Tetrahedron, 2007, 63, 5820–5831 CrossRef CAS.
-
(a) G. M. Sammis, H. Danjo and E. N. Jacobsen, J. Am. Chem. Soc., 2004, 126, 9928–9929 CrossRef CAS PubMed;
(b) C. Mazet and E. N. Jacobsen, Angew. Chem., Int. Ed., 2008, 47, 1762–1765 CrossRef CAS PubMed;
(c) Y. Tanaka, M. Kanai and M. Shibasaki, J. Am. Chem. Soc., 2008, 130, 6072–6073 CrossRef CAS PubMed;
(d) Y. Tanaka, M. Kanai and M. Shibasaki, J. Am. Chem. Soc., 2010, 132, 8862–8863 CrossRef CAS PubMed;
(e) N. Kurono, N. Nii, Y. Sakaguchi, M. Uemura and T. Ohkuma, Angew. Chem., Int. Ed., 2011, 50, 5541–5544 CrossRef CAS PubMed.
-
(a) B. A. Provencher, K. J. Bartelson, Y. Liu, B. M. Foxman and L. Deng, Angew. Chem., Int. Ed., 2011, 50, 10565–10569 CrossRef CAS PubMed;
(b) J. Wang, W. Li, Y. Liu, Y. Chu, L. Lin, X. Liu and X. Feng, Org. Lett., 2010, 12, 1280–1283 CrossRef CAS PubMed;
(c) Y.-F. Wang, W. Zeng, M. Sohail, J. Guo, S. Wu and F.-X. Chen, Eur. J. Org. Chem., 2013, 2013, 4624–4633 CrossRef CAS.
-
(a) X. Fang, P. Yu and B. Morandi, Science, 2016, 351, 832–836 CrossRef CAS PubMed;
(b) P. Yu and B. Morandi, Angew. Chem., Int. Ed., 2017, 56, 15693–15697 CrossRef CAS.
- For selected reviews on the borrowing-hydrogen methodology, see:
(a) Q. Yang, Q. Wang and Z. Yu, Chem. Soc. Rev., 2015, 44, 2305–2329 RSC;
(b) M. H. S. A. Hamid, P. A. Slatford and J. M. J. Williams, Adv. Synth. Catal., 2007, 349, 1555–1575 CrossRef CAS;
(c) T. D. Nixon, M. K. Whittlesey and J. M. J. Williams, Dalton Trans., 2009, 753–762 RSC;
(d) G. E. Dobereiner and R. H. Crabtree, Chem. Rev., 2010, 110, 681–703 CrossRef CAS PubMed;
(e) G. Guillena, D. J. Ramón and M. Yus, Chem. Rev., 2010, 110, 1611–1641 CrossRef CAS PubMed;
(f) A. J. A. Watson and J. M. J. Williams, Science, 2010, 329, 635–636 CrossRef CAS PubMed;
(g) S. Bähn, S. Imm, L. Neubert, M. Zhang, H. Neumann and M. Beller, ChemCatChem, 2011, 3, 1853–1864 CrossRef;
(h) S. Pan and T. Shibata, ACS Catal., 2013, 3, 704–712 CrossRef CAS;
(i) A. Corma, J. Navas and M. J. Sabater, Chem. Rev., 2018, 118, 1410–1459 CrossRef CAS PubMed;
(j) C. Gunanathan and D. Milstein, Science, 2013, 341, 1229712 CrossRef PubMed;
(k) T. Irrgang and R. Kempe, Chem. Rev., 2018, 118, 7586–7656 CrossRef PubMed. For a recent review on enantioselective borrowing hydrogen, see:
(l) A. Quintard and J. Rodriguez, Chem. Commun., 2016, 52, 10456–10473 RSC.
- For selected reviews on α-alkylation reactions using alcohols with hydrogen borrowing methodologies, see:
(a) F. Huang, Z. Liu and Z. Yu, Angew. Chem., Int. Ed., 2016, 55, 862–875 CrossRef CAS PubMed;
(b) Y. Obora, Top. Curr. Chem., 2016, 374, 11 CrossRef PubMed;
(c) Y. Obora, ACS Catal., 2014, 4, 3972–3981 CrossRef CAS; for selected examples of α-alkylation reactions using alcohols with hydrogen borrowing methodologies, see:
(d) M. Peña-López, P. Piehl, S. Elangovan, H. Neumann and M. Beller, Angew. Chem., Int. Ed., 2016, 55, 14967–14971 CrossRef PubMed;
(e) C. Seck, M. D. Mbaye, S. Coufourier, A. Lator, J. F. Lohier, A. Poater, T. R. Ward, S. Gaillard and J. L. Renaud, ChemCatChem, 2017, 9, 4410–4416 CrossRef CAS;
(f) F. Freitag, T. Irrgang and R. Kempe, Chem.–Eur. J., 2017, 23, 12110–12113 CrossRef CAS PubMed;
(g) G. Zhang, J. Wu, H. Zeng, S. Zhang, Z. Yin and S. Zheng, Org. Lett., 2017, 19, 1080–1083 CrossRef CAS PubMed;
(h) B. C. Roy, S. Debnath, K. Chakrabarti, B. Paul, M. Maji and S. Kundu, Org. Chem. Front., 2018, 5, 1008–1018 RSC.
- F. Chen, T. Wang and N. Jiao, Chem. Rev., 2014, 114, 8613–8661 CrossRef CAS PubMed.
- For selected examples of acetone cyanohydrin in hydrocyanation addition reactions, see:
(a) A. Siby, O. Loreau and F. Taran, Synthesis, 2009, 2365–2370 CAS;
(b) M. de Greef and B. Breit, Angew. Chem., Int. Ed., 2009, 48, 551–554 CrossRef CAS PubMed;
(c) A. Falk, A.-L. Göderz and H.-G. Schmalz, Angew. Chem., Int. Ed., 2013, 52, 1576–1580 CrossRef CAS PubMed;
(d) S. Arai, Y. Amako, X. Yang and A. Nishida, Angew. Chem., Int. Ed., 2013, 52, 8147–8150 CrossRef CAS PubMed;
(e) K. Nemoto, T. Nagafuchi, K.-i. Tominaga and K. Sato, Tetrahedron Lett., 2016, 57, 3199–3203 CrossRef CAS;
(f) F. Ye, J. Chen and T. Ritter, J. Am. Chem. Soc., 2017, 139, 7184–7187 CrossRef CAS PubMed.
-
(a) B. N. Bhawal and B. Morandi, ACS Catal., 2016, 6, 7528–7535 CrossRef CAS;
(b) B. N. Bhawal and B. Morandi, Chem.–Eur. J., 2017, 23, 12004–12013 CrossRef CAS PubMed.
- For selected examples of 1,2-addition of cyanide to chalcones, see:
(a) D. E. Fuerst and E. N. Jacobsen, J. Am. Chem. Soc., 2005, 127, 8964–8965 CrossRef CAS PubMed;
(b) X. Liu, B. Qin, X. Zhou, B. He and X. Feng, J. Am. Chem. Soc., 2005, 127, 12224–12225 CrossRef CAS PubMed;
(c) Q.-W. Yu, L.-P. Wu, T.-C. Kang, J. Xie, F. Sha and X.-Y. Wu, Eur. J. Org. Chem., 2018, 2018, 3992–3996 CrossRef CAS;
(d) X.-P. Zeng, Z.-Y. Cao, X. Wang, L. Chen, F. Zhou, F. Zhu, C.-H. Wang and J. Zhou, J. Am. Chem. Soc., 2016, 138, 416–425 CrossRef CAS PubMed.
Footnote |
† Electronic supplementary information (ESI) available. See DOI: 10.1039/c9sc00640k |
|
This journal is © The Royal Society of Chemistry 2019 |
Click here to see how this site uses Cookies. View our privacy policy here.