DOI:
10.1039/C9BM01422E
(Paper)
Biomater. Sci., 2020,
8, 250-255
Porous ZnO modified silk sutures with dual light defined antibacterial, healing promotion and controlled self-degradation capabilities†
Received
4th September 2019
, Accepted 9th October 2019
First published on 15th October 2019
Abstract
Current sutures have disadvantages such as poor antibacterial activities, low healing effects, and a lack of self-degradation ability. To solve these problems, here a biocompatible and dual light (yellow and NIR light) responsive porous ZnO (PZ) was synthesized to modify silk thread to improve the healing rate, antibacterial activities and controlled self-degradation speed simultaneously. The prepared silk thread was characterized by using scanning electron microscopy (SEM) and X-ray diffractometry (XRD). Besides, the antibacterial activity, degradation, dual light responsive capability and cytocompatibility of the sample were evaluated. The obtained data strongly encourage the application of this silk thread for wound treatment. Furthermore, in vivo evaluation in mice revealed that the silk thread reduced surgical-site infection and enhanced wound healing. Therefore, this silk thread shows potential for application in wound treatment clinically.
Introduction
In recent years, due to its impressive bio-compatibility, low inflammatory response and high processability,1 silk-modified biomaterials have been used for regeneration of various tissues,2–6 especially in the area of suturing.7 However, there are still some shortcomings in the traditional silk thread: the antibacterial performance is not strong, the healing effect is insufficient and the degradation ability in the body is limited. In response to these clinical problems, in recent years, a large number of research studies have been conducted on the modification of silk thread for clinical use.8–10 Silk fibroin thread was impregnated with Antheraea assama for controlled antibiotic-releasing sutures for preventing bacterial infection.11 The characteristics of silkworm silk fibroin were also improved by RGD peptide-fused recombinant mussel adhesive protein to accelerate bio-degradation in the body.12 However, in practical applications, because the requirement of degradation time is complex, it is not conducive to meet the actual needs of each patient. In theory, a desirable suture material should have the following advantages: an excellent antibacterial effect, accelerated tissue healing ability, and flexible degradation time, which could be defined according to different people's actual situations.
In order to meet the above requirements, we proposed the modification of porous ZnO (PZ) on the surface of silk (Fig. 1A). ZnO is a common antibacterial material with biosafety,13,14 which is also one of the few nanomaterials that have been approved by the FDA. The application of biomaterials based on ZnO in the biomedical field has attracted attention, and a large number of inspiring articles have been published.15–17 Compared with conventional ZnO, the specific nano-ZnO we synthesized here has a porous structure and a much lower energy generation gap (Fig. S1,† normal ZnO: 3.2 eV PZ: 2.2 eV), which thus exhibited different response effects to yellow or NIR light. In addition, it is worth mentioning that the yellow light source used in our research is not the conventional yellow LED light (a combination of blue light and yellow phosphor), but a “pure” yellow LED chip that does not include the blue light spectrum. In our previous experiments, this yellow light has been shown to accelerate wound healing.18 Combined with low-energy generation gap ZnO we used here, this light source can enhance the antibacterial effect and reduce wound infection simultaneously (Fig. 1B-I–III). In addition, under the excitation of NIR light, the rapid heating effect of PZ is used to achieve the controllable degradation of silk (Fig. 1B-IV), which is beneficial for the removal of silk thread after the recovery of wounds.
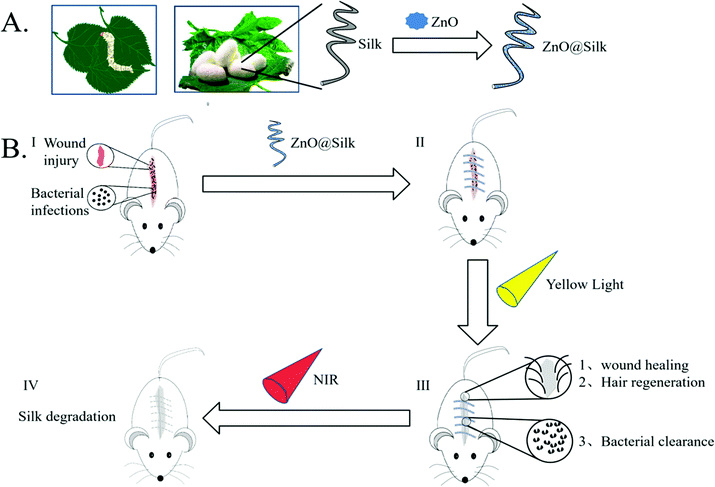 |
| Fig. 1 (A) Schematic illustration of the preparation of silk with dual light controlled capabilities. ZnO is loaded on the silk. (B) Suturing the wound with ZnO@silk (BI, BII). Yellow light stimulates ZnO to cure the wound (BII, BIII). NIR stimulates ZnO to degrade the silk (BIII, BIV). | |
Experimental
Materials
Zinc nitrate hexahydrate (Zn(NO3)2·6H2O), sodium citrate (Na3C6H5O7), hydroxypropyl methyl cellulose (HPMC), and lignin (dealkaline) were purchased from Macklin (Shanghai, China). Hexamethylene tetramine was purchased from Sinopharm Chemical Reagent Co., Ltd. Simulated body fluid (SBF) was purchased from Shanghai Hewu Biological Technology Co., Ltd. Rhodamine B was purchased from Biotop Technology Co., Ltd.
Characterization
The size and morphology of the synthesized PZ were observed by SEM (Zeiss/Sigma 300, Japan). The surface topography of the specimens was also observed by SEM. The crystalline structure of the samples was determined by X-ray diffraction (XRD) and transmission electron microscopy (TEM), respectively. The pore volume–pore size distribution was measured using a specific surface area and porosity analyzer (JW-BK132F). Enzyme-linked immunosorbent assay (SpectraMax M5 type, USA) was employed to evaluate the cytocompatibility of the silk thread treated with an HSF viable reagent. The absorbance of Rhodamine B solution was measured using an ultraviolet spectrophotometer (UV-2600, Shimadzu, China).
H&E staining was observed using a microscope.
Preparation of the sample
80 mM NH3·H2O, 50 mM Zn(NO3)2·6H2O and 25 mM HMT were dissolved in 100 mL deionized water by stirring for 10 minutes in a sealed beaker. After 15 minutes of heating in a water bath at 65 °C, 0.14 g Na3C6H5O7, 0.1 g HPMC, 0.1 g carbon, and 0.1 g lignin were added into the liquor, and the water bath was maintained at 85 °C for 10 hours. Then the mixture was washed twice with absolute ethanol and twice with water, subjected to microwave irradiation (850 W, 15 min), freeze-dried for 12 hours after pre-freezing at −80 °C, and finally the PZ powder was obtained. Silk immersed in PZ powder solution was oscillated for a period of time, and then dried at 60 °C to obtain silk loaded with PZ.
Adsorption property assay in vitro
2 mg PZ powder was placed in a certain concentration of Rhodamine B solution, and shaken at a rate of 150 rpm in a shaker to observe the color change of Rhodamine B solution after 1, 2, 3, and 24 hours, respectively. The supernatant at the different time periods was measured using the ultraviolet spectrophotometer to determine the OD value of the Rhodamine B absorption peak.
Durability assay in vitro
The silk threads loaded with PZ were divided into three groups, with three threads in each group, which were placed in SBF and immersed for 1, 3, and 7 days, respectively. The supernatant was taken and subjected to ICP-MS to measure the content of the Zn element, and thereby the rate of adhesion was calculated. The loaded silk thread was observed under SEM for the loading of PZ.
Degradation property assay in vitro
Nine portions of silk thread loaded with PZ were divided into three groups: a stroke-physiological saline solution group (SPSS), an SBF group, and an SBF + NIR group. The silk samples were numbered and weighed as the original weight A. The samples from the two groups were immersed in 5 mL SBF, one group was immersed in physiological saline, and the other groups were placed in a 37 °C incubator. The SBF + NIR group was taken out daily with NIR irradiation, and then the three groups were taken out and dried. The weight B was calculated, and the degradation rate of the sample was calculated as (A − B)/A × 100%. Thus, the in vitro degradation data for the entire experimental cycle (a week) was obtained.
Antibacterial property assay in vitro
E. coli and S. aureus were chosen to evaluate the antibacterial ability of the silk thread. E. coli and S. aureus were cultured in Luria–Bertani broth at 37 °C for 24 hours and adjusted to a concentration of 107 CFU mL−1. Then, 100 μL of bacterial stock solution was taken into 5 mL of Luria–Bertani broth, with the PZ-loaded silk, silk and control groups (nothing) incubated for 6–8 hours. After that, 100 μL of the coculture liquid was diluted to 102 CFU mL−1 and 50 μL of the diluent was used to spread on the Petri dishes which were placed in a constant temperature incubator (37 °C) for 24 hours. The antibiotic potency of the samples from the three groups was compared by the plate counting method. The antibacterial rates (R) for E. coli and S. aureus in the medium were calculated based on the following formula: R = (A − B)/A × 100%, where A is the number of bacteria in the control group and B is the number of bacteria in the silk or PZ-loaded silk group.19
Cytotoxicity assay
The cytotoxicity of the PZ modified silk was assessed by cell counting kit-8 (CCK-8) assay. We cultured human fibroblasts (HSF) in DMEM medium. The PZ modified silk was also immersed in DEEM medium, then the silk was immersed in DEEM medium for 1, 3, and 7 days, and the cells were treated with 25, 50, 100, and 200 μg mL−1 immersion solutions, respectively. When the cells were grown for 1, 3, and 7 days, the medium containing 10% CCK8 was directly placed for replacement. Incubation was carried out for 2 hours at 37 °C, and the absorbance at 450 nm was measured using a microplate reader. HSF were kindly provided by the Stem Cell Bank, Chinese Academy of Sciences, and they were derived from the skin fibroblasts of a foreign newborn boy. When frozen, they showed P18 generation. All experiments were performed in accordance with the guidelines of the Nanchang University ethics committee, and experiments were approved by the ethics committee at Nanchang University. Informed consent was obtained from the human participants of this study.
In vivo surgical suture studies
All animal procedures were performed in accordance with the Guidelines for Care and Use of Laboratory Animals of Nanchang University and approved by the Animal Ethics Committee of Jiangxi. SD mice were obtained from Hunan Slac Jingda Laboratory Animal Co., Ltd. Nine adult male SD mice were used for surgical suture experiments. They were divided into three groups: a PZ-loaded silk thread group, a yellow light + PZ-loaded silk thread group and a normal silk thread group. Mice were anesthetized by an intraperitoneal injection of 4% chloral hydrate (0.01 mL g−1), and then the back area of each rat was sterilized and rejected and a 2–3 cm midline incision was made. The two groups were sutured with PZ-loaded silk thread, and one of them was irradiated every day. One group was sutured with normal silk thread. An infection was then induced by adding a certain amount of a S. aureus suspension near the wound, and the incision recovery state was observed in the following week. The mice were euthanized one week later. Skin and muscle tissues near the wound of the rat were taken out and placed in an appropriate amount of PBS and a tissue fixative to facilitate plate count and paraffin embedding. The PBS containing skin and muscle tissues was applied to the medium, and then placed in a constant temperature incubator (37 °C) for 24 hours. The number of bacterial colonies in the medium was calculated. The skin and muscle tissues placed in the tissue fixative were embedded in paraffin and stained with the conventional HE stain.
Statistical analysis
All experimental data were obtained at least in triplicate. The results were expressed as mean values ± standard deviation and analyzed using the Student–Newman–Keuls (SNK) test to evaluate the significance of differences between different groups. A probability value (p-value) of <0.05 was considered statistically significant (*p < 0.05, ** p < 0.01 and ***p < 0.001).
Results and discussion
Synthesis and characterization of porous ZnO
PZ was synthesized by a microwave hydrothermal method (Fig. 2A). The porous structure could be observed using TEM and SEM (Fig. 2B–C and Fig. S2†). The composite porous structure greatly enhanced the specific surface area of the ZnO material. Elemental analysis of the energy spectrum also revealed that it was rich in C, O, and Zn elements (Fig. 2D–G). Due to appropriate doping of carbon materials, the band gap of ZnO was also lowered (Fig. S8†). Instead of conventional UV irradiation, a safer light source with longer wavelength (such as yellow-green light) can stimulate this porous material, which will fundamentally improve the safety of its photocatalytic antibacterial applications. PZ can be effectively loaded on the silk surface by a simple charge action.20,21 It can be seen from scanning electron microscopy that ZnO was uniformly decorated on the surface of the silk (Fig. 2H–I), and XRD also proved this modification (Fig. 2J).
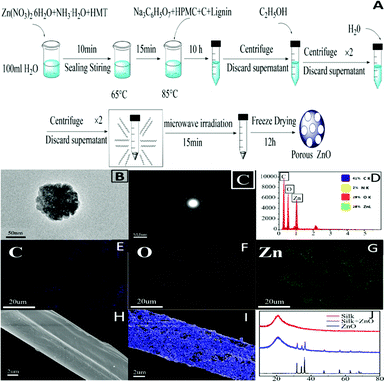 |
| Fig. 2 (A) The synthesis steps of porous ZnO. (B) SEM image of porous ZnO. (C) Lattice diffraction pattern of porous ZnO. (D–G) The EDS elemental mapping results of porous ZnO. (H–I) SEM image of original silk and ZnO@silk. (J) XRD spectra of silk (red), silk (blue) and porous ZnO (black). | |
Assessment of the properties of porous ZnO in vitro
From the pore size distribution curve (Fig. 3A), we further knew that there were different sizes of pores on PZ. The adsorption of this porous ZnO was demonstrated using Rhodamine B (Fig. 3B and Fig. S5†). This feature may also enable adsorption of harmful components in the exudate during wound care, thus accelerating wound healing. Then we evaluated the durability of the PZ on the silk surface after loading the silk. We found that in the simulated body fluid (SBF) environment, the ZnO on the surface of the silk retained a loading rate of about 50% even after one week (Fig. 3C and Fig. S4†). In the short treatment, this durability is sufficient for wound sutures. Afterward, the in vitro heating performance of PZ was also evaluated, and we found that it can rapidly heat up in a short time under excitation with NIR light (Fig. 3D–E). In theory, this obvious warming effect can effectively increase the degradation rate of the wire (Fig. 3F). Therefore, we designed an in vitro degradation experiment to observe the degradation of the wire in different environments. The results showed that with the help of NIR light, the degradation ability of the silk could be accelerated considerably.
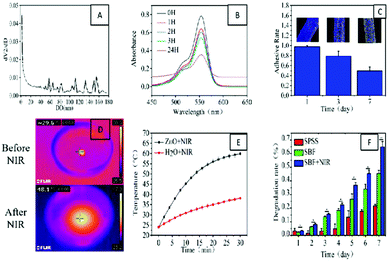 |
| Fig. 3 (A) The pore volume–pore size distribution curve of porous ZnO (differential). (B) The change of absorbance of Rhodamine B solution over time. (C) The durability of ZnO on the surface of the silk. (D) Infrared photograph of silk warming in vitro. (E) In vitro heating curve of porous ZnO. (F) Degradation of the silk over time. | |
Antibacterial property assay in vitro
The antibacterial property of this silk thread was analyzed by using Gram-negative Escherichia coli (E. coli) and Gram-positive Staphylococcus aureus (S. aureus). We quantitatively studied the antibacterial activity of the silk. A silk thread of non-PZ was used as the control group. The plate count results showed that the silk thread loaded with PZ had a killing rate of about 80% to S. aureus and E. coli (Fig. 4A–B and Fig. S6†). This demonstrated the enormous potential of the silk thread in its resistance to infection. It has been reported that Zn2+ has an excellent antibacterial effect and that Zn2+ can enhance oxidative stress and combine with bacteria to change the fluidity of the bacterial membrane.22,23
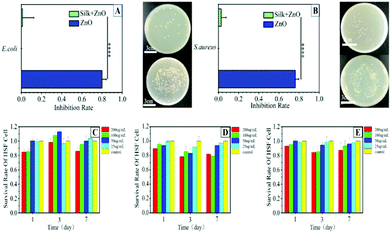 |
| Fig. 4 (A–B) The inhibition rate of silk and ZnO@silk and an image of agar plate colony number. (C–E) The cytotoxicity assay histogram for HSF cells after immersion of ZnO@silk for 1, 3, and 7 days, respectively. | |
Cytotoxicity assessment in vitro
Favorable cytocompatibility is a vital criterion for clinical applications of the silk. Therefore, we selected human fibroblasts (HSF) to assess the cytotoxicity. We immersed the silk for 1, 3, and 7 days, and treated the cells with 25, 50, 100, and 200 μg mL−1 immersion solutions, respectively (Fig. 4C–E). Even the highest concentration group had no more than an 80% killing rate for HSF, and the immersion liquid after 7 days did not have much negative impact on the cells. This suggested that the silk possessed desirable cytocompatibility and could be further utilized in vivo.
Effect assessment in vivo
We simulated the common surgical incision in the dorsal tissue of the mice and sutured with silk thread to observe the healing of the incision. It can be found that the healing condition is better than that of the control group after loading PZ, and the effect is further increased in the presence of yellow light (Fig. 5A–C). It can be seen from the incision skin and nearby muscle tissues that the bacterial infection of the wound is suppressed (Fig. 5D and Fig. S7†), and the healing range of the incision can also reveal that the yellow light can accelerate healing (Fig. 5E). HE staining also yielded corresponding results (Fig. 5F–K). The whole system had greatly promoted the early repair and post-treatment of wounds. The results of animal experiments showed the potential of clinical application of this PZ modified silk thread.
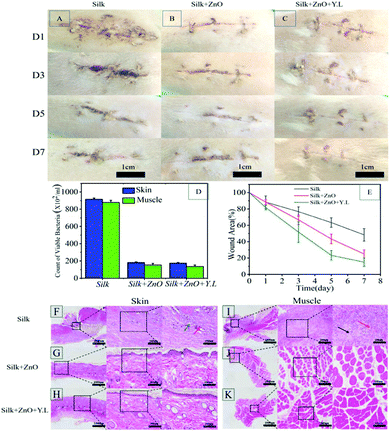 |
| Fig. 5 (A–C) Optical photographs of wound healing at 1, 3, 5 and 7 days: the silk group, silk + ZnO group and silk + ZnO + Y.L. (from left to right). (D) Count of viable bacteria on the skin (blue) and muscle (green) around the wound. (E) The wound area curves of three groups. (F–K) HE staining results of skin and muscle around the wound. | |
Conclusions
In summary, sutures have a positive impact on wound healing, infection prevention, scar formation, etc.24,25A desirable surgical suture material should have excellent antibacterial effect and accelerated tissue healing ability. After the wound is healed, rapid self-degradation is also preferred to be triggered according to the actual situation of different people. In this study, we have prepared a PZ modified silk thread with antibacterial activity and favorable cytocompatibility. The results showed that the as-prepared silk thread can not only prevent wound infection and accelerate wound healing, but also exhibit unique light defined degradation. Although the degradation rate needs to be further improved, we have created a new research direction for the development of suture degradation. On the other hand, the biocompatibility of the nano-ZnO synthesized by us needs to be further improved, and the detailed mechanism of yellow-light's benefits in wound recovery is still unclear. The ongoing research will focus on these problems.
Conflicts of interest
There are no conflicts to declare.
Acknowledgements
This work was supported by the National Natural Science Foundation of China (No. 31860263 to Xiaolei Wang; No. 21461015 to Xiaolei Wang) and the Science Foundation of Jiangxi Provincial Department of Education (KJLD14010, 20153BCB23035, 20161ACB21002, and 20165BCB19002 to Xiaolei Wang).
References
- L.-D. Koh, Y. Cheng, C.-P. Teng, Y.-W. Khin, X.-J. Loh, S.-Y. Tee, M. Low, E. Ye, H.-D. Yu, Y.-W. Zhang and M.-Y. Han, Prog. Polym. Sci., 2015, 46, 86–110 CrossRef CAS.
- R. D. Abbott, E. P. Kimmerling, D. M. Cairns and D. L. Kaplan, ACS Appl. Mater. Interfaces, 2016, 8, 21861–21868 CrossRef CAS.
- G. Lu, Z. Ding, Y. Wei, X. Lu, Q. Lu and D. L. Kaplan, ACS Appl. Mater. Interfaces, 2018, 10, 44314–44323 CrossRef CAS.
- S. Selvaraj and N. N. Fathima, ACS Appl. Mater. Interfaces, 2017, 9, 5916–5926 CrossRef CAS.
- L. Shi, F. Wang, W. Zhu, Z. Xu, S. Fuchs, J. Hilborn, L. Zhu, Q. Ma, Y. Wang, X. Weng and D. A. Ossipov, Adv. Funct. Mater., 2017, 27 Search PubMed.
- F. Han, S. Liu, X. Liu, Y. Pei, S. Bai, H. Zhao, Q. Lu, F. Ma, D. L. Kaplan and H. Zhu, Acta Biomater., 2014, 10, 921–930 CrossRef CAS PubMed.
- C. Holland, K. Numata, J. Rnjak-Kovacina and F. P. Seib, Adv. Healthcare Mater., 2019, 8, 1800465 CrossRef.
- X. Chen, D. Hou, X. Tang and L. Wang, J. Mech. Behav. Biomed. Mater., 2015, 50, 160–170 CrossRef CAS.
- X. Chen, D. Hou, L. Wang, Q. Zhang, J. Zou and G. Sun, ACS Appl. Mater. Interfaces, 2015, 7, 22394–22403 CrossRef CAS.
- D.-Q. Wu, H.-C. Cui, J. Zhu, X.-H. Qin and T. Xie, J. Mater. Chem. B, 2016, 4, 2606–2613 RSC.
- A. J. Choudhury, D. Gogoi, J. Chutia, R. Kandimalla, S. Kalita, J. Kotoky, Y. B. Chaudhari, M. R. Khan and K. Kalita, Surgery, 2016, 159, 539–547 CrossRef PubMed.
- Y. J. Yang, Y. Kwon, B. H. Choi, D. Jung, J. H. Seo, K. H. Lee and H. J. Cha, Biomacromolecules, 2014, 15, 1390–1398 CrossRef CAS.
- N. Vigneshwaran, S. Kumar, A. A. Kathe, P. V. Varadarajan and V. Prasad, Nanotechnology, 2006, 17, 5087–5095 CrossRef CAS.
- F. Yu, X. Fang, H. Jia, M. Liu, X. Shi, C. Xue, T. Chen, Z. Wei, F. Fang, H. Zhu, H. Xin, J. Feng and X. Wang, Chemistry, 2016, 22, 8053–8058 CrossRef CAS.
- J. Li, L. Tan, X. Liu, Z. Cui, X. Yang, K. W. K. Yeung, P. K. Chu and S. Wu, ACS Nano, 2017, 11, 11250–11263 CrossRef CAS.
- M. Li, X. Liu, L. Tan, Z. Cui, X. Yang, Z. Li, Y. Zheng, K. W. K. Yeung, P. K. Chu and S. Wu, Biomater. Sci., 2018, 6, 2110–2121 RSC.
- Y. Zhang, X. Liu, Z. Li, S. Zhu, X. Yuan, Z. Cui, X. Yang, P. K. Chu and S. Wu, ACS Appl. Mater. Interfaces, 2018, 10, 1266–1277 CrossRef CAS.
- J. Lin, X. Ding, C. Hong, Y. Pang, L. Chen, Q. Liu, X. Zhang, H. Xin and X. Wang, Sci. Rep., 2019, 9, 7560 CrossRef.
- H. Liao, X. Miao, J. Ye, T. Wu, Z. Deng, C. Li, J. Jia, X. Cheng and X. Wang, ACS Appl. Mater. Interfaces, 2017, 9, 13009–13015 CrossRef CAS.
- Y. Gong, T. Andelman, G. F. Neumark, S. O'Brien and I. L. Kuskovsky, Nanoscale Res. Lett., 2007, 2, 297–302 CrossRef CAS.
- A. S. Lammel, X. Hu, S. H. Park, D. L. Kaplan and T. R. Scheibel, Biomaterials, 2010, 31, 4583–4591 CrossRef CAS.
- Y. Xiang, X. Liu, C. Mao, X. Liu, Z. Cui, X. Yang, K. W. K. Yeung, Y. Zheng and S. Wu, Mater. Sci. Eng., C, 2018, 85, 214–224 CrossRef CAS.
- Y. Xiang, C. Mao, X. Liu, Z. Cui, D. Jing, X. Yang, Y. Liang, Z. Li, S. Zhu, Y. Zheng, K. W. K. Yeung, D. Zheng, X. Wang and S. Wu, Small, 2019, 15, e1900322 CrossRef.
- J. Boateng and O. Catanzano, J. Pharm. Sci., 2015, 104, 3653–3680 CrossRef CAS.
- C. Ghobril and M. W. Grinstaff, Chem. Soc. Rev., 2015, 44, 1820–1835 RSC.
Footnote |
† Electronic supplementary information (ESI) available. See DOI: 10.1039/c9bm01422e |
|
This journal is © The Royal Society of Chemistry 2020 |
Click here to see how this site uses Cookies. View our privacy policy here.