DOI:
10.1039/C9FO00811J
(Paper)
Food Funct., 2020,
11, 211-220
Evolution of important glucosinolates in three common Brassica vegetables during their processing into vegetable powder and in vitro gastric digestion
Received
19th April 2019
, Accepted 5th December 2019
First published on 6th December 2019
Abstract
Evolution of important glucosinolates (GLSs), namely, sinigrin, glucoraphanin, glucoerucin and glucobrassicin, in three commonly consumed Brassica vegetables viz. white cabbage, Chinese cabbage and bok choy during their processing into vegetable powder was investigated. Drying was noted to be a major processing step causing significant losses of GLSs. Interestingly, different GLSs and even the same GLSs in different vegetables showed different thermal stabilities during drying. The stability of GLSs in vegetable powder during in vitro gastric digestion was also studied. Glucoraphanin exhibited the highest stability while glucobrassicin was the most vulnerable GLS under in vitro gastric conditions. White cabbage is found to be a promising material for the production of vegetable powder as it contains high contents of GLSs, especially glucoraphanin and glucoerucin, which are important precursors of anticarcinogenic compounds, namely sulforaphane and erucin. These two GLSs were also noted to be stable during in vitro gastric digestion.
1. Introduction
Dietary fiber (DF) is an important group of diets, which, when consumed in sufficient amount, is capable of conferring several health benefits, including improved digestion and gut microbiota modulation, among others. Besides the ingestion of DF that is naturally available in fruits and vegetables, DF can be prepared and consumed in a dry form. Such a form in many cases is preferred as it can be consumed more conveniently; DF in the dry form also has high stability and has an extended shelf-life. Brassica vegetables are among the many promising candidates for the production of DF in a dried powdery form. This is because numerous epidemiological studies have revealed that adequate consumption of Brassica vegetables can play an important role in the prevention of many chronic diseases, including cardiovascular disease, type II diabetes, obesity and some cancers.1 Such beneficial functions are attributed to a certain group of phytochemicals, namely, glucosinolates (GLSs), which are abundantly found in these vegetables.2
GLSs are naturally stored in plant S-cell vacuoles. When plant tissues are damaged, either during food preparation or chewing, myrosinase (thioglucoside glucohydrolase, EC 3.2.3.1), which is originally present in plant myrosin cells, would be released and come into contact with GLSs, resulting in the hydrolysis of these compounds into various derivatives. Among the hydrolysis products, isothiocyanates and indole-3-carbinol are biologically active compounds exhibiting anticarcinogenic activities.3,4 For those GLSs that have passed through the GI tract and remained in the digesta, hydrolysis may also occur in the large intestine under the action of colonic microflora exhibiting myrosinase-like activity.5
Understanding the evolution of GLSs, both during powder preparation and digestion of the resulting powder, is crucial to the maintenance of the nutritional quality of DF powder. Key processing steps, including blanching and drying, are known to affect the contents and activities of bioactive compounds and endogenous enzymes in plants. In the case of Brassica vegetables, since the desirable anticancer compounds are mostly heat-sensitive and degrade significantly upon gastric digestion, myrosinase, which catalyzes the hydrolysis reaction of GLSs, should first be inactivated. Phytochemicals obtained during the consumption of Brassica vegetable powders would mostly be GLSs and not their sensitive derivatives.6–8 The unconverted GLSs could be released during digestion and utilized by the microflora in the colon to generate the desirable compounds.
Besides the change in GLSs as influenced by the powder production process, the stability of the phytochemicals during digestion is also of great concern. Throughout the human digestive system, GLSs are subject to rather extreme conditions with abundant and intensive enzymatic activities at both acidic and alkaline pH. Vallejo et al.9 found a 69% decrease in the total GLS content in broccoli during in vitro gastric digestion and a further decrease (12% loss) after intestinal digestion. These studies focused nevertheless only on the profiles of glucoraphanin in fresh and steamed vegetables. However, important GLSs present in Brassica vegetables also include sinigrin, glucoerucin, and glucobrassicin; all these phytochemicals could also generate hydrolysis products with anticancer properties. The fate of these individual GLSs during the production and digestion of Brassica vegetable powder remains unclear. This is particularly applicable to the processes of drying and grinding, which are indispensable for the production of dried vegetable powder. A comparison study between the results obtained for different Brassica vegetables undergoing the same controlled processes has also never been conducted, so conclusion on the effects of the processing steps as well as digestion on the different GLSs in different vegetables can never be drawn.
This work aimed at studying the effects of necessary processing steps (steam blanching, drying and grinding) on the retention of important GLSs, i.e., sinigrin, glucoraphanin, glucoerucin and glucobrassicin, during the production of vegetable powder from three commonly consumed Brassica vegetables (i.e., white cabbage, Chinese cabbage and bok choy). Changes in the GLS contents during in vitro gastric digestion of the resulting vegetable powder were also investigated.
2. Materials and methods
2.1 Preparation of vegetable powder
White cabbage (Brassica oleracea L. var. capitata), Chinese cabbage (Brassica rapa L. ssp. pekinensis) and bok choy (Brassica rapa L. ssp. chinensis) were obtained from a specific seller at a local market in Suzhou, Jiangsu, China. The vegetables were kept at 4 °C until the time of experiment, which was no longer than 3 days.
Prior to each experiment, the outermost leaves of the vegetables were removed. The leaves in the 2nd to 4th layers of a vegetable head, which are usually discarded during vegetable preparation in the consumer market, were used to prepare vegetable powder. Such a practice can eventually lead to a process that can be used to add value to the otherwise waste products in the future. The leaves were washed under running tap water and drained on a screen to get rid of excess water. Dry mass of the fresh sample was determined using a gravimetric method at 105 °C (AOAC method 984.25).10
Steam blanching was carried out using an electric steam oven (Media, TPN26MSS-SSL, Dongguan, China); the whole leaves were placed as a single layer on a perforated tray suspended over boiling water. The suitable steam blanching time for each vegetable was predetermined based on peroxidase and myrosinase inactivation. After blanching the leaves were immediately cooled in cold water (4 °C). The blanched leaves were sliced and then chopped using an electric chopper (Braun, K600 Type 3205, Kronberg, Germany) at 11
000 rpm for 1 min to obtain the sample size of approximately 1–3 mm.
Drying was performed by spreading 120 g of each prepared sample as a single layer over a cooking sheet placed on a meshed wire tray. The sample was dried at 80 °C using a laboratory-scale hot air oven (Hengzi, GZF-GF101-4-BS-II, Shanghai, China). This drying temperature was suggested by Tanongkankit et al.11 as the temperature that provided dried cabbage powder with the highest retention of important phytochemicals. Drying was continued until the mass of the sample reached an equilibrium value. The drying curve was then obtained and used to determine the time needed to dry the sample to the desired final moisture content of around 10% dry basis.12 Drying was conducted at a constant air velocity of 0.5 m s−1 and relative humidity in the range of 65–90%. After drying, the dried sample was ground into fine powder using a ball mill (MITR, YXQM-0.4L, Shanghai, China) for 10 min to obtain the particle size of less than 300 μm.12
2.2 Determination of myrosinase activity
2.2.1 Myrosinase extraction.
Myrosinase extraction was performed following the methods described by Charron et al.13 with some modification. Fresh or steamed vegetables were sliced into small pieces using a stainless-steel knife and then chopped using an electric chopper for 1 min. Twenty-five g of the sample was homogenized with 50 mL of chilled 30 mM citrate/phosphate buffer (pH 7) using an ultraturrax (IKA, T18, Staufen, Germany) at 10
000 rpm for 1 min. The mixture was filtered through a 425 μm stainless steel screen; the filtrate was placed into a 50 mL tube and centrifuged using a refrigerated centrifuge (Heal Force, Neofuge 18R, Shanghai, China) at 17
500g at 4 °C for 15 min. The supernatant was again filtered through Whatman No. 1 filter paper; the filtrate was kept at 4 °C until further analysis.
2.2.2 Quantification of myrosinase activity.
A reaction mixture containing 50 μL of the above-mentioned crude enzyme extract, 1.35 mL of 32.22 mM citrate/phosphate buffer (pH 6.5) with 1.07 mM EDTA and 100 μL of 37.50 mM sinigrin was prepared in a 5 mL plastic tube at 25 ± 1 °C. One hundred μL of Milli-Q water was used as a reference. The contents of each tube were mixed using a vortex mixer (TZL-5009, Suzhou Percival Laboratory Equipment Co. Ltd, Suzhou, China) for 30 s. The absorbance (at 227 nm) of sinigrin was measured using a UV-vis spectrophotometer (Molecular Devices, Spectramax M5, Sunnyvale, CA) at every 5 s for a period of 5 min. Myrosinase activity was determined from the linear slope of a plot between the absorbance of sinigrin and the reaction time, which represents the rate of disappearance of sinigrin from the reaction mixture. A unit (U) of activity is defined as a decrease in the absorbance resulting from the hydrolysis of 1 μmol of sinigrin per min.
2.3 Determination of GLS contents
2.3.1 Sample extraction.
The extraction of GLSs was performed following the methods described by Lee et al.14 with some modification. Fresh or steamed vegetables were sliced into small pieces using a stainless-steel knife and chopped using an electric chopper for 1 min. Based on the same dry-mass ratio of the sample and methanol, approximately 25 g of fresh and steamed samples or approximately 0.5 g of dried and powdery samples was shaken with 50 mL of methanol in a water-bath shaker (ZSIMC, ZWY-110X30, Hangzhou, China) at 100 rpm at 70 °C for 15 min. The mixture was cooled to room temperature (25 °C) and filtered through Whatman No. 1 filter paper. The retentate was re-extracted with 50 mL of methanol in the water-bath shaker under the same conditions for 3 min. The methanol fraction was dehydrated using a rotary evaporator (IKA, RV 10, Staufen, Germany) at 50 °C at 100 rpm. The residue was dissolved in 5 mL of methanol and the solution was filled into a microcentrifuge tube and kept at −78 °C until further analysis.
2.3.2 Quantification of GLSs.
Sep-pak® Florisil 6 cc cartridge (Waters, Milford, MA) was used to eliminate non-GLS interferences from the crude GLS extract. The activated cartridge was pre-cleaned with 5 mL of 30% (v/v) dichloromethane in hexane. Then, 1 mL of the crude GLS extract was injected into the cartridge. The interferences were washed from the cartridge using 5 mL of 30% (v/v) dichloromethane in hexane. GLSs were eluted from the cartridge three times using 5 mL of 30% (v/v) ethyl acetate in methanol. The fraction was evaporated to dryness using a rotary evaporator at 50 °C at 100 rpm for 10 min; the content was reconstituted with 500 μL of HPLC water. The solution was then filtered through a 0.2 μm nylon filter and was collected for HPLC analysis.
Ten μL of the filtrate was injected into an Xselect CSH C18 HPLC column (4.6 × 250 mm) (Waters, Milford, MA) using a gradient elution composed of 30 mM ammonium acetate buffer at pH 5 (denoted as component A) and methanol (denoted as component B) as the mobile phase. The gradient elution conditions were: 100% A–0% B for 5 min, then gradually increased to 70% A–30% B from 5 to 25 min, maintained at 70% A–30% B for 5 min, adjusted to 100% A–0% B in 1 min, and maintained at this composition for another 5 min. The flow rate of the mobile phase was set at 1 mL min−1. A UV detector at a wavelength of 233 nm was used for detecting individual GLSs. The individual GLS content was calculated from the standard curves of sinigrin (Sigma-Aldrich, St Louis, MO), glucoraphanin potassium salt (Chromadex, Irvine, CA), glucoerucin (Chromadex, Irvine, CA) and glucobrassicin (AppliChem, St Louis, MO). Each standard curve was prepared at the concentrations of 0–100 μg g−1.
2.4
In vitro digestion
Artificial saliva was prepared following the methods described by Wu et al.15 Briefly, α-amylase (from Bacillus subtilis, 10
070, ∼59.9 U mg−1) was dissolved in 1 mL of simulated saliva fluid (SSF) at a concentration of around 7982 U mL−1; SSF in turn contained KCl (15.1 mmol L−1), KH2PO4 (3.7 mmol L−1), NaHCO3 (13.6 mmol L−1), MgCl2(H2O)6 (0.15 mmol L−1) and (NH4)2CO3 (0.06 mmol L−1).16 The pH of the artificial saliva was adjusted to 7.80 ± 0.44 using 1 M NaOH. The resulting saliva was maintained at 37 °C.
Artificial gastric juice was prepared following the methods of Wu et al.15 Pepsin (from porcine gastric mucosa, P7125, ≥400 U mg−1 solids) and mucin were dissolved in 50 mL of simulated gastric fluid (SGF) to achieve the concentrations of 250 U mL−1 and 1.5 g L−1. Note that SGF contained KCl (6.9 mmol L−1), KH2PO4 (0.9 mmol L−1), NaHCO3 (25 mmol L−1), NaCl (47.2 mmol L−1), MgCl2(H2O)6 (0.1 mmol L−1) and (NH4)2CO3 (0.5 mmol L−1).16 The pH of the artificial gastric juice was adjusted to 1.63 ± 0.01 using 1 M HCl. The resulting juice was also maintained at 37 °C.
In vitro gastric digestion was performed using the methods described by Wu et al.15 with some modification. One g of vegetable powder was combined with 1 mL of artificial saliva and 5.03 mL of artificial gastric juice to simulate the digestion in an in vitro rat stomach model.17 The content was mixed using a vortex mixer for 1 min and then placed in a shaking incubator (LiTekvo, THZ-98AB, Shanghai, China) at 100 rpm at 37 °C for 3 h. The sample was collected at time intervals and then centrifuged at 17
500g for 20 min at 25 °C to separate the digestive fluid from the digested sample. One mL of the digestive fluid was filtered through a 0.2 μm nylon filter prior to HPLC analysis of GLSs following the procedures described in section 2.3.2.
2.5 Microstructure evaluation
The microstructure of the prepared vegetable powder as well as that of the digested sample were examined using a scanning electron microscope (Hitachi, SU1510, Tokyo, Japan). In the case of the digested sample, a specimen was placed in a freezer at −78 °C for 4 h, after which it was dehydrated in a freeze dryer (Xiangyi, LGJ-10D, Hunan, China) for 48 h. All samples were coated with platinum in an ion sputter (Hitachi, MC1000, Tokyo, Japan) before being observed and photographed at 200× and 1000× magnifications.
2.6 Statistical analysis
The experimental data were subject to analysis of variance (ANOVA) and are presented as mean values with standard deviations. Differences between the mean values were established using Duncan's new multiple range tests at a confidence level of 95%. All statistical analyses were performed using SPSS® software (version 17, SPSS Inc., Chicago, IL). All experiments were independently performed at least in triplicate.
3. Results and discussion
3.1 GLS profiles of fresh Brassica vegetables
GLS profiles of the three Brassica vegetables are given in Table 1. The total GLS content reported in this study was calculated as the sum of the four GLSs. White cabbage possessed the highest total GLS content, followed by Chinese cabbage and bok choy; bok choy indeed possessed the lowest contents of all four tested GLSs. Similar results were reported by He et al.18 who reported that the total GLS content of bok choy was much lower than those of white cabbage and Chinese cabbage.
Table 1 Contents of GLSs (μmol per 100 g dry mass) in fresh Brassica vegetables
Trivial name |
Compound group |
Chemical name of side chain |
Structure of side-chain (R=) |
White cabbage |
Chinese cabbage |
Bok choy |
Each value represents mean ± SD of three replicates. ND = not detected. Different lowercase letters in the same column indicate that the values are significantly different (p ≤ 0.05). Different uppercase letters in the same row indicate that the values are significantly different (p ≤ 0.05). |
Sinigrin |
Aliphatic |
2-Propenyl |
|
463.3 ± 112.5b,B |
30.4 ± 2.9a,A |
ND |
Glucoraphanin |
Aliphatic |
4-(Methylsulfinyl)butyl |
|
173.1 ± 13.5a,B |
120.1 ± 38.2a,A |
71.5 ± 20.5a,A |
Glucoerucin |
Aliphatic |
4-(Methylsulfanyl)butyl |
|
70.3 ± 9.9a,B |
39.4 ± 7.7a,A |
31.5 ± 6.3a,A |
Glucobrassicin |
Indole |
3-Indolylmethyl |
|
322.1 ± 40.5b,B |
743.2 ± 115.2b,C |
143.6 ± 40.2b,A |
Total 4 GLSs |
|
1028.7 ± 129.7c,B |
933.1 ± 84.1c,B |
246.6 ± 36.1c,A |
Amongst the four kinds of GLSs, sinigrin and glucobrassicin (463.3 and 322.1 μmol per 100 g dry mass, respectively) were the abundant ones in white cabbage. These results are consistent with those reported by other researchers.19,20 In particular, the amount of sinigrin in white cabbage was significantly higher than those in the other two vegetables. The sinigrin content in Chinese cabbage (21.0 μmol per 100 g dry mass) was less than 1/10 compared to that in white cabbage, while the sinigrin content in bok choy was too low to be detected. The absence of sinigrin in bok choy is in agreement with that mentioned in the report of Wiesner et al.21 The glucoraphanin content of white cabbage (173.1 μmol per 100 g dry mass) was within the previously reported range, viz. 167–1070 μmol per 100 g dry mass.22 Glucoerucin was detected only in a small amount; similar findings were reported by other investigators. Kushad et al.22 and Park et al.23 reported that the glucoerucin contents in white cabbage were 49 and 69 μmol per 100 g dry mass, respectively.
Glucobrassicin was found to be the dominant GLS in Chinese cabbage, with a much larger amount (743.2 μmol per 100 g dry mass) compared with those of the other three GLSs (aliphatic GLSs). The glucobrassicin content in Chinese cabbage was the highest compared to those in the three tested vegetables (322.1 and 143.6 μmol per 100 g dry mass for white cabbage and bok choy, respectively). Yang and Quiros24 reported that the contents of indole GLSs in Chinese cabbage were significantly higher than those of aliphatic GLSs. The highest glucobrassicin content reported in the present study was higher than that reported by Kim et al.,25 where the glucobrassicin contents in 24 cultivars of Chinese cabbages were in the range of 13 to 631 μmol per 100 g dry mass.
Glucobrassicin was also found to be the major GLS in bok choy although the content was much lower than those found in white cabbage and Chinese cabbage. Glucobrassicin contents reported in this study were in the range of those found in 13 cultivars of bok choy (68–284 μmol per 100 g dry mass) reported by Wiesner et al.21 but higher than those reported by Zhu et al.26 in 7 different cultivars of bok choy (47.6–103.9 μmol per 100 g dry mass).
By comparing the results obtained here, white cabbage was found to be the most interesting source of GLSs, especially glucoraphanin and glucoerucin, which are the precursors of sulforaphane and erucin, the potent anticancer compounds.
3.2 Evolution of GLSs during the preparation of vegetable powder
Steam blanching was selected as an appropriate treatment prior to drying as it has proved to exhibit minute effect on GLSs.11 The results indicated, as expected, that the activities of peroxidase and myrosinase decreased with increased blanching time (Table 2). Thermal stabilities of peroxidase and myrosinase from different vegetables were different. Peroxidase and myrosinase in Chinese cabbage were more stable than those in white cabbage and bok choy, showing remaining activities of 2.76% and 8.42%, respectively, after 2 min of steam blanching. Myrosinase was noted to be slightly more thermostable than peroxidase in all three vegetables. Based on these data, the suitable time for steam blanching white cabbage and bok choy was 2 min, whereas the suitable blanching time for Chinese cabbage was 3 min.
Table 2 Remaining activities of peroxidase and myrosinase in Brassica vegetables after steam blanching
Vegetable |
Steam blanching time (min) |
% Remaining activity |
Peroxidase |
Myrosinase |
Each value represents mean ± SD of three replicates. ND = not detected. |
White cabbage |
Fresh sample |
100.00 ± 0.00 |
100.00 ± 0.00 |
1 |
6.56 ± 3.54 |
12.84 ± 1.35 |
2 |
ND |
ND |
3 |
ND |
ND |
|
Chinese cabbage |
Fresh sample |
100.00 ± 0.00 |
100.00 ± 0.00 |
1 |
10.12 ± 2.06 |
32.84 ± 11.45 |
2 |
2.76 ± 1.51 |
8.42 ± 2.49 |
3 |
ND |
ND |
|
Bok choy |
Fresh sample |
100.00 ± 0.00 |
100.00 ± 0.00 |
1 |
3.28 ± 1.73 |
10.07 ± 5.82 |
2 |
ND |
ND |
3 |
ND |
ND |
Changes in individual GLS contents in Brassica vegetables during different processing steps, i.e., blanching, drying and grinding, are shown in Fig. 1. The results showed that blanching could help preserve GLSs due to the rapid inactivation of myrosinase. Steam blanching did not have a significant effect on the contents of sinigrin, glucoraphanin and glucoerucin. In contrast, glucobrassicin was slightly more sensitive to heat during steaming. It has indeed been reported that indole GLSs (e.g., glucobrassicin) are more thermolabile than the aliphatic GLSs (e.g., glucoiberin, sinigrin and glucoraphanin).27,28
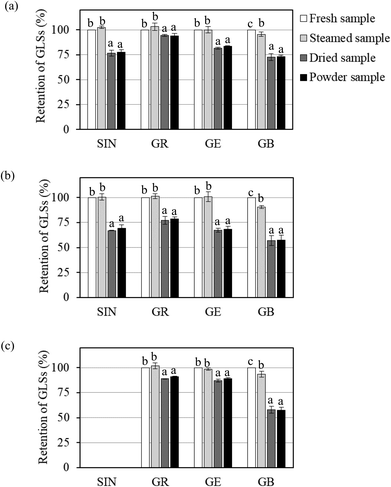 |
| Fig. 1 Retention of GLSs in three common Brassica vegetables during their preparation into vegetable powder: (a) white cabbage; (b) Chinese cabbage and (c) bok choy. The measured GLSs are sinigrin (SIN), glucoraphanin (GR), glucoerucin (GE) and glucobrassicin (GB). Different letters over the bars belonging to the same GLSs indicate that the values are significantly different (p ≤ 0.05). | |
The required drying time for white cabbage, Chinese cabbage and bok choy to reach the desired final moisture content of less than 0.1 g per g dry basis was 210, 240 and 180 min, respectively. A significant reduction in the GLS contents was observed during drying, while no further loss during grinding was observed, as shown in Fig. 1. Previous studies have indicated that drying caused a significant reduction in GLSs. Chaisamlitpol et al.,29 for example, reported that GLSs in white cabbage undergoing drying started to degrade at around 70 °C. Approximately 19–33% of GLSs in Thai Brassica vegetables (Chinese cabbage, Chinese kale and Chinese mustard) degraded during hot air drying at 60–80 °C.7
The overall retention of GLSs in vegetable powders as compared with the contents in the fresh leaves is given in Table 3. The results showed that sinigrin (retention of 69.3%–77.5%), glucoraphanin (retention of 78.5%–94.0%) and glucoerucin (retention of 68.1%–88.8%) showed higher stabilities than glucobrassicin (retention of 57.4%–73.3%). Among the three aliphatic GLSs, glucoraphanin exhibited the highest thermal stability. This is due to the chemical structure of glucoraphanin. Methylsulfinyl GLSs (e.g., glucoraphanin) are generally more stable than methylsulfanyl GLSs (e.g., glucoerucin).30 The results further illustrated that even the same GLSs in different vegetables showed different thermal stabilities. For example, glucoraphanin in white cabbage (retention of 94.0%) exhibited higher thermal stability than that in Chinese cabbage and bok choy (retention of 78.5% and 90.8%, respectively), while glucoerucin in bok choy (retention of 88.8%) was thermally more stable than that in white cabbage and Chinese cabbage (retention of 83.4% and 68.1%, respectively). Generally speaking, GLSs in Chinese cabbage showed the lowest thermal stability. Dekker et al.31 also found large differences in the thermal stabilities of the same GLSs in different Brassica vegetables. Sarvan et al.32 reported that glucoraphanin in white cabbage showed higher thermal stability than that in red cabbage and Brussels sprouts. Such differences might be from the natural environment in the plant tissues where GLSs are present; cellular compositions and/or chemical changes as well as water content in the cell structure may also lead to such differences.33
Table 3 Overall contents and retention of GLSs in vegetable powder prepared from Brassica vegetables
Type of GLS |
Individual GLS |
GLS content (μmol per 100 g dry mass) |
White cabbage |
Chinese cabbage |
Bok choy |
Each value represents mean ± SD of three replicates. ND = not detected. Data in parentheses are percentage of GLS retention in vegetable powder in terms of (C/C0) × 100, where C is the GLS content in DF powder and C0 is the GLS content in a fresh sample. Different lowercase letters in the same column indicate that values in parentheses are significantly different (p ≤ 0.05). Different capital letters in the same row indicate that values in parentheses are significantly different (p ≤ 0.05). |
Aliphatic GLSs |
Sinigrin |
360.5 ± 97.5 (77.5 ± 2.6)b,B |
21.0 ± 1.8 (69.3 ± 3.6)bc,A |
ND |
— |
Glucoraphanin |
162.9 ± 16.4 (94.0 ± 2.3)d,C |
93.8 ± 27.0 (78.5 ± 2.2)c,A |
64.8 ± 18.5 (90.8 ± 0.5)c,B |
Glucoerucin |
58.6 ± 8.5 (83.4 ± 0.5)c,B |
27.0 ± 6.2 (68.1 ± 3.1)b,A |
28.0 ± 5.5 (88.8 ± 1.0)c,C |
Indole GLSs |
Glucobrassicin |
235.8 ± 27.0 (73.3 ± 1.1)a,B |
423.4 ± 38.4 (57.4 ± 4.7)a,A |
82.1 ± 20.2 (57.5 ± 2.6)a,A |
|
|
Total 4 GLSs |
817.8 ± 102.5 |
565.2 ± 20.9 |
174.9 ± 17.5 |
|
% Total retention |
(79.5 ± 0.3)b,C |
(60.8 ± 4.3)ab,A |
(71.3 ± 3.6)b,B |
Overall, the results showed that drying is the critical step controlling the amounts of GLSs in the vegetable powder produced from Brassica vegetables. Thermal stability of each GLS varied greatly in the same vegetable, where indole GLSs were more thermolabile than aliphatic GLSs regardless of the type of vegetable. Thermal stabilities of the same GLSs presented in different vegetables were also different, with Chinese cabbage experiencing the lowest retention of all four GLSs.
By selecting glucoraphanin as a target GLS, white cabbage is considered as the best choice of raw material to produce vegetable powder. Glucoraphanin in white cabbage exhibited the highest thermal stability compared to that in Chinese cabbage and bok choy. In addition, the abundance of sinigrin and glucobrassicin in white cabbage would also help increase the nutritional value of vegetable powder. The sum of 4 GLS contents in the white cabbage powder produced in the present study was 817.8 μmol per 100 g dry mass.
3.3 Stability of GLSs in vegetable powder during in vitro gastric digestion
Fig. 2 presents the evolution of individual GLSs during in vitro gastric digestion of the vegetable powder in terms of the normalized bioaccessible GLS content, which is defined as the ratio of instantaneous GLS content in digestive fluid to the GLS content in undigested vegetable powder. Digestion profiles of each GLS were similar in all three vegetable powder. The content of glucoraphanin in the digestive fluid gradually increased (C/C0 around 1.1) at the initial 30 min of the digestion process; the content then leveled off toward the end of the process (3 h). Glucoerucin exhibited a similar evolution pattern, except that a slight decrease toward the end of the digestion experiment was observed in the case of Chinese cabbage powder. In contrast, the contents of sinigrin and glucobrassicin in the fluids digesting white cabbage and Chinese cabbage powder showed a gradual decrease as the digestion experiments progressed (Fig. 2a and b). In the digestion process of bok choy, sinigrin was not detected in the fresh vegetable as well as in the vegetable powder (Fig. 1), while glucobrassicin could not be detected after digestion, probably because its initial amount in the powder was too low (82.1 μmol per 100 g dry mass). Similar digestion profiles observed for different types of vegetable powders indicated that changes in the amount of GLSs during gastric digestion are mainly dependent on the chemical properties of each GLSs rather than the source of vegetable used to prepare the powder.
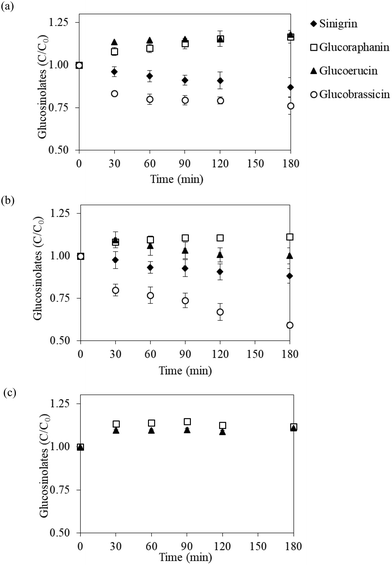 |
| Fig. 2 Evolution of GLSs (C/C0) in digestive fluid during in vitro gastric digestion of three vegetable powder in terms of normalized bioaccessible GLS contents. (a) White cabbage powder; (b) Chinese cabbage powder and (c) bok choy powder. The measured GLSs are sinigrin (◆), glucoraphanin (□), glucoerucin (▲) and glucobrassicin (○). | |
The unexpectedly higher normalized bioaccessible glucoraphanin and glucoerucin contents could be due to the contact between the vegetable powder and digestive fluid. The acidic environment and digestive enzymes in the reaction mixture might induce more severe damage to plant tissues compared to the standard extraction procedures that were used in the quantification assays. In addition, glucoraphanin appeared to be stable in the digestive fluid with a negligible decrease during the 3 h digestion. As a result, accumulation of glucoraphanin in the digestive fluid, especially in the cases of that from white cabbage and Chinese cabbage, at prolonged digestion time was observed (see Fig. 2a and b). In comparison with the increase shown by glucoraphanin and glucoerucin, sinigrin and glucobrassicin seemed to be more susceptible to the acidic environment during digestion. The degradation rates of these GLSs were most probably higher than the rates of release from the powder. It is indeed possible that the short duration that was employed to adjust the pH to gastric conditions (pH 1.63) was sufficient to hydrolyze sinigrin and glucobrassicin present in the samples.
Fig. 3 presents the microstructures of vegetable powder from white cabbage, Chinese cabbage and bok choy before (t = 0) and after in vitro gastric digestion (t = 30 and 180 min). It is clearly seen that the microstructure of the vegetable powder was significantly modified by the digestive fluid. Before digestion, the powder exhibited heterogeneity in terms of size, shape and structure but with a relatively smooth surface. After 30 min of digestion, the powder was swollen; rough surfaces as well as loosened layers of the plant tissues could be observed. More severe damage was observed at a prolonged digestion time. By the end of digestion (180 min), higher degrees of swelling as well as disrupted inner cellular structure were observed. During gastric digestion, acidic pH and enzymatic hydrolysis occurred; this in turn resulted in plant tissue softening.34 Partial solubilization of such soluble components as soluble dietary fiber into the digestive fluid also occurred, leading to changes in the integrity of plant cell walls as well as particle surface and structure and eventually surface disintegration.34–36 Such disruption might help release GLSs from the vegetable cells. Note that food structure disruption may be even more severe in the in vivo system due to the effects of peristaltic movement and gastrointestinal secretions.37,38 This should facilitate the release of bioactive compounds from the plant cells. Confirmation of this hypothesis should be made in a future study.
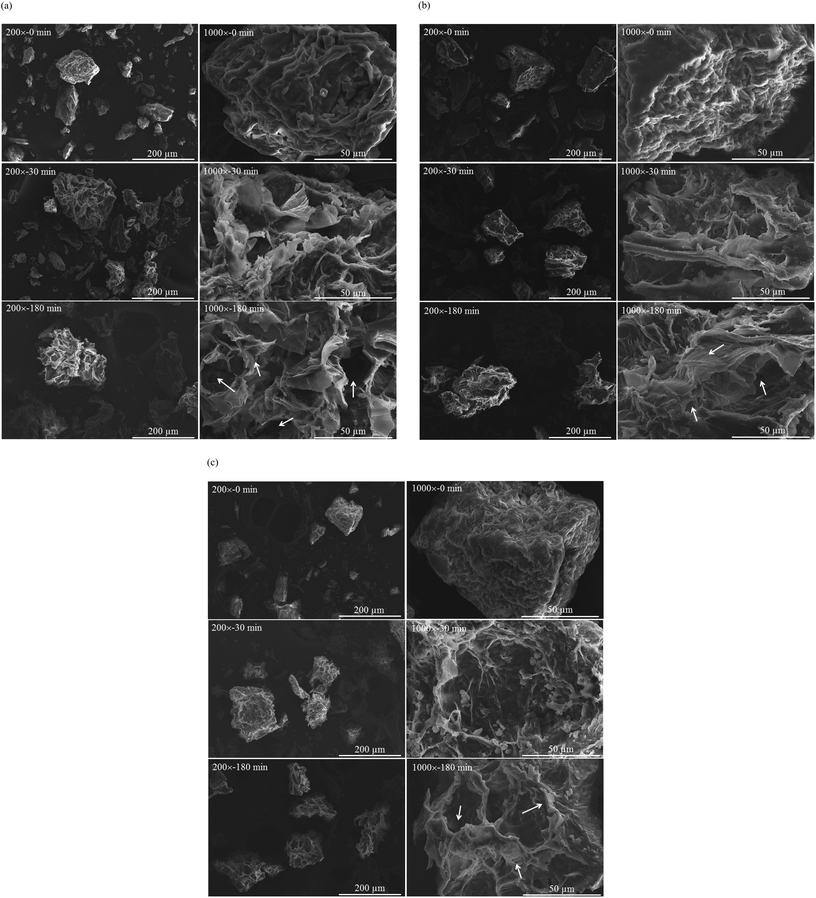 |
| Fig. 3 SEM images of vegetable powder prepared from (a) white cabbage; (b) Chinese cabbage and (c) bok choy before (t = 0 min) and after in vitro gastric digestion for 30 and 180 min at magnifications of 200× and 1000×. The arrows refer to typical xylem vessels and damage of the capillary porous structure. | |
The above results illustrated that glucoraphanin and glucoerucin in the three vegetables can be adequately released during in vitro gastric digestion for up to 180 min, with no losses under the acidic environment. This implied high bioaccessibility of the compounds in subsequent intestinal digestion. Approximately 87%–88% of sinigrin could also be detected in the gastric juice after 3 h digestion. Glucobrassicin was, on the other hand, the most acid-vulnerable GLS and the degree of degradation varied greatly depending on the vegetable type (retention of 59%–76% by the end of digestion experiments). Maskell and Smithard39 reported that the amount of GLSs from rapeseed meal that degraded during in vitro gastric digestion was in the range of 3–23%, depending on the type of GLSs. Previous studies have shown that glucoraphanin, be it semi-purified glucoraphanin from broccoli seeds40 or steamed broccoli,41 was stable throughout the whole in vitro digestion process. Cuomo et al.42 also reported that glucoraphanin from steamed broccoli degraded only by 5% after in vitro digestion. Vegetable powder in the present study showed similar or even higher retention compared to the results of the previous studies, indicating that the matrices of vegetable powder prepared from Brassica vegetables are capable of preserving GLSs during gastric digestion. As such, the powder can serve as a promising functional ingredient with high contents of DF and GLSs.
4. Conclusion
Stabilities of major GLSs in three popular Brassica vegetables during their preparation into vegetable powder were investigated. Steam blanching did not have a significant effect on aliphatic GLSs (i.e., sinigrin, glucoraphanin and glucoerucin) and had only a minute effect on indole GLSs (i.e., glucobrassicin). Drying was noted to be a critical processing step adversely affecting the GLS contents. The overall retention of GLSs in the vegetable powder was 61–80% as compared with the contents in the fresh vegetables. Bioaccessible GLS contents in the vegetable powder during in vitro gastric digestion were then determined. Glucoraphanin and glucoerucin exhibited higher stabilities than sinigrin and glucobrassicin; the two former compounds could be fully accessed after gastric digestion. By comparing the studied vegetables, white cabbage is suggested as an appropriate raw material to produce vegetable powder as it contains high contents of GLSs, especially glucoraphanin and glucoerucin, which possess high thermal and acid stabilities as well as bioaccessibilities. Studies on other health-related functional properties, e.g., glucose and bile acid absorption capacities, as well as the release characteristics of important antioxidants, e.g., flavonoids, during the digestion of the selected vegetable powder are recommended for future work.
Conflicts of interest
The authors declare no conflict of interest.
Acknowledgements
This work was supported by the Natural Science Foundation of China – the Optimized Research on In Vitro Soft-Elastic Rat Stomach Biomimetic Digestive System (General program, grant number 21676172), the Natural Science Foundation of China (Youth program, grant number 31601513), the National Key Research and Development Program of China (project number 2017YFD0400905), Priority Academic Program Development (PAPD) of Jiangsu Higher Education Institutions as well as the Higher Education Research Promotion and the National Research University Project of Thailand, Office of the Higher Education Commission and the Thailand Research Fund (TRF; grant no. RTA6180008). Kuljarachanan thanks the TRF, through the Royal Golden Jubilee (RGJ) Scholarship program, and the King Mongkut's University of Technology Thonburi for supporting her doctoral study.
References
- J. Kapusta-Duch, B. Kusznierewicz, T. Leszczyńska and B. Borczak, Effect of cooking on the contents of glucosinolates and their degradation products in selected Brassica vegetables, J. Funct. Foods, 2016, 23, 412–422 CrossRef CAS.
- R. Verkerk, M. Schreiner, A. Krumbein, E. Ciska, B. Holst, I. Rowland, R. De Schrijver, M. Hansen, C. Gerhauser, R. Mithen and M. Dekker, Glucosinolates in Brassica vegetables: The influence of the food supply chain on intake, bioavailability and human health, Mol. Nutr. Food Res., 2009, 53, S219–S265 CrossRef PubMed.
- A. F. A. Razis, M. Bagatta, G. R. De Nicola, R. Iori and C. Ioannides, Up-regulation of cytochrome P450 and phase II enzyme systems in rat precision-cut rat lung slices by the intact glucosinolates, glucoraphanin and glucoerucin, Lung Cancer, 2011, 71, 298–305 CrossRef PubMed.
- J.-R. Weng, C.-H. Tsai, S. K. Kulp and C.-S. Chen, Indole-3-carbinol as a chemopreventive and anti-cancer agent, Cancer Lett., 2008, 262, 153–170 CrossRef CAS PubMed.
- J. W. Fahey, S. L. Wehage, W. D. Holtzclaw, T. W. Kensler, P. A. Egner, T. A. Shapiro and P. Talalay, Protection of humans by plant glucosinolates: Efficiency of conversion of glucosinolates to isothiocyanates by the gastrointestinal microflora, Cancer Prev. Res., 2012, 5, 603–611 CrossRef CAS PubMed.
- Y. Tanongkankit, N. Chiewchan and S. Devahastin, Physicochemical property changes of cabbage outer leaves upon preparation into functional dietary fiber powder, Food Bioprod. Process., 2012, 90, 541–548 CrossRef CAS.
-
C. Buachap, M. Eng. Thesis in Food Engineering, King Mongkut's University of Technology Thonburi, Bangkok, Thailand, 2015.
- N. Tawatsinlapasorn, T. Kuljarachanan, N. Chiewchan and S. Devahastin, Effects of drying techniques on selected functional properties and bioactive compounds of dietary fiber from the outer leaves of cabbage, Chiang. Mai Univ. J. Nat. Sci., 2017, 16, 19–30 Search PubMed.
- F. Vallejo, A. Gil-Izquierdo, A. Perez-Vicente and C. Garcia-Viguera,
In vitro gastrointestinal digestion study of broccoli inflorescence phenolic compounds, glucosinolates and vitamin C, J. Agric. Food Chem., 2004, 52, 135–138 CrossRef CAS PubMed.
-
AOAC, Official methods of analysis, Association of Official Analytical Chemists, Gaithersburg, 17th edn, 2000 Search PubMed.
- Y. Tanongkankit, N. Chiewchan and S. Devahastin, Effect of processing on antioxidants and their activity in dietary fiber powder from cabbage outer leaves, Drying Technol., 2010, 28, 1063–1071 CrossRef CAS.
- J. A. Larrauri, New approaches in the preparation of high dietary fibre powders from fruit by-products, Trends Food Sci. Technol., 1999, 10, 3–8 CrossRef CAS.
- C. S. Charron, A. M. Saxton and C. E. Sams, Relationship of climate and genotype to seasonal variation in the glucosinolate–myrosinase system. I. Glucosinolate content in ten cultivars of Brassica oleracea grown in fall and spring seasons, J. Sci. Food Agric., 2005, 85, 671–681 CrossRef CAS.
- K. C. Lee, M. W. Cheuk, W. Chan, A. W. Lee, Z. Z. Zhao, Z. H. Jiang and Z. Cai, Determination of glucosinolates in traditional Chinese herbs by high-performance liquid chromatography and electrospray ionization mass spectrometry, Anal. Bioanal. Chem., 2006, 386, 2225–2232 CrossRef CAS PubMed.
- P. Wu, L. Chen, X. Wu and X. D. Chen, Digestive behaviours of large raw rice particles in vivo and in vitro rat stomach systems, J. Food Eng., 2014, 142, 170–178 CrossRef.
- M. Minekus, M. Alminger, P. Alvito, S. Ballance, T. Bohn, C. Bourlieu, F. Carriere, R. Boutrou, M. Corredig, D. Dupont, C. Dufour, L. Egger, M. Golding, S. Karakaya, B. Kirkhus, S. Le Feunteun, U. Lesmes, A. Macierzanka, A. Mackie, S. Marze, D. J. McClements, O. Menard, I. Recio, C. N. Santos, R. P. Singh, G. E. Vegarud, M. S. Wickham, W. Weitschies and A. Brodkorb, A standardised static in vitro digestion method suitable for food - an international consensus, Food Funct., 2014, 5, 1113–1124 RSC.
- P. Pongmalai, N. Fu, S. Soponronnarit, N. Chiewchan, S. Devahastin and X. D. Chen, Microwave pretreatment enhances the formation of cabbage sulforaphane and its bioaccessibility as shown by a novel dynamic soft rat stomach model, J. Funct. Foods, 2018, 43, 186–195 CrossRef CAS.
- H. He, L. Liu, S. Song, X. Tang and Y. Wang, Evaluation of glucosinolate composition and contents in Chinese Brassica vegetables, Acta Hortic., 2003, 620, 85–92 CrossRef CAS.
- M. M. Kushad, A. F. Brown, A. C. Kurilich, J. A. Juvik, B. P. Klein, M. A. Wallig and E. H. Jeffery, Variation of glucosinolates in vegetable crops of Brassica oleracea, J. Agric. Food Chem., 1999, 47, 1541–1548 CrossRef CAS PubMed.
- B. Kusznierewicz, A. Bartoszek, L. Wolska, J. Drzewiecki, S. Gorinstein and J. Namieśnik, Partial characterization of white cabbages (Brassica oleracea var. capitata f. alba) from different regions by glucosinolates, bioactive compounds, total antioxidant activities and proteins, LWT – Food Sci. Technol., 2008, 41, 1–9 CrossRef CAS.
- M. Wiesner, R. Zrenner, A. Krumbein, H. Glatt and M. Schreiner, Genotypic variation of the glucosinolate profile in Pak Choi (Brassica rapa ssp. chinensis), J. Agric. Food Chem., 2013, 61, 1943–1953 CrossRef CAS PubMed.
- M. M. Kushad, R. Cloyd and M. Babadoost, Distribution of glucosinolates in ornamental cabbage and kale cultivars, Sci. Hortic., 2004, 101, 215–221 CrossRef CAS.
- M.-H. Park, M. V. Arasu, N.-Y. Park, Y.-J. Choi, S.-W. Lee, N. A. Al-Dhabi, J. B. Kim and S.-J. Kim, Variation of glucoraphanin and glucobrassicin: Anticancer components in Brassica during processing, Food Sci. Technol., 2013, 33, 624–631 CrossRef.
- B. Yang and C. F. Quiros, Survey of glucosinolate variation in leaves of Brassica rapa crops, Genet. Resour. Crop Evol., 2010, 57, 1079–1089 CrossRef CAS.
- J. K. Kim, S. M. Chu, S. J. Kim, D. J. Lee, S. Y. Lee, S. H. Lim, S.-H. Ha, S. J. Kweon and H. S. Cho, Variation of glucosinolates in vegetable crops of Brassica rapa L. ssp. pekinensis, Food Chem., 2010, 119, 423–428 CrossRef CAS.
- B. Zhu, J. Yang and Z.-j. Zhu, Variation in glucosinolates in Pak Choi cultivars and various organs at different stages of vegetative growth during the harvest period, J. Zhejiang Univ., Sci., B, 2013, 14, 309–317 CrossRef PubMed.
- E. Ciska and H. Kozłowska, The effect of cooking on the glucosinolates content in white cabbage, Eur. Food Res. Technol., 2001, 212, 582–587 CrossRef CAS.
- K. Oerlemans, D. M. Barrett, C. B. Suades, R. Verkerk and M. Dekker, Thermal degradation of glucosinolates in red cabbage, Food Chem., 2006, 95, 19–29 CrossRef CAS.
- S. Chaisamlitpol, B. Hiranvarachat, J. Srichumpoung, S. Devahastin and N. Chiewchan, Bioactive compositions of extracts from cabbage outer leaves as affected by drying pretreatment prior to microwave-assisted extraction, Sep. Purif. Technol., 2014, 136, 177–183 CrossRef CAS.
- F. S. Hanschen, S. Rohn, I. Mewis, M. Schreiner and L. W. Kroh, Influence of the chemical structure on the thermal degradation of the glucosinolates in broccoli sprouts, Food Chem., 2012, 130, 1–8 CrossRef CAS.
- M. Dekker, K. Hennig and R. Verkerk, Differences in thermal stability of glucosinolates in five Brassica vegetables, Czech J. Food Sci., 2009, 27, S85–S88 CrossRef CAS.
- I. Sarvan, R. Verkerk, M. van Boekel and M. Dekker, Comparison of the degradation and leaching kinetics of glucosinolates during processing of four Brassicaceae (broccoli, red cabbage, white cabbage, Brussels sprouts), Innovative Food Sci. Emerging Technol., 2014, 25, 58–66 CrossRef CAS.
- F. S. Hanschen, E. Lamy, M. Schreiner and S. Rohn, Reactivity and stability of glucosinolates and their breakdown products in foods, Angew. Chem., Int. Ed., 2014, 53, 11430–11450 CrossRef CAS PubMed.
- F. Kong and R. P. Singh, Modes of disintegration of solid foods in simulated gastric environment, Food Biophys., 2009, 4, 180–190 CrossRef PubMed.
- F. Kong and R. P. Singh, Solid loss of carrots during simulated gastric digestion, Food Biophys., 2011, 6, 84–93 CrossRef PubMed.
- E. Capuano, The behavior of dietary fiber in the gastrointestinal tract determines its physiological effect, Crit. Rev. Food Sci. Nutr., 2017, 57, 3543–3564 CrossRef CAS PubMed.
- M. M. Grundy, C. H. Edwards, A. R. Mackie, M. J. Gidley, P. J. Butterworth and P. R. Ellis, Re-evaluation of the mechanisms of dietary fibre and implications for macronutrient bioaccessibility, digestion and postprandial metabolism, Br. J. Nutr., 2016, 116, 816–833 CrossRef CAS PubMed.
- A. Lovegrove, C. H. Edwards, I. De Noni, H. Patel, S. N. El, T. Grassby, C. Zielke, M. Ulmius, L. Nilsson, P. J. Butterworth, P. R. Ellis and P. R. Shewry, Role of polysaccharides in food, digestion, and health, Crit. Rev. Food Sci. Nutr., 2017, 57, 237–253 CrossRef CAS PubMed.
- I. Maskell and R. Smithard, Degradation of glucosinolates during in vitro incubations of rapeseed meal with myrosinase (EC 3.2.3.1) and with pepsin (EC 3.4.23.1)-hydrochloric acid, and contents of porcine small intestine and caecum, Br. J. Nutr., 1994, 72, 455–466 CrossRef CAS PubMed.
- R. H. Lai, M. J. Miller and E. Jeffery, Glucoraphanin hydrolysis by microbiota in the rat cecum results in sulforaphane absorption, Food Funct., 2010, 1, 161–166 RSC.
- I. Sarvan, E. Kramer, H. Bouwmeester, M. Dekker and R. Verkerk, Sulforaphane formation and bioaccessibility are more affected by steaming time than meal composition during in vitro digestion of broccoli, Food Chem., 2017, 214, 580–586 CrossRef CAS PubMed.
- V. Cuomo, F. Luciano, G. Meca, A. Ritieni and J. Mañes, Bioaccessibility of glucoraphanin from broccoli using an in vitro gastrointestinal digestion model, CyTA – J. Food, 2014, 13, 361–365 CrossRef.
|
This journal is © The Royal Society of Chemistry 2020 |
Click here to see how this site uses Cookies. View our privacy policy here.