DOI:
10.1039/C9FO01028A
(Paper)
Food Funct., 2020,
11, 1098-1109
Microalgae aqueous extracts exert intestinal protective effects in Caco-2 cells and dextran sodium sulphate-induced mouse colitis†
Received
14th May 2019
, Accepted 2nd December 2019
First published on 2nd December 2019
Abstract
Microalgae are emerging as a good source of natural nutraceuticals. Here, we examined the intestinal protective effects of microalgae aqueous extracts (MAEs) from Chlorella pyrenoidosa, Spirulina platensis, and Synechococcus sp. PCC 7002 in human intestinal epithelial Caco-2 cells and dextran sodium sulphate (DSS)-induced colitis in C57BL/6 mice. MAEs displayed intestinal barrier-protective activities in Caco-2 cells by increasing the expression of heat shock protein (Hsp)-27 and tight junction proteins of occludin and claudin-4 and attenuating the H2O2-induced intracellular reactive oxygen species production, plasma membrane impairment and apoptosis. They also showed anti-inflammatory potential in tumor necrosis factor (TNF)-α-, interleukin (IL)-1β- and H2O2-stimulated Caco-2 cells by suppressing the secretion of IL-8 and the expression of cyclooxygenase 2 (COX-2) and inducible nitric oxide synthase (iNOS). The 8 d daily intragastric administration of MAEs during and after 4 d DSS exposure effectively alleviated colitis symptoms of weight loss, diarrhea, rectal bleeding, and colon shortening and histopathology, protected intestinal barrier function by increasing colonic Hsp-25, occludin and claudin-4, and attenuated colonic and systemic inflammation by suppressing colonic myeloperoxidase activity, production of TNF-α, IL-1β and IL-6, expression of COX-2 and iNOS, and peripheral leukocytosis, monocytosis and granulocytosis. Microalgae can thus serve as a functional food to maintain gut health.
Introduction
Inflammatory bowel diseases (IBD) including Crohn's disease and ulcerative colitis are gastrointestinal disorders involving complex interactions of genetic, immunologic, environmental and microbiota factors. IBD affect more than 4 million people in the Western countries and are increasingly prevalent in Asian countries keeping pace with “Westernization” of society.1 The hallmark of IBD is chronic, uncontrolled inflammation of the intestinal mucosa. IBD display various symptoms depending on the severity of inflammation. These symptoms mainly include reduced appetite, fever, fatigue, abdominal pain and cramping, diarrhea, and bloody stool. Conventional IBD therapies, such as with 5-aminosalicylic acid, sulphasalazine, corticosteroids and anti-tumor necrosis factor (TNF)-α antibodies, have various side effects, and are not effective in all patients.2 Attention has therefore been devoted to developing alternative remedies against IBD based on natural ingredients in recent years.3
Microalgae are a group of diverse unicellular organisms existing individually or in groups. They are ubiquitously distributed throughout the biosphere and can survive a variety of extreme conditions. Their wide ecological adaptation provides an opportunity for their genetic and metabolic diversity and thus capacities to produce a wide spectrum of bioactive metabolites. As a consequence, growing studies have been conducted on microalgae for their potential to promote human health.4Chlorella is a green alga that has been utilized since 1950s for its high nutritive value and health benefits. As a filamentous cyanobacterium, Spirulina has been used as a dietary supplement and the primary source of phycocyanin, a natural coloring agent with well-documented nutraceutical potential.5Chlorella and Spirulina contribute to the majority of commercial microalgal biomass.6Synechococcus is one of the two predominant cyanobacterial genera across the ocean, and a coastal strain PCC 7002 exhibits promising potential to produce bioactive compounds owing to its ultrafast growth and good tolerance to a wide spectrum of salinity, temperature and irradiation conditions.7,8
Several recent studies have renewed interest in the protective potential of microalgae under diverse disease conditions such as diabetes, hepatic injury, and neurodegeneration.9,10 Microalgal biomass and extracts have been found to alleviate IBD symptoms in acetic acid- and 2,4,6-trinitrobenzene sulphonic acid-induced murine models and human clinical trials.11–14 In this sense, supplementing the diet with microalgal biomass or extracts is a promising strategy to improve the quality of life of IBD patients. Further research is needed to test the anti-IBD activities of more microalgal species and to elucidate the underlying mechanism. To the best of our knowledge, microalgae have not been examined for their beneficial properties in human intestinal epithelial cells and in dextran sulphate sodium (DSS)-induced acute colitis. The present study aimed to estimate the protective potential of aqueous extracts from three microalgae, i.e. Chlorella pyrenoidosa (C. pyrenoidosa), Spirulina platensis (S. platensis) and Synechococcus sp. PCC 7002 (Synechococcus 7002), in Caco-2 cells and DSS-induced mouse colitis.
Experimental section
Reagents
Dimethyl sulfoxide (DMSO), methylthiazolyldiphenyl-tetrazolium bromide (MTT), hydrogen peroxide (H2O2), radio immunoprecipitation assay (RIPA) lysis buffer, phenylmethanesulfonyl fluoride (PMSF), 2′,7′-dichlorodihydrofluorescein diacetate (DCFH-DA), a lactic dehydrogenase (LDH)-based in vitro toxicology assay kit (4744934001), and the primary antibody anti-mouse β-actin (R1011) were purchased from Sigma-Aldrich Co. (Shanghai, China). Recombinant human TNF-α and IL-1β, a Pierce™ BCA protein assay kit, phosphate-buffered saline (PBS), an enhanced chemiluminescence (ECL) detection kit and sandwich enzyme-linked immunosorbent assay (ELISA) kits for human IL-8 and mouse interleukin (IL)-6, IL-1β and TNF-α were provided by Thermo Fisher Scientific Co., Ltd (Shanghai, China). Dulbecco's modified Eagle's medium (DMEM) and fetal bovine serum (FBS) were obtained from Biological Industries (Beth Haemek, Israel). The primary antibodies anti-mouse heat shock protein 25 (Hsp-25) (ab202846), heat shock protein 27 (Hsp-27) (ab5579), anti-human claudin-4 (ab15104), anti-human occludin (ab167161), anti-human cyclooxygenase 2 (COX-2) (ab15191), anti-human inducible nitric oxide synthase (iNOS) (ab202417) and horseradish peroxidase (HRP)-conjugated secondary antibodies of anti-mouse IgG (ab6728) and anti-rabbit IgG (ab6721) were acquired from Abcam PLC. (MA, USA). Sodium dodecyl sulfate (SDS)-polyacrylamide gel electrophoresis (PAGE) sample loading buffer (5×) was supplied by Beijing Solarbio Science & Technology Co., Ltd. DSS (molecular weight, 36–50 kDa) was purchased from Yisheng Biotechnology Co., Ltd (Shanghai, China). Other reagents used were of analytical grade and commercially available.
Preparation and characterization of microalgae aqueous extracts (MAEs)
Food-grade powders of C. pyrenoidosa and S. platensis were purchased from Fuqing King Dnarmsa Spirulina Co., Ltd (Fuqing, China). Synechococcus 7002 was cultured in medium A under aeration conditions with filtered air and 100 μmol m−2 s−1 irradiation at 32 °C for 10 days,15 harvested by centrifugation at 6000g for 5 min, washed three times with distilled water, and lyophilized as an algal powder.
MAEs were prepared according to the procedure described by Lee et al. (2017).16 Microalgal powders were extracted with 10 volumes of distilled water at 40 °C for 8 h under magnetic stirring, followed by centrifugation at 8000g for 10 min, and the supernatants were lyophilized as MAEs. The samples were stored in air-tight amber bottles at −20 °C before use. MAEs from C. pyrenoidosa, S. platensis and Synechococcus 7002 are denoted as ChAE, SpAE and SyAE, respectively, in the following sections.
Protein contents in MAEs were determined using the BCA assay kit as per the manufacturer's instructions. Total carbohydrate was assayed by the phenol-sulfuric acid method.17 The amounts of phenolic compounds in MAEs were measured with the Folin–Ciocalteu method.18 The phycobiliproteins of phycocyanin (PC), allophycocyanin (APC) and phycoerythrin (PE) were measured spectroscopically at 652, 615 and 562 nm, respectively.19
Cellular experiments
Caco-2 cells were provided by the Cell Bank of Chinese Academy of Sciences (Shanghai, China) and routinely maintained in 10% FBS-supplemented DMEM. The passage number of cells used was between 55 and 65 and the cell culture medium was changed every 2–3 days. All cultures were incubated at 37 °C under a humidified atmosphere of 5% CO2 and 95% air.
To assay the expression of Hsp-27 and tight junction proteins, wells were set up with each containing 5 × 105 cells in a 12-well plate. The cells were cultured for 12 d before treatment with MAEs (8 μg mL−1) in complete medium for 24 h, and were then lysed in RIPA buffer containing 1 mM PMSF at 4 °C for 30 min. After centrifugation at 12
000g for 15 min at 4 °C, the supernatant was used as the whole cell lysate for western blotting analysis.
Intracellular reactive oxygen species (ROS) were assayed using the oxidant-sensitive dye DCFH-DA. Briefly, wells were set up with each containing 1 × 104 cells in a 96-well plate. The cells were incubated for 72 h before treatment with MAEs (8 μg mL−1) in complete medium for 24 h, and were then loaded with 10 μM DCFH-DA in HBSS for 40 min. After rinsing of the fluorescent dye, the DCF-loaded cells were stimulated with 500 μM H2O2 for 30 min, and the fluorescence was then determined with excitation at 485 nm and emission at 528 nm in a Synergy 2 microplate reader (Bio-Tek, VT, USA).
To assay LDH release, wells were set up with each containing 1 × 104 cells in a 96-well plate. The cells were cultured for 72 h and treated with 8 μg mL−1 MAEs in complete medium for another 24 h. After a triple rinse in DPBS, the cells were stimulated with 500 μM H2O2 for 60 min, and the culture supernatant was harvested for LDH activity assay using a commercial kit according to the instructions of the manufacturer.
To assay oxidant-induced apoptosis, wells were set up with each containing 1 × 104 cells in a 96-well plate. The cells were cultured for 72 h and treated with 8 μg mL−1 MAEs in complete medium for another 24 h. After a triple rinse in DPBS, the cells were stimulated with 1.5 mM H2O2 for 60 min. The MTT solution (0.5 mg mL−1 in DMEM) was then added and the plate was incubated for 4 h. Following medium removal, 150 μL DMSO was added to each well and the plate was agitated for 10 min. The absorbance was then measured at 570 nm.
To determine the anti-inflammatory activities of MAEs, Caco-2 cells were seeded on 24-well plates at a density of 1 × 105 cells per well. The cells were incubated for 12 d before treatment with MAEs (8 μg mL−1) in complete medium for 24 h, and were then stimulated with TNF-α (10 ng mL−1), IL-1β (10 ng mL−1) or H2O2 (500 μM) for 24 h, 16 h and 0.5 h, respectively. The supernatant was collected and analyzed for IL-8 using an ELISA kit as per the manufacturer's instructions. The cells were lysed in RIPA buffer containing 1 mM PMSF at 4 °C for 30 min, and after centrifugation at 12
000g for 15 min at 4 °C, the supernatant was used as the whole cell lysate for western blotting analysis of COX-2 and iNOS.
Animals
Male 6–7-week-old C57BL/6 mice (weighing 19.0–21.0 g) were obtained from Lukang Pharmaceutical Co. (Shandong, China). All mice were individually housed in an air-conditioned room maintained at 20–24 °C with 55%–65% relative humidity and a 12 h light
:
12 h dark lighting schedule. The animals were fed on an NIH-41 standard diet (Trophic Animal Feed High-Tech Co., Ltd, Nantong, China) ad libitum. All experiments were performed in accordance with the principles of the National Institutes of Health (NIH) Guide for the Care and Use of Laboratory Animals, and were approved by the Committee on the Ethics of Animal Experiments of Ocean University of China (no. SPXY20180929).
Colitis induction protocol
Following a 5 d acclimation period, the mice were randomized into five groups (n = 8 for each group), comprising normal control, DSS control, ChAE + DSS, SpAE + DSS, and SyAE + DSS groups. The normal control group received deionized water ad libitum throughout the 8 d experimental period, while the other groups received 3% DSS solution (w/v) for 4 d before changing to deionized water for the remaining 4 d (Fig. 3a). All mice received daily intragastric intubation, consisting of 200 μL PBS for the normal and DSS control groups and 200 μL PBS containing 2 mg mL−1 MAEs for the MAE-treated groups, for 8 d until sacrificed.
Clinical evaluation of colitis
Food intake, body weight, and colitis symptoms (i.e. diarrhea and rectal bleeding) were recorded daily. To evaluate the severity of colitis, an ulcerative colitis disease activity index (DAI) was calculated as the sum scores of stool consistency, rectal bleeding and body weight loss, which were scored following the criteria listed in Table S1.†
20
Animal sacrifice
At the end of the experimental period, the animals were euthanized by cervical dislocation. Blood samples were collected on ethylenediaminetetraacetic acid and analyzed for hematological parameters on an XN-9000 hematology analyzer (Sysmex, Kobe, Japan). The entire colon was removed from the abdominal cavity and the length of each colon was immediately measured at rest, without stretching. Following a thorough rinse with PBS, the colon was cut into small pieces (0.5 or 1 cm long).
Histopathological and immunofluorescence analysis
A 0.5 cm piece of the distal colon was fixed in 4% paraformaldehyde and dehydrated in a series of ethanol, before being embedded in paraffin. The embedded tissue was cut into 5 μm thin sections. For histopathology, the sections were stained with hematoxylin and eosin (H&E), and scanned under a Pannoramic MIDI II automatic digital slide scanner (3DHISTECH, Budapest, Hungary), followed by a blind scoring of histologic lesions as described previously.21 For immunofluorescence, after deparaffinization using xylene and rehydration in water, the sections were blocked with 5% bovine serum albumin and incubated sequentially with primary antibodies for 12 h at 4 °C and an Alexa Fluor 647-labeled secondary antibody at room temperature for 1 h in the dark, followed by fluorescence visualization using a Nikon Eclipse Ti-SR microscope with a Nikon DS-U3 digital camera (Tokyo, Japan).
Measurement of myeloperoxidase (MPO) activity
The MPO activity was determined according to a method reported earlier.22 A pre-weighed piece of the proximal colon was homogenized in ice-cold PBS containing 5% hexadecyl trimethyl ammonium bromide with a KZ-II TissueLyser (Servicebio, Wuhan, China). The homogenate samples were centrifuged at 13
000g for 5 min at 4 °C, and 10 μL of the resulting supernatant was mixed with 200 μL of an active dianisidine substrate comprising 0.2 mg mL−1o-dianisidine hydrochloride and 0.002% H2O2 on an ELISA plate. The change in absorbance at 450 nm was measured immediately with a microplate reader. MPO activity was expressed in units per milligram of wet tissue. The MPO assay was performed in 48 h once the animals were necropsied.
Quantification of cytokine and protein expression in the colon
Colon samples were homogenized in 25 volumes of RIPA lysis buffer containing protease inhibitors with the KZ-II TissueLyser. The homogenate was centrifuged at 4 °C for 10 min at 10
000g. The resulted supernatant was used as the tissue extract. TNF-α, IL-1β and IL-6 in the tissue extract were measured using the ELISA kits according to the manufacturer's instructions. Hsp-25 (Hsp-27 in humans), claudin-4, occludin, COX-2 and iNOS in the tissue extract were quantified by western blotting analysis.
Western blotting
Protein concentrations in the whole cell lysate and the tissue extract were determined with the Pierce BCA protein assay kit according to the manufacturer's instructions. The samples were denatured in SDS-loading buffer, separated by SDS–PAGE and blotted onto a polyvinylidene fluoride (PVDF) membrane. After being blocked with 5% bovine serum albumin in Tris-buffered saline containing 0.1% Tween-20 (TBST) for 1 h at room temperature, the membrane strips were incubated with primary antibodies at appropriate concentrations for 12 h at 4 °C. A second incubation was performed with HRP-conjugated secondary antibodies at room temperature for 1 h. Chemiluminescence was observed using an ECL detection kit and detected on a Tanon-5200 Multi automatic image analyzer (Tanon, Shanghai, China).
Statistical analysis
The results were statistically analyzed using IBM SPSS Statistics for Windows version 19.0 (IBM Corp., NY, USA) and Origin version 8.0 (OriginLab, Northampton, USA). Data were presented as means ± standard deviations and compared by one-way analysis of variance (ANOVA) followed by Tukey's post hoc test. Significant differences were defined at p-values of p < 0.05.
Results
Chemical composition of the MAEs
The chemical compositions of raw powders (Fig. 1a) and aqueous extracts (Fig. 1b) of C. pyrenoidosa, S. platensis, and Synechococcus 7002 are shown in Table S2† and Table 1, respectively. Synechococcus 7002 contained a comparable protein level to those in C. pyrenoidosa and S. platensis that are well known protein-rich microalgae. Total protein contents in SpAE and SyAE were increased by about 10% compared with those in raw powders of S. platensis and Synechococcus 7002; so protein enrichment seems to occur during aqueous extraction of these two cyanobacteria. Over one-fold enrichment of carbohydrate was observed during aqueous extraction of C. pyrenoidosa.
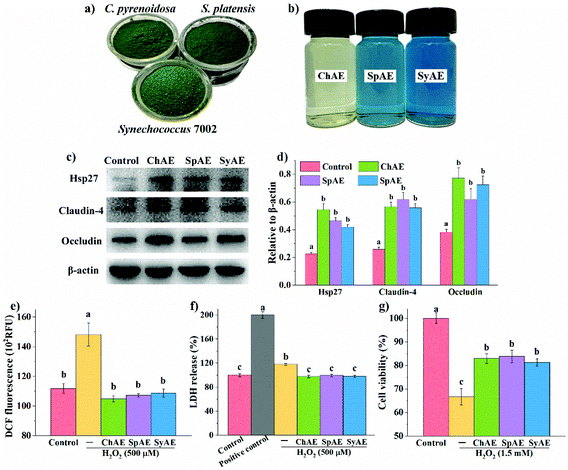 |
| Fig. 1 Beneficial effects of MAEs on the intestinal barrier function in Caco-2 cells: the appearance of (a) microalgal powders and (b) MAEs; (c) representative images and (d) densitometry of western blotting analysis (n = 3); the oxidant-induced (e) intracellular ROS (n = 6), (f) LDH release (n = 6) and (g) apoptosis (n = 6). Data were expressed as means ± standard deviations. Different superscript letters (a–c) denote statistically significant differences (p < 0.05). Abbreviations: MAEs, microalgae aqueous extracts; ROS, reactive oxygen species; and LDH, lactate dehydrogenase. | |
Table 1 Chemical composition of microalgae aqueous extracts
|
Protein |
Total carbohydrate |
Total phenols |
Total protein |
PC |
APC |
PE |
Data are expressed in % of dry weight (means ± standard deviations, n = 3). Different superscript letters (a–b) denote statistically significant differences in the same column (p < 0.05). Abbreviations: ChAE, aqueous extracts of Chlorella pyrenoidosa; SpAE, aqueous extracts of Spirulina platensis; SyAE, aqueous extracts of Synechococcus sp. PCC 7002; PC, phycocyanin; APC, allophycocyanin; and PE, phycoerythrin. |
ChAE |
53.7 ± 1.42b |
— |
— |
— |
33.1 ± 1.08a |
1.59 ± 0.01a |
SpAE |
77.5 ± 2.12a |
14.9 ± 1.18b |
6.25 ± 0.67a |
0.93 ± 0.07a |
11.8 ± 1.14b |
0.99 ± 0.01b |
SyAE |
74.9 ± 2.56a |
20.6 ± 0.22a |
5.39 ± 0.07b |
0.35 ± 0.01b |
11.6 ± 0.93b |
1.06 ± 0.02b |
The total protein contents in SpAE and SyAE were about 40% higher than that in ChAE. SpAE and SyAE contained over 20% dry mass of phycobiliproteins (i.e. PC, APC and PE) that were not present in ChAE. Compared to SpAE, SyAE contained a 23% higher level of the blue-colored phycobiliproteins (i.e. PC and APC), which was in line with the evident bluer appearance of SyAE than that of SpAE (Fig. 1b). ChAE contained about twice more carbohydrate and 50% more phenolic compounds than SpAE and SyAE.
Beneficial effects of MAEs on intestinal barrier function in Caco-2 cell monolayers
The leakage of the intestinal epithelial barrier due to loss of tight junction proteins or injured intestinal epithelial cells can result in intestinal and systemic inflammatory complications. Based on the results of MTT tests shown in Fig. S1,† 8 μg mL−1 of ChAE, SpAE and SyAE had no significant cytotoxicity against Caco-2 cells, and this dose of MAEs was used in the cellular experiments below. Western blotting analysis (Fig. 1c and d) revealed that ChAE, SpAE and SyAE significantly elevated the expression of a cytoprotective protein Hsp-27 and two typical tight junction proteins, i.e. claudin-4 and occludin, in Caco-2 cell monolayers, indicating a role of MAEs in the enhancement of intestinal stress tolerance and tight junction integrity.
Microalga-derived phycobiliproteins, polysaccharides and phenolic compounds have been well documented as good antioxidants.23 In this study, the 0.5 h treatment with 500 μM H2O2 caused significantly increased DCF fluorescence in Caco-2 cells (Fig. 1e), and this was completely prevented by the pretreatments of the cells with ChAE, SpAE and SyAE for 24 h. These results suggest that MAEs were effective at attenuating the H2O2-induced burst of intracellular ROS.
The epithelial plasma membrane is prone to ROS attack during intestinal pathological processes. As shown in Fig. 1f, the treatment with 500 μM H2O2 for 1 h caused evident leakage of cytosolic LDH, and this was completely avoided by the 24 h pretreatments of cells with ChAE, SpAE and SyAE. These results suggest a protective role of MAEs against oxidant-induced membrane leakage of epithelial cells.
As shown in Fig. 1g, Caco-2 cells suffered an average 32% loss of viability following the treatment with 1.5 mM H2O2 for 1 h, and this was significantly attenuated by the 24 h preincubation with ChAE, SpAE and SyAE (p < 0.05). These results suggest that MAEs exerted cytoprotective effects against oxidant-induced epithelial apoptosis.
Anti-inflammatory effects of MAEs in Caco-2 cells
In response to pro-inflammatory cytokines or oxidative stress, intestinal epithelial cells can directly modulate host immune responses by secreting immunological mediators especially IL-8.24 As shown in Fig. 2a–c, the treatments of Caco-2 cells with TNF-α, IL-1β and H2O2 for 24 h, 16 h, and 0.5 h, respectively, significantly stimulated IL-8 production. These effects were remarkably attenuated by the 24 h pretreatments with ChAE, SpAE and SyAE (p < 0.05), suggesting an anti-inflammatory role of these MAEs in intestinal epithelial cells.
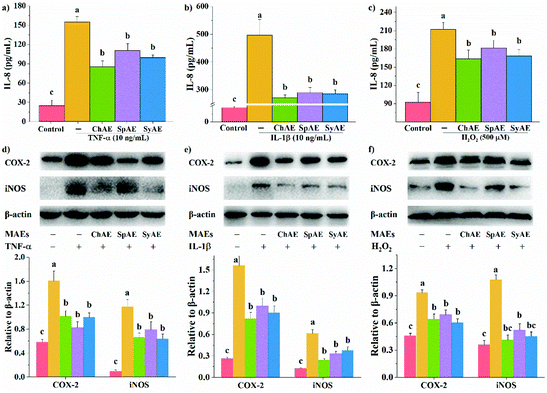 |
| Fig. 2 Anti-inflammatory effects of MAEs in Caco-2 cells: IL-8 production when stimulated with (a) TNF-α, (b) IL-1β and (c) H2O2; western blotting analysis of the expression of COX-2 and iNOS when stimulated with (d) TNF-α, (e) IL-1β and (f) H2O2. Data are expressed as means ± standard deviations (n = 4). Different superscript letters (a–c) denote statistically significant differences (p < 0.05). Abbreviations: MAEs, microalgae aqueous extracts; IL, interleukin; TNF, tumor necrosis factor; COX-2, cyclooxygenase-2; and iNOS, inducible nitric oxide synthase. | |
COX-2 and iNOS are important contributors to the cascade of epithelial inflammatory responses induced by certain pathogens or diseases. In the present study, we observed increased expression of COX-2 and iNOS in Caco-2 cells in response to TNF-α, IL-1β and H2O2 (Fig. 2d–f). When pretreated with ChAE, SpAE and SyAE, the cells were significantly less sensitive to these stimulants (p < 0.05). These results implied the anti-inflammatory potential of MAEs in intestinal epithelial cells again.
MAEs suppressed colitis in DSS-administered mice
MAEs were administered at the dose of 200 mg per kg body weight by oral gavage to the DSS-treated mice for 8 d to assess their potential therapeutic efficacy on acute colitis. The human equivalent dose of MAEs was calculated to be 854 mg for a 60 kg human,25 and considering the extraction yield of about 20% (data not shown), this dose corresponds to 4.27 g microalgal biomass per day. C. pyrenoidosa and S. platensis are “generally recognized as safe” for human intake, and their acceptable daily intake doses are set at 5–10 g.26,27 The whole genome of Synechococcus 7002 revealed no known toxic genes.28
During the experiment period, the DSS-treated mice had a total food intake of 21.1 ± 1.2 g per mouse, which was significantly lower than that of the normal controls (26.7 ± 0.3 g per mouse) (p < 0.05). The mice treated with MAEs had increased total food intake (i.e. 23.0 ± 1.1, 22.4 ± 1.5 and 22.7 ± 1.3 g per mouse for the groups of ChAE + DSS, SpAE + DSS and SyAE + DSS, respectively) over the DSS controls.
The mice in the DSS control group began to show significant weight loss compared to the normal control animals from day 5 (p < 0.05) (Fig. 3b). The MAE-treated mice had notably less weight loss than the DSS controls from day 6 (p < 0.05) (Fig. 3b). The DSS treatment caused a rise in the DAI from day 3 (p < 0.05), and this was attenuated by oral administration of ChAE and SpAE from day 4 (p < 0.05) and SyAE from day 6 (p < 0.01) (Fig. 3c), indicating the amelioration of colitis symptoms in mice treated with MAEs.
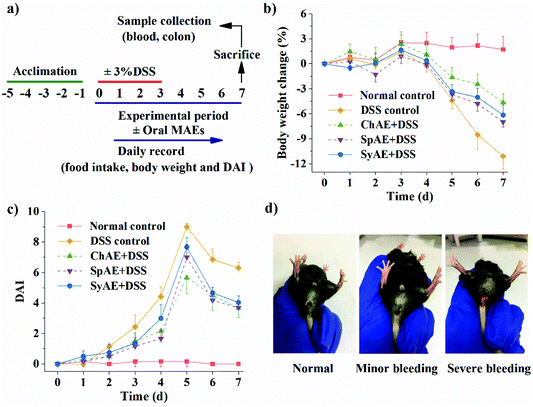 |
| Fig. 3 Clinical symptoms of DSS-induced mouse colitis: (a) experimental design; (b) body weight changes; (c) DAI; and (d) representative images of rectal bleeding. Data are expressed as means ± standard deviations (n = 8). Abbreviation: DAI, disease activity index. | |
The colon length is a biological marker for the severity of acute colitis in mice. Fig. 4a and b show that the DSS-induced colon shortening is effectively prevented by the supplemental MAEs. Histological examination of H&E-stained colon tissues (Fig. 4d) revealed typical colitis lesions (e.g. crypt damage, edema, mucosal ulceration, and infiltration of inflammatory cells) in the DSS control mice, while those treated with MAEs displayed less remarkable histological lesions, which was evidenced by the significantly lower histopathological scores of the groups of ChAE + DSS, SpAE + DSS and SyAE + DSS compared to that of the DSS control group (Fig. 5c). These results suggest that MAEs could effectively ameliorate the DSS-induced colonic injury.
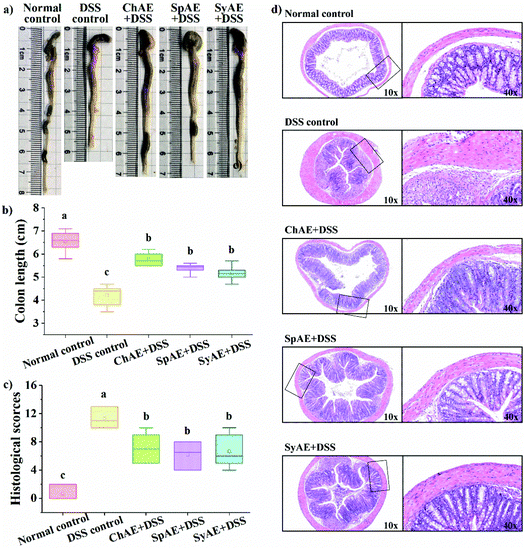 |
| Fig. 4 Effects of orally administered ChAE, SpAE and SyAE on histological symptoms of the colon: (a) typical images of colon length measurements; (b) colon length; (c) histological scores and (d) typical images of H&E stained colonic sections. Right side images (40×) in (d) are the high magnification views of the frame regions of left side images (10×). Data were expressed as means ± standard deviations (n = 8). Different superscript letters (a–c) denote statistically significant differences (p < 0.05). Abbreviations: ChAE, aqueous extracts of Chlorella pyrenoidosa; SpAE, aqueous extracts of Spirulina platensis; and SyAE, aqueous extracts of Synechococcus sp. PCC 7002. | |
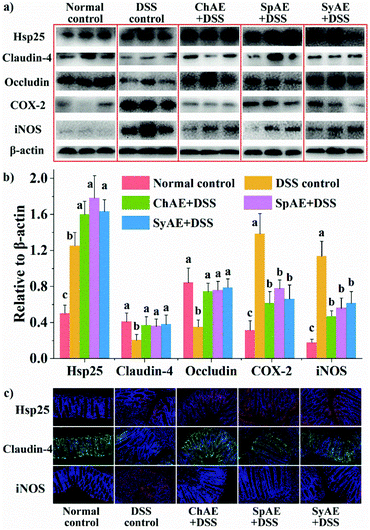 |
| Fig. 5 Effects of orally administered ChAE, SpAE and SyAE on the colonic levels of Hsp, tight junction proteins and inflammatory mediators in DSS-induced colitis mice: western blotting (a) imaging and (b) densitometric analysis; and (c) immunofluorescence microscopy. Images in (a) and (c) are representative of 8 animals in each group. Densitometry results in (b) are expressed as means ± standard deviations (n = 8). Different superscript letters (a–c) denote statistically significant differences (p < 0.05). Abbreviations: ChAE, aqueous extracts of Chlorella pyrenoidosa; SpAE, aqueous extracts of Spirulina platensis; SyAE, aqueous extracts of Synechococcus sp. PCC 7002; and DSS, dextran sodium sulphate. | |
Based on the above results of weight loss, DAI, colon length and histopathology, ChAE, SpAE and SyAE exhibited evident and similar anti-colitis activities. DSS drinking has been reported to lead to a “leaky” epithelial barrier, and as a result, luminal antigens or microorganisms would stimulate inflammatory responses after invading lamina propria and systemic circulation.29–31 In the following sections, to elucidate the anti-colitis mechanism of MAEs, we will examine their gut barrier-protecting and anti-inflammatory potential in DSS-treated mice.
MAEs protected intestinal barrier function in DSS-induced colitis
Heat shock proteins (Hsp) protect intestinal epithelial cells from stressful conditions including heat stress, infection, and inflammation.32 The results of western blotting (Fig. 5a and b) and immunofluorescence microscopy (Fig. 5c) revealed increased colonic Hsp-25 in DSS control-treated mice compared to normal control-treated ones, confirming the stress-responsive expression of cytoprotective Hsp,33 and the oral treatments with ChAE, SpAE and SyAE notably stimulated the expression of more colonic Hsp-25 in DSS-induced colitis mice.
Tight junction proteins between lumen-lining cells play crucial roles in the maintenance of the gut barrier function.34 According to the results of western blotting analysis (Fig. 5a and b), the DSS control mice had reduced colonic levels of claudin-4 and occludin compared to the normal controls, while orally administered ChAE, SpAE and SyAE effectively prevented the DSS-induced loss of these tight junction proteins in the colon. Immunofluorescence microscopy (Fig. 5c) also revealed the preservation of claudin-4 at intestinal epithelial tight junctions by the oral treatments with ChAE, SpAE and SyAE in DSS-induced colitis mice.
According to the above results of colonic Hsp-25 and tight junction proteins, MAEs could protect the gut barrier function in acute colitis by enhancing intestinal stress tolerance and preserving tight junction integrity. These in vivo findings aligned well with those of our in vitro study using Caco-2 cell monolayers (Fig. 1c and d). It thus seems that gut barrier protection may contribute to the anti-colitis potential of MAEs.
MAEs attenuated colonic and systemic inflammation in DSS-induced colitis mice
MPO is a heme-containing lysosomal enzyme expressed mainly in neutrophils, and generates reactive halide and nitrogen that could impair host tissue during intestinal inflammation in IBD.35 As shown in Fig. 6a, the supplemental MAEs significantly attenuated the DSS-induced increase in colonic MPO activity.
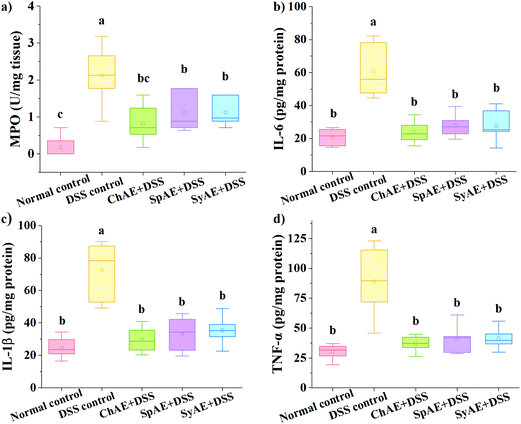 |
| Fig. 6 Effects of orally administered ChAE, SpAE and SyAE on the colonic levels of (a) MPO activity, (b) TNF-α, (c) IL-6 and (d) IL-1β in DSS-induced colitis mice. Data were expressed as means ± standard deviations (n = 8). Different superscript letters (a–c) denote statistically significant differences (p < 0.05). Abbreviations: ChAE, aqueous extracts of Chlorella pyrenoidosa; SpAE, aqueous extracts of Spirulina platensis; SyAE, aqueous extracts of Synechococcus sp. PCC 7002; MPO, myeloperoxidase; TNF, tumor necrosis factor; and IL, interleukin. | |
Pro-inflammatory cytokines of TNF-α, IL-1β and IL-6 have been abundantly found in the colonic tissues of IBD patients and animal models.36 In this study, the DSS control group displayed approximately 3–4 times higher levels of colonic TNF-α, IL-1β and IL-6 than the normal control group. The treatments with ChAE, SpAE and SyAE greatly suppressed the DSS-induced burst of these pro-inflammatory cytokines in the colon (p < 0.05) (Fig. 6b–d).
The colonic levels of COX-2 and iNOS are shown in Fig. 5. We observed greatly increased expression of COX-2 and iNOS in the DSS control mice than in the normal controls, and the treatments with ChAE, SpAE and SyAE effectively prevented the DSS-induced increase in these pro-inflammatory mediators in the colon (Fig. 5a and b). Immunofluorescence microscopy confirmed the down-regulation of colonic iNOS by MAEs in DSS-induced colitis mice (Fig. 5c).
Hematologic parameters have diagnostic importance in detecting systemic inflammation. As shown in Table 2, the DSS control group displayed an about twice higher level of white blood cells (WBC) than the normal control group (p < 0.01), and among WBC, monocytes and granulocytes were especially sensitive to DSS administration, showing approximately 5- and 7-times higher levels, respectively, in the DSS control group than in the normal control group. MAEs greatly suppressed the DSS-induced elevation of peripheral levels of WBC, monocytes and granulocytes.
Table 2 Hematological parameters in blood samples from mice
|
WBC (109 L−1) |
Monocytes (109 L−1) |
Granulocytes (109 L−1) |
Data are expressed as means ± standard deviations (n = 8). Different superscript letters (a–b) denote statistically significant differences in the same column (p < 0.05). Abbreviations: ChAE, aqueous extracts of Chlorella pyrenoidosa; SpAE, aqueous extracts of Spirulina platensis; SyAE, aqueous extracts of Synechococcus sp. PCC 7002; DSS, dextran sodium sulphate; and WBC, white blood cells. |
Normal control |
2.71 ± 0.40b |
0.1 ± 0.13b |
0.26 ± 0.12b |
DSS control |
5.22 ± 1.35a |
0.47 ± 0.17a |
1.88 ± 1.10a |
ChAE + DSS |
2.86 ± 0.74b |
0.06 ± 0.03b |
0.4 ± 0.13b |
SpAE + DSS |
2.84 ± 0.90b |
0.1 ± 0.05b |
0.29 ± 0.16b |
SyAE + DSS |
3.27 ± 0.66b |
0.26 ± 0.13b |
0.31 ± 0.16b |
Based on the above results of biochemical and hematological parameters, MAEs exerted inhibitory effects on both colonic and systemic inflammation in colitis mice. These in vivo findings were in line with the anti-inflammatory potential of MAEs in Caco-2 cells (Fig. 2). It thus seems that MAEs attenuate DSS-induced colitis at least in part via anti-inflammatory activities.
Discussion
Phycobiliproteins are mainly present in cyanobacteria and red algae, and as more primitive participators of photosynthesis, they are replaced by chlorophyll proteins in Chlorella and higher plants.37 Phycobiliproteins are pigment proteins with good water solubilities, while most chlorophyll proteins are water insoluble.38 It is thus no surprise that protein enrichment occurred during aqueous extraction of S. platensis and Synechococcus 7002, rather than C. pyrenoidosa. Compared to green algae, cyanobacteria generally have less complex cell walls,39 which are structurally composed mainly of polysaccharides, and this might be the reason for the relatively lower carbohydrate contents in raw powders and aqueous extracts of S. platensis and Synechococcus 7002 compared to that of C. pyrenoidosa. Despite their varied chemical compositions, ChAE, SpAE and SyAE displayed similar gut barrier-protecting and anti-inflammatory activities in Caco-2 cells and DSS-induced mouse colitis. MAEs thus seem to exert intestinal protective effects by a combined action of protein, carbohydrate and phenols.
Oxidative stress plays a key role in IBD pathogenesis, and antioxidants from natural sources are believed to protect the colon against ulcers by improving antioxidant nutrition.40 Microalgae are a rich source of antioxidants, and when consumed whole, they can exert antioxidative effects in animal models of various diseases.41 The aqueous extract of S. platensis has also been reported to play an antioxidative role in protecting against cell death induced by free radicals in mouse fibroblast cells.42 In the present study, ChAE, SpAE and SyAE displayed evident antioxidant potential in Caco-2 cells according to their prevention of H2O2-induced intracellular oxidant burst (Fig. 1e), cell membrane damage (Fig. 1f) and apoptosis (Fig. 1g). We thus propose that antioxidative protection should be one of the intestinal protective mechanisms of MAEs.
Phycocyanin from cyanobacterial algae has been well-documented for its potential to scavenge several ROS in vitro, and can protect against oxidative stress-induced apoptosis in a variety of cellular models (e.g. cardiomyocytes, erythrocytes, cerebellar granule cells and neuroblastoma cells).43–46 Polysaccharides derived from several microalgae such as S. platensis, C. pyrenoidosa, Dunaliella salina and Porphyridium cruentum have also shown antioxidant properties and effective ROS scavenging abilities. Unlike in many vegetables, fruits and medicinal plants, phenolic compounds are not major contributors to antioxidant capacity in microalgae due to the low phenolic contents.47 In this study, SpAE and SyAE contained abundant phycocyanin and carbohydrate, both of which accounted for more than 10% of dry mass (Table 1); so phycocyanin and polysaccharides seem to be the major antioxidant constituents of these two MAEs. In ChAE, polysaccharides might be the major antioxidant contributor considering the absence of phycobiliproteins and an over 30% carbohydrate content.
ROS play an important role in the initiation and perpetuation of inflammatory cascades, and antioxidants have been frequently found to be effective at attenuating inflammation. In the present study, MAEs exerted anti-inflammatory effects in TNF-α-, IL-1β- and H2O2-stimulated Caco-2 cells, and reduced colonic and systemic inflammation in colitis mice. Ávila-Román et al. (2014 and 2016) reported the anti-inflammatory effects of microalgal biomass of Chlamydomonas debaryana in 2,4,6-trinitrobenzenesulfonic acid-induced acute and recurrent colitis in mice.13,14 Romay et al. (1998a and 1998b) revealed a significant contribution of the antioxidative property of phycocyanin to its anti-inflammatory activity in several cellular and animal models of inflammation.48,49 According to Guzmán et al. (2003) and Yang et al. (2009), microalgal polysaccharides from S. platensis, C. stigmatophora and Phaeodactylum tricornutum could exert anti-inflammatory effects in mouse alkali burn-induced corneal neovascularization and rat carrageenan-induced paw edema.50,51 The gastrointestinal tract is the first point of contact with microalgal bioactive compounds. Consumption of microalgae thus seems to be beneficial for IBD patients to control gut inflammation.
The expression of Hsp is a natural physiological mechanism of cellular protection, and can be stimulated by several dietary nutrients including whey hydrolysate, probiotic-derived peptides, glutamine, psyllium and chicory fiber, sulfur compounds (e.g. sulforaphane, glutathione, and allicins) and phenolic compounds (e.g. resveratrol, quercetin and those from red wine and propolis) in animal and human studies.52 There are also some food substances boosting tight junction protein expression, such as glutamine, tryptophan, peptides derived from milk proteins, and phenolic compounds (e.g. quercetin, myricetin, and kaempferol).53 In the present study, MAEs induced Hsp response and tight junction protein expression in Caco-2 cells and DSS-induced colitis mice (Fig. 1c, d, and 5a–c), which might be associated with microalga-derived proteins, polysaccharides or phenolic compounds, and this provides an intestinal protective mechanism alternative to antioxidation and anti-inflammation.
Conclusions
Overall, our in vitro and in vivo results demonstrate that ChAE, SpAE and SyAE are effective at maintaining gut health by protecting the intestinal barrier function and suppressing colonic and systemic inflammatory responses. Microalgae can thus serve as functional foods to improve disease conditions in IBD patients. Further studies are needed to elucidate the functional ingredients in MAEs and their mechanisms of action.
Abbreviations
APC | Allophycocyanin |
C. pyrenoidosa
|
Chlorella pyrenoidosa
|
COX-2 | Cyclooxygenase 2 |
ChAE | Aqueous extracts of Chlorella pyrenoidosa |
DAI | Disease activity index |
DSS | Dextran sodium sulphate |
Hsp | Heat shock protein |
IBD | Inflammatory bowel diseases |
IL | Interleukin |
iNOS | Inducible nitric oxide synthase |
LDH | Lactic dehydrogenase |
MAEs | Microalgae aqueous extracts |
MPO | Myeloperoxidase |
PC | Phycocyanin |
PE | Phycoerythrin |
ROS | Reactive oxygen species |
S. platensis
|
Spirulina platensis
|
SpAE | Aqueous extracts of Spirulina platensis |
SyAE | Aqueous extracts of Synechococcus 7002 |
Synechococcus 7002 |
Synechococcus sp. PCC 7002 |
TNF | Tumor necrosis factor |
Conflicts of interest
There are no conflicts to declare.
Acknowledgements
This work was supported by the National Natural Science Foundation of China (No. 31601406).
References
- G. G. Kaplan, The global burden of IBD: from 2015 to 2025, Nat. Rev. Gastroenterol. Hepatol., 2015, 12, 720–727 CrossRef PubMed
.
- W. J. Sandborn, The present and future of inflammatory bowel disease treatment, Gastroenterol. Hepatol., 2016, 12, 438–441 Search PubMed
.
- M. Al Mijan and B. O. Lim, Diets, functional foods, and nutraceuticals as alternative therapies for inflammatory bowel disease: present status and future trends, World J. Gastroenterol., 2018, 24, 2673–2685 CrossRef CAS PubMed
.
- M. G. de Morais, B. d. S. Vaz, E. G. de Morais and J. A. V. Costa, Biologically active metabolites synthesized by microalgae, Biomed. Res. Int., 2015, 2015, 835761 Search PubMed
.
- C. Romay, R. Gonzalez, N. Ledon, D. Remirez and V. Rimbau, C-phycocyanin: a biliprotein with antioxidant, anti-inflammatory and neuroprotective effects, Curr. Protein Pept. Sci., 2003, 4, 207–216 CrossRef CAS PubMed
.
- R. Sathasivam, R. Radhakrishnan, A. Hashem and E. F. A. Allah, Microalgae metabolites: a rich source for food and medicine, Saudi J. Biol. Sci., 2017, 26, 709–722 CrossRef PubMed
.
- G. Feng, S. Dong, M. Huang, M. Zeng, Z. Liu, Y. Zhao and H. Wu, Biogenic polyphosphate nanoparticles from a marine cyanobacterium Synechococcus sp. PCC 7002: production, characterization, and anti-inflammatory properties in vitro, Mar. Drugs, 2018, 16, 322 CrossRef PubMed
.
- H. C. Bernstein, R. S. McClure, E. A. Hill, L. M. Markillie, W. B. Chrisler, M. F. Romine, J. E. McDermott, M. C. Posewitz, D. A. Bryant, A. E. Konopka, J. K. Fredrickson and A. S. Beliaev, Unlocking the constraints of cyanobacterial productivity: acclimations enabling ultrafast growth, mBio, 2016, 7, 1–10 CrossRef PubMed
.
- F. A. V. Lima, I. P. Joventino, F. P. Joventino, A. C. de Almeida, K. R. T. Neves, M. R. do Carmo, L. K. A. M. Leal, G. M. de Andrade and G. S. D. Viana, Neuroprotective activities of Spirulina platensis in the 6-OHDA model of Parkinson's disease are related to its anti-inflammatory effects, Neurochem. Res., 2017, 42, 3390–3400 CrossRef CAS
.
- F. Nasirian, M. Dadkhah, N. Moradi-kor and Z. Obeidavi, Effects of Spirulina platensis microalgae on antioxidant and anti-inflammatory factors in diabetic rats, Diabetes, Metab. Syndr. Obes.: Targets Ther., 2018, 11, 375–380 CrossRef CAS PubMed
.
- R. Gonzalez, S. Rodriguez, C. Romay, O. Ancheta, A. Gonzalez, J. Armesto, D. Remirez and N. Merino, Anti-inflammatory activity of phycocyanin extract in acetic acid-induced colitis in rats, Pharmacol. Res., 1999, 39, 55–59 CrossRef CAS PubMed
.
- R. E. Merchant and C. A. Andre, A review of recent clinical trials of the nutritional supplement Chlorella pyrenoidosa in the treatment of fibromyalgia, hypertension, and ulcerative colitis, Altern. Ther. Health Med., 2001, 7, 79–90 CAS
.
- J. Ávila-Román, E. Talero, A. Alcaide, C. de los Reyes, E. Zubia, S. Garcia-Maurino and V. Motilva, Preventive effect of the microalga Chlamydomonas debaryana, on the acute phase of experimental colitis in rats, Br. J. Nutr., 2014, 112, 1055–1064 CrossRef
.
- J. Ávila-Román, E. Talero, A. Rodriguez-Luna, S. Garcia-Maurino and V. Motilva, Anti-inflammatory effects of an oxylipin-containing lyophilised biomass from a microalga in a murine recurrent colitis model, Br. J. Nutr., 2016, 116, 2044–2052 CrossRef
.
- S. Stevens and C. Van Baalen, Characteristics of nitrate reduction in a mutant of the blue-green alga Agmenellum quadruplicatum, Plant Physiol., 1973, 51, 350–356 CrossRef CAS
.
- J. Lee, A. Park, M. Kim, H. Lim, Y. Rha and H. Kang, Spirulina extract enhanced a protective effect in type 1 diabetes by anti-apoptosis and anti-ROS production, Nutrients, 2017, 9, 1363 CrossRef
.
-
S. S. Nielsen, Total carbohydrate by phenol-sulfuric acid method, in Food Analysis Laboratory Manual, Springer International Publishing, Cham, 2017, pp. 137–141 Search PubMed
.
- L. Chen, C. Cheng and J. Liang, Effect of esterification condensation on the Folin–Ciocalteu method for the quantitative measurement of total phenols, Food Chem., 2015, 170, 10–15 CrossRef CAS PubMed
.
- S. Baer, M. Heining, P. Schwerna, R. Buchholz and H. Huebner, Optimization of spectral light quality for growth and product formation in different microalgae using a continuous photobioreactor, Algal Res., 2016, 14, 109–115 CrossRef
.
- S. N. S. Murthy, H. S. Cooper, H. Shim, R. S. Shah, S. A. Ibrahim and D. J. Sedergran, Treatment of dextran sulfate sodium-induced murine colitis by intracolonic cyclosporin, Dig. Dis. Sci., 1993, 38, 1722–1734 CrossRef CAS
.
- I. Hirata, S. Yasumoto, K. Toshina, T. Inoue, T. Nishikawa, N. Murano, M. Murano, F. Wang and K. Katsu, Evaluation of the effect of pyrrolidine dithiocarbamate in suppressing inflammation in mice with dextran sodium sulfate-induced colitis, World J. Gastroenterol., 2007, 13, 1666–1671 CrossRef CAS
.
- A. Rodriguezpalacios, T. Kodani, L. Kaydo, D. Pietropaoli, D. Corridoni, S. J. Howell, J. A. Katz, W. Q. Xin, T. T. Pizarro and F. Cominelli, Stereomicroscopic 3D-pattern profiling of murine and human intestinal inflammation reveals unique structural phenotypes, Nat. Commun., 2015, 6, 7577–7577 CrossRef PubMed
.
- M. F. G. Assuncao, R. Amaral, C. B. Martins, J. D. Ferreira, S. Ressurreicao, S. D. Santos, J. M. T. B. Varejao and L. M. A. Santos, Screening microalgae as potential sources of antioxidants, J. Appl. Phycol., 2017, 29, 865–877 CrossRef CAS
.
- J. Van De Walle, A. Hendrickx, B. Romier, Y. Larondelle and Y. J. Schneider, Inflammatory parameters in Caco-2 cells: effect of stimuli nature, concentration, combination and cell differentiation, Toxicol. in Vitro, 2010, 24, 1441–1449 CrossRef CAS
.
- A. B. Nair and S. Jacob, A simple practice guide for dose conversion between animals and human, J. Basic Clin. Pharm., 2016, 7, 27–31 CrossRef
.
- Y. Panahi, B. Darvishi, N. Jowzi, F. Beiraghdar and A. Sahebkar,
Chlorella vulgaris: a multifunctional dietary supplement with diverse medicinal properties, Curr. Pharm. Des., 2016, 22, 164–173 CrossRef PubMed
.
- R. J. Marles, M. L. Barrett, J. Barnes, M. L. Chavez, P. Gardiner, R. Ko, G. B. Mahady, T. L. Dog, N. D. Sarma, G. I. Giancaspro, M. Sharaf and J. Griffiths, United States pharmacopeia safety evaluation of Spirulina, Crit. Rev. Food Sci. Nutr., 2011, 51, 593–604 CrossRef CAS
.
- N. Jakubowska and E. Szeląg-Wasielewska, Toxic picoplanktonic cyanobacteria–review, Mar. Drugs, 2015, 13, 1497–1518 CrossRef CAS
.
- L. Jansky, P. Reymanova and J. Kopecky, Dynamics of cytokine production in human peripheral blood mononuclear cells stimulated by LPS or infected by Borrelia, Physiol. Res., 2003, 52, 593–598 Search PubMed
.
- K. Meixenberger, F. Pache, J. Eitel, B. Schmeck, S. Hippenstiel, H. Slevogt, P. N'Guessan, M. Witzenrath, M. G. Netea, T. Chakraborty, N. Suttorp and B. Opitz, Listeria monocytogenes-Infected human peripheral blood mononuclear cells produce IL-1 beta, depending on listeriolysin O and NLRP3, J. Immunol., 2010, 184, 922–930 CrossRef CAS
.
- S. U. Seo, N. Kamada, R. Munoz-Planillo, Y. G. Kim, D. Kim, Y. Koizumi, M. Hasegawa, S. D. Himpsl, H. P. Browne, T. D. Lawley, H. L. T. Mobley, N. Inohara and G. Nunez, Distinct commensals induce interleukin-1 beta via NLRP3 inflammasome in inflammatory monocytes to promote intestinal inflammation in response to injury, Immunity, 2015, 42, 744–755 CrossRef CAS
.
- J. J. Malago, J. F. J. G. Koninkx and J. E. Van Dijk, The heat shock response and cytoprotection of the intestinal epithelium, Cell Stress Chaperones, 2002, 7, 191–199 CrossRef CAS
.
- E. O. Petrof, M. J. Ciancio and E. B. Chang, Role and regulation of intestinal epithelial heat shock proteins in health and disease, Chin. J. Dig. Dis., 2004, 5, 45–50 CrossRef CAS
.
- N. Ichikawa-Tomikawa, K. Sugimoto, S. Satohisa, K. Nishiura and H. Chiba, Possible involvement of tight junctions, extracellular matrix and nuclear receptors in epithelial differentiation, BioMed. Res. Int., 2015, 2011, 137–143 Search PubMed
.
- Y. Aratani, Myeloperoxidase: its role for host defense, inflammation, and neutrophil function, Arch. Biochem. Biophys., 2018, 640, 47–52 CrossRef CAS
.
- I. Soufli, R. Toumi, H. Rafa and C. Touilboukoffa, Overview of cytokines and nitric oxide involvement in immuno-pathogenesis of inflammatory bowel diseases, World J. Gastrointest. Pharmacol. Ther., 2016, 7, 353–360 CrossRef
.
- B. Palenik and R. Haselkorn, Multiple evolutionary origins of prochlorophytes, the chlorophyll b-containing prokaryotes, Nature, 1992, 355, 265–267 CrossRef CAS
.
-
Govindjee, J. T. Beatty, H. Gest and J. F. Allen, Discoveries in Photosynthesis, Springer, Netherlands, 2005 Search PubMed
.
- L. Mendez, B. Sialve, E. Tomas-Pejo, M. Ballesteros, J. P. Steyer and C. Gonzalez-Fernandez, Comparison of Chlorella vulgaris and cyanobacterial biomass: cultivation in urban wastewater and methane production, Bioprocess Biosyst. Eng., 2016, 39, 703–712 CrossRef CAS
.
- A. Bhattacharyya, R. Chattopadhyay, S. Mitra and S. E. Crowe, Oxidative stress: an essential factor in the pathogenesis of gastrointestinal mucosal diseases, Physiol. Rev., 2014, 94, 329–354 CrossRef CAS
.
- J. Matos, C. Cardoso, N. M. Bandarra and C. Afonso, Microalgae as healthy ingredients for functional food: a review, Food Funct., 2017, 8, 2672–2685 RSC
.
- W. Chu, Y. Lim, A. K. Radhakrishnan and P. Lim, Protective effect of aqueous extract from Spirulina platensis against cell death induced by free radicals, BMC Complementary Altern. Med., 2010, 10, 53 CrossRef
.
- M. Khan, S. Varadharaj, J. C. Shobha, M. U. Naidu, N. L. Parinandi, V. K. Kutala and P. Kuppusamy, C-phycocyanin ameliorates doxorubicin-induced oxidative stress and apoptosis in adult rat cardiomyocytes, J. Cardiovasc. Pharmacol., 2006, 47, 9–20 CrossRef CAS
.
- J. Marin-Prida, G. Penton-Rol, F. P. Rodrigues, L. C. Alberici, K. Stringhetta, A. M. Leopoldino, Z. Naal, A. C. M. Polizello, A. Llopiz-Arzuaga, M. N. Rosa, J. L. Liberato, W. F. dos Santos, S. A. Uyemura, E. Penton-Arias, C. Curti and G. L. Pardo-Andreu, C-Phycocyanin protects SH-SY5Y cells from oxidative injury, rat retina from transient ischemia and rat brain mitochondria from Ca2+/phosphate-induced impairment, Brain Res. Bull., 2012, 89, 159–167 CrossRef CAS
.
- P. Pleonsil, S. Soogarun and Y. Suwanwong, Anti-oxidant activity of holo- and apo-C-phycocyanin and their protective effects on human erythrocytes, Int. J. Biol. Macromol., 2013, 60, 393–398 CrossRef CAS
.
- V. Rimbau, A. Camins, D. Pubill, F. X. Sureda, C. Romay, R. Gonzalez, A. Jimenez, E. Escubedo, J. Camarasa and M. Pallas, C-phycocyanin protects cerebellar granule cells from low potassium/serum deprivation-induced apoptosis, Naunyn-Schmiedeberg's Arch. Pharmacol., 2001, 364, 96–104 CrossRef CAS
.
- H. Li, K. Cheng, C. Wong, K. Fan, F. Chen and Y. Jiang, Evaluation of antioxidant capacity and total phenolic content of different fractions of selected microalgae, Food Chem., 2007, 102, 771–776 CrossRef CAS
.
- C. Romay, J. Armesto, D. Remirez, R. Gonzalez, N. Ledon and I. Garcia, Antioxidant and anti-inflammatory properties of C-phycocyanin from blue-green algae, Inflammation Res., 1998, 47, 36–41 CrossRef CAS
.
- C. Romay, N. Ledon and R. Gonzalez, Further studies on anti-inflammatory activity of phycocyanin in some animal models of inflammation, Inflammation Res., 1998, 47, 334–338 CrossRef CAS
.
- L. Yang, Y. Wang, Q. Zhou, P. Chen, Y. Wang, Y. Wang, T. Liu and L. Xie, Inhibitory effects of polysaccharide extract from Spirulina platensis on corneal neovascularization, Mol. Vision, 2009, 15, 1951–1961 CAS
.
- S. Guzmán, A. Gato, M. Lamela, M. Freire-Garabal and J. M. Calleja, Anti-inflammatory and immunomodulatory activities of polysaccharide from Chlorella stigmatophora and Phaeodactylum tricomutum, Phytother. Res., 2003, 17, 665–670 CrossRef
.
- C. S. Moura, P. C. B. Lollo, P. N. Morato and J. Amaya-Farfan, Dietary nutrients and bioactive substances modulate heat shock protein (HSP) expression: a review, Nutrients, 2018, 10, 683 CrossRef PubMed
.
- S. De Santis, E. Cavalcanti, M. Mastronardi, E. Jirillo and M. Chieppa, Nutritional keys for intestinal barrier modulation, Front. Immunol., 2015, 6, 612 Search PubMed
.
Footnote |
† Electronic supplementary information (ESI) available. See DOI: 10.1039/c9fo01028a |
|
This journal is © The Royal Society of Chemistry 2020 |
Click here to see how this site uses Cookies. View our privacy policy here.